Abstract
Objective
The aim of this study was to examine the properties of fiber-reinforced composite and stainless steel twisted retainers for orthodontic retention.
Methods
Three different span lengths (5.0, 8.0, and 14.0 mm) of fiber-reinforced composite were investigated. The three fiber-reinforced composite retainer groups were subdivided according to the storage condition (dry and wet), resulting in a total of six groups. Each stainless steel and fiber-reinforced composite group was comprised of six specimens. The three-point bending flexural test was conducted using a universal testing machine. ANOVA was used to assess differences in the maximum load and maximum stress according to the span length, material, and storage condition. Post-hoc comparisons were performed if necessary.
Results
The maximum stress and maximum load were significantly (p < 0.001) associated with the span length, material, and storage condition. The significant interaction between the material and span length (p < 0.001) indicated the differential effects of the material for each span length on the maximum stress and maximum load, with the difference between materials being the highest for the maximum span length.
Retention is considered an important phase of orthodontic treatment as there is a tendency for teeth to relapse or return to their initial positions without any type of retention.1
Orthodontic bonded retainers are frequently used to prevent the relapse of crowding in the mandibular anterior region.2345 A previous study showed that patients who wore a fixed retention appliance exhibited consistently better alignment at 5 and 10 years after treatment compared with those without fixed retention, even though the former exhibited higher Peer Assessment Rating scores before treatment compared with the latter.2
The introduction of fiber-reinforced composites (FRCs) has presented a potential alternative to bonded metal wires for fixed orthodontic retention. The good engineering properties of FRCs are primarily the result of their high stiffness-weight (specific modulus) and strength-weight (specific strength) ratios compared with those of other structural materials.67 The specific modulus of elasticity and enhanced load-bearing capabilities of the polymers offer exciting possibilities for several applications in orthodontic practice, particularly with regard to the ease of manipulation and better esthetics compared with those of stainless steel and metal alloys.8910
FRC retainers (everStick ORTHO; StickTech, Turku, Finland) are composed of glass fibers, thermoplastic polymer, and light-cured resin matrix for reinforcement of the dental polymer.1011 The final product contains 1,000 individual glass fibers; this yields an effective diameter of 0.75 mm and a cross-sectional area of 0.5 mm2.
From the biomechanical perspective, however, several factors influence the properties of FRCs. Two primary factors include the fiber properties and fiber-matrix interaction properties. While fiber properties include the orientation, quantity, and type of fibers, fiber-matrix interaction properties include impregnation of fibers with a polymer matrix, adhesion of fibers to the polymer matrix, and harmony between the characteristics of the fibers and polymer matrix.12 Indeed, the relevance of fiber behavior is reflected in their ability to prevent, decelerate, or even enhance cracking.91213
For the consideration of FRC retainers as a possible alternative to conventional multistrand stainless steel retainers for orthodontic treatment, a comparison of load values between the two materials would be of interesting value.
Accordingly, the aim of the present study was to evaluate the force levels of FRC retainers according to three different span lengths (5.0, 8.0, and 14.0 mm) and two different storage conditions (dry and wet, in order to evaluate how mechanical properties change in a wet environment like the oral cavity) using a three-point bending flexural test and compare the findings with those for multistrand stainless steel wires (diameter, 0.55 mm).14
Two different orthodontic retainers were evaluated in this article: FRCs retainers (everStick ORTHO) and a multistrand stainless steel wire (Penta One; Masel, Bristol, PA, USA) (Table 1). Each material was tested for three different span lengths of 5.0, 8.0, and 14.0 mm. The three FRC groups were subdivided according to the storage condition (dry and wet), resulting in a total of six FRC groups. Each FRC and stainless steel group comprised of six specimens. All FRC specimens were handled according to the manufacturer's guidelines and were cut to a length of 20 mm using scissors before polymerization.
All FRC samples (length, 20 mm) were polymerized, according to the manufacturer's guidelines, for 10 seconds per tooth and for 40 seconds after the first polymerization using a halogen curing unit (Optilux; SDS Kerr, Danbury, CT, USA) with a light intensity 930 mW/cm2 and wavelength of 400–505 nm. The light intensity was measured by the internal radiometer device of the curing unit and monitored throughout the study. After curing, the specimens were stored under dry conditions. To test the water absorption ability, nine FRC specimens were weighed with a precision balance (Delta Range AT 261; Mettler Toledo, Columbus, OH, USA) before (T0) and 6 (T1), 12 (T2), 24 (T3), 48 (T4), and 72 (T5) hours after immersion in distilled water at 37℃. The water absorption capacity of the FRC specimens was reached within 2 days (Figure 1).
All stainless steel and FRC specimens were subsequently tested with a three-point bending flexural test,15 which was conducted using a universal testing machine (Lloyd LRX; AMETEK, Inc., Berwyn, PA, USA), for the span lengths of 5, 8, and 14 mm (crosshead speed, 1.0 mm/min). The machine applied a load to the center of the specimens, and load-deflection curves were recorded for 1.0 mm of deflection using Nexygen 4.0 MT software (Lloyd LRX). The interpoint distance for the 5-, 8-, and 14-mm span lengths was 7, 4, and 2.5 mm, respectively. The center point of the machine was moved by a computer-controlled stepper motor, and, at the same time, the force and position of the center point of the machine relative to the passive position were recorded by electronic sensors.
Descriptive statistics were computed as medians and 25th–75th percentiles for continuous variables and as numbers and percentage for categorical variables. ANOVA was used to assess differences in the maximum load (N) and maximum stress (MPa) according to the span length, material, and storage condition. Post-hoc comparisons were performed if necessary.
A two-sided p-value of < 0.05 and < 0.001 was considered statistically significant in the main analysis and post-hoc comparisons (Bonferroni correction), respectively. All statistical analyses were performed using STATA 10 (Stata Corp, College Station, TX, USA).
From the load trials conducted for the different FRC (6 groups) and stainless steel (3 groups) groups, it was possible to find the average curves for their mechanical behavior (Figure 2). None of the specimens completely fractured in any test, and only microfractures were clinically observed as white spots.
The descriptive statistics for the nine groups and comparisons of flexural values (maximum stress [MPa] and maximum load [N]) are shown in Table 2.
The maximum stress and maximum load significantly differed among the span lengths for each material and storage condition (p < 0.001 for all).
Three-factor ANOVA for the maximum load and maximum stress revealed significant differences in values according to the span length, material, and storage condition (p < 0.001). Post-hoc tests demonstrated significantly better mechanical properties for the FRC groups than for the stainless steel groups (p < 0.001). Moreover, the differences between the two materials were the highest for the longest span length (p < 0.001). With regard to the span length of 5 mm, there was no significant difference between the FRC specimens stored under wet conditions and stainless steel specimens.
Under equal conditions (span length, deflection, and storage condition), the stainless steel wires reached significantly inferior loads compared with the FRC specimens stored under dry conditions (p < 0.001). However, the stainless steel wires exhibited greater elasticity compared with the FRC specimens (Figure 2).
This present study determined the effects of three different span lengths and different storage conditions (dry and wet) on the load-deflection behavior of FRC retainers and compared the findings with those for conventional orthodontic multistrand archwires.
Concerning the 5-mm span length, there were no significant differences between stainless steel and FRC retainers stored under wet conditions. From the load trials carried out on various samples of FRCs and multistrand stainless steel wires, it was possible to derive the average curves of their mechanical behavior. The failure behavior of FRCs could be due to their anisotropic character, as demonstrated in another study.16 The ability of laminated composites to absorb energy during a local impact is relatively poor.17 Therefore, reinforcement with more fibers in a given composite volume may change the load-bearing capacity of the entire structure.1819
Overall, the FRC groups (dry and wet storage conditions) exhibited greater variations in load and elasticity values, whereas the stainless steel groups exhibited greater consistency. The wet fibers reached inferior loads compared to the dry fibers; moreover, the former exhibited greater elasticity compared with the latter. Thus, the characteristics of the FRC specimens stored under wet conditions were more similar to those of stainless steel. With regard to the FRC retainers stored under dry conditions, those with a span length of 5 mm supported greater loads compared with their stainless steel counterparts. Moreover, those with an increased span length tolerated smaller loads and exhibited greater elasticity compared to those with a 5-mm span length, appearing more similar to the stainless steel specimens. Bearn et al.20 claimed that wires with a larger diameter and greater surface area require greater force to pull the wire out from the composite resin. However, Baysal et al.21 found that the Penta One wire exhibited the highest mean resistance to displacement from composite resin, even though it was not the wire with the largest diameter.
In the present study, a difference in the results was observed in relation to the storage conditions of the fibers (wet or dry). The different material, storage condition (dry and wet), and span length significantly influenced the maximum load (p < 0.005).
Collectively, our findings demonstrated that FRC retainers stored under wet conditions were more similar to the stainless steel retainers. Finally, the ultimate success of a bonded retainer is determined by the size and quality of teeth, the occlusal load on the retainer, and minimum thickness, considering that bulky constructions may result in plaque accumulation with reversible or irreversible damage to the enamel and dentin and occasionally irritate the tongue.222324 Large tooth crowns provide large areas of bonding, which allows the load to spread over a wide area of enamel. Therefore, to evaluate the success rates for these wires, randomized controlled in vivo studies should be performed. Nevertheless, it is possible to state that FRCs exhibit mechanical properties that may be similar to or better than those of metal alloys and exhibit several clinical advantages, including suitability for patients requiring long-term retention, increased patient satisfaction, ease of cleaning, good esthetics, and less chair time.
The following important findings were observed in our study.
1) Stainless steel wires supported smaller loads and exhibited greater elasticity compared with FRC wires.
2) FRC wires with a smaller span length supported greater loads compared with stainless steel wires.
3) FRC wires with a greater span length supported smaller loads but were more elastic; therefore, they appeared more similar to stainless steel wires.
4) FRC wires stored under wet conditions supported smaller loads and exhibited greater elasticity compared to those stored under dry conditions, thus appearing more similar to stainless steel wires.
5) FRC wires are completely transparent and can be fabricated to resemble the shade of the tooth.
6) FRC wires can be used in patients with metal allergy.
In summary, our findings indicate that the mechanical properties of FRCs are similar to or better than those of metals. FRC retainers may be an effective alternative for orthodontic retention in patients with esthetic concerns or allergy to conventional stainless steel wires and can be used for anchorage during orthodontic treatment.
Figures and Tables
Figure 1
Water absorption after immersion of fiber-reinforced composite retainers (OrthoStick) in distilled water at 37℃.
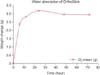
Figure 2
Load-deflection curves generated on the basis of a three-point bending flexural test for different span lengths of multistrand stainless steel (SS) and fiber-reinforced composite (FRC) retainers stored under dry and wet conditions. A, 5 mm; B, 8 mm; C, 14 mm.
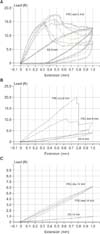
ACKNOWLEDGEMENTS
The authors would like to acknowledge Vita Salute San Raffaele University, Milano, Italy.
References
1. Littlewood SJ, Millett DT, Doubleday B, Bearn DR, Worthington HV. Retention procedures for stabilising tooth position after treatment with orthodontic braces. Cochrane Database Syst Rev. 2016; (1):CD002283.


2. Al Yami EA, Kuijpers-Jagtman AM, van't Hof MA. Stability of orthodontic treatment outcome: follow-up until 10 years postretention. Am J Orthod Dentofacial Orthop. 1999; 115:300–304.


3. Dahl EH, Zachrisson BU. Long-term experience with direct-bonded lingual retainers. J Clin Orthod. 1991; 25:619–630.
4. Lucchese A, Gherlone E, Portelli M, Bertossi D. Tooth orthodontic movement after maxillofacial surgery. Eur J Inflamm. 2012; 10:227–231.


5. Portelli M, Matarese G, Militi A, Logiudice G, Nucera R, Lucchese A. Temporomandibular joint involvement in a cohort of patients with juvenile idiopatic arthritis and evaluation of the effect induced by functional orthodontic appliance: clinical and radiographic investigation. Eur J Paediatr Dent. 2014; 15:63–66.
6. Lucchese A, Carinci F, Brunelli G, Monguzzi R. An in vitro study of resistance to corrosion in brazed and laser-welded orthodontic appliances. Eur J Inflamm. 2011; 9:67–72.
7. Ardeshna AP. Clinical evaluation of fiber-reinforced-plastic bonded orthodontic retainers. Am J Orthod Dentofacial Orthop. 2011; 139:761–767.


8. Goldberg AJ, Burstone CJ. The use of continuous fiber reinforcement in dentistry. Dent Mater. 1992; 8:197–202.


9. Freilich MA, Meiers JC, Duncan JP, Goldberg AJ. Fiber-reinforced composites in clinical dentistry. Chicago: Quintessence Publishing;1999. p. 18–46.
10. Burstone CJ, Kuhlberg AJ. Fiber-reinforced composite in orthodontics. J Clin Orthod. 2000; 34:271–279.
11. Lucchese A, Manuelli M, Bassani L, Albertini P, Matarese G, Perillo L, et al. Fiber reinforced composites orthodontic retainers. Minerva Stomatol. 2015; 64:323–333.
12. Lastumäki TM, Kallio TT, Vallittu PK. The bond strength of light-curing composite resin to finally polymerized and aged glass fiber-reinforced composite substrate. Biomaterials. 2002; 23:4533–4539.


13. Göhring TN, Gallo L, Lüthy H. Effect of water storage, thermocycling, the incorporation and site of placement of glass-fibers on the flexural strength of veneering composite. Dent Mater. 2005; 21:761–772.


14. Garoushi S, Lassila LV, Vallittu PK. The effect of span length of flexural testing on properties of short fiber reinforced composite. J Mater Sci Mater Med. 2012; 23:325–328.


15. Rucker BK, Kusy RP. Elastic flexural properties of multistranded stainless steel versus conventional nickel titanium archwires. Angle Orthod. 2002; 72:302–309.
16. Tezvergil A, Lassila LV, Vallittu PK. Strength of adhesive-bonded fiber-reinforced composites to enamel and dentin substrates. J Adhes Dent. 2003; 5:301–311.
17. Van Meerbeek B, Perdigão J, Lambrechts P, Vanherle G. The clinical performance of adhesives. J Dent. 1998; 26:1–20.


18. Roncati M, Lucchese A, Carinci F. Non-surgical treatment of peri-implantitis with the adjunctive use of an 810-nm diode laser. J Indian Soc Periodontol. 2013; 17:812–815.


19. Lucchese A, Carinci F, Saggese V, Lauritano D. Immediate loading versus traditional approach in functional implantology. Eur J Inflamm. 2012; 10:55–58.
20. Bearn DR, McCabe JF, Gordon PH, Aird JC. Bonded orthodontic retainers: the wire-composite interface. Am J Orthod Dentofacial Orthop. 1997; 111:67–74.


21. Baysal A, Uysal T, Gul N, Alan MB, Ramoglu SI. Comparison of three different orthodontic wires for bonded lingual retainer fabrication. Korean J Orthod. 2012; 42:39–46.


22. Bryan DC, Sherriff M. An in vitro comparison between a bonded retainer system and a directly bonded flexible spiral wire retainer. Eur J Orthod. 1995; 17:143–151.


23. Lucchese A, Bertacci A, Chersoni S, Portelli M. Primary enamel permeability: a SEM evaluation in vivo. Eur J Paediatr Dent. 2012; 13:231–235.
24. Prati C, Chersoni S, Lucchese A, Pashley DH, Mongiorgi R. Dentin permeability after toothbrushing with different toothpastes. Am J Dent. 1999; 12:190–193.