Abstract
Purpose
To investigate whether a correlation exists between the semi-quantitative breast-specific gamma imaging (BSGI) findings and dynamic contrast-enhanced (DCE) MRI parameters assessed by a computer-aided evaluation program.
Materials and Methods
Semi-quantitative index of the lesion to non-lesion (L/N) ratio in BSGI and DCE-MRI parameters was assessed by a computer-aided evaluation program, where 47 cases of invasive breast cancer were obtained. Correlations between the L/N ratio and DCE-MRI parameters were assessed by a computer-aided evaluation program. Tumor diameter (cm), angio-volume (cc), degree of initial peak enhancement (%), persistent enhancement proportion (%), and washout enhancement proportion (%) were analysed. The relationships between the L/N ratio and DCE-MRI parameters were evaluated by a univariate and multivariate regression analysis.
Results
The mean L/N ratio of the 47 tumors was 3.63 ± 2.19 (range: 1–13.1). The L/N ratio was higher in tumors with larger diameters (p < 0.001), increased angio-volume (p < 0.001), higher degree of initial peak enhancement (p = 0.005) and increased washout enhancement proportion (p = 0.004). In the multivariate regression analysis, angio-volume (cc) and washout enhancement proportion (%) were associated with L/N ratio (p = 0.007 and p = 0.024, respectively).
Breast cancer is one of the most common female malignancies. In the past decade, breast-specific gamma imaging (BSGI) with 99mTc-methoxyisobutylisonitrile (MIBI) has evolved for use in cancer detection and has complemented traditional imaging methods. However, the exact uptake mechanism of 99mTc-MIBI is still under investigation. It is reported that 99mTc-MIBI is distributed inside tissues in proportion to the blood flow and is especially concentrated in malignant cells. Approximately 90% of the tracer is stored within mitochondria (1). BSGI provides independent biological information specific to breast cancer (23456).
MRI is an effective diagnostic tool for differentiation between benign and malignant breast masses, preoperative evaluation in breast cancer patients, breast cancer screening and early prediction of the response to neoadjuvant chemotherapy (78). Dynamic contrast-enhanced (DCE) MRI can depict the distribution of a contrast agent within a tumor over time and can non-invasively assess the tissue vasculature (9). Computer-aided detection (CAD) programs for DCE-MRI automate kinetic assessment and provide an easier way to interpret the patterns of contrast enhancement across a series of images (1011).
Several studies have demonstrated that BSGI and DEC-MRI have similar sensitivity and specificity in breast cancer detection (1213). However, little is known about the correlation of 99mTc-MIBI uptake in BSGI with DCE-MRI parameters of breast cancer. Therefore, we aimed to investigate whether a correlation exists between the semi-quantitative BSGI findings and DCE-MRI parameters assessed by a computer-aided evaluation program for breast cancer.
The visual interpretation criteria for BSGI were suggested earlier (14). Images were categorized asnormal (score of 1), with no focal or diffuse uptake; benign (score of 2), with minimal patchy uptake; probably benign (score of 3), with minimal patchy uptake with some areas of more focal uptake; probably abnormal (score of 4), with mild focal radiotracer uptake; and abnormal (score of 5), with marked focal radiotracer uptake. These criteria are qualitative and rather subjective. The lesion to non-lesion (L/N) ratio for semi-quantitative analysisreflects the 99mTc-MIBI uptake level and is an important parameter of BSGI as a functional imaging (3).
The Institutional Review Board of our hospital approved this retrospective study (2014-12-012); the requirement for informed consent was waived. Between January 2014 and December 2014, 113 patients who had suspicious mammography or ultrasound findings were imaged with BSGI. Among them, 47 patients with lesions that had been biopsied and were confirmed to be invasive breast cancer underwent breast DCE-MRI for initial staging. One patient was later excluded due to non-physiological CAD parameter values. One patient had bilateral breast cancer. Finally, this retrospective study involved 46 patients (mean age: 51 years, range: 32–68 years) with 47 index breast cancers. None of the patients received chemotherapy or radiation therapy prior to the study.
BSGI examinations were performed after the patients were administered 20 mCi 99mTc-MIBI through an antecubital vein. The patients were seated for the procedure, and craniocaudal (CC) and mediolateral oblique (MLO) view images were acquired using a high-resolution breast-specific gamma camera (Dilon 6800 gamma camera; Dilon Technologies, Newport News, VA, USA). Images were acquired for 10 min for each view.
Semi-quantitative analysis of BSGI was performed by two board-certified radiologists in consensus, one with 10 years of experience and one with 6 years of experience in breast imaging. The readers were not blinded to the lesion site. The L/N ratio was considered as the semi-quantitative indices of BSGI.
For lesions, a region of interest (ROI) was manually drawn covering the target lesion area. The maximum 9 pixel counts of the ROI were measured on different CC and MLO images (6). The higher value was chosen for lesion maximal uptake between the CC and MLO views. For non-lesions, another three circular ROIs, approximately 1 cm in diameter, were drawn in the contralateral trisectioned breast parallel to the base of the breast (anterior/middle/posterior), and the mean values of the three ROIs were averaged into a single value (Fig. 1). The L/N ratio was then calculated by dividing the maximum pixel counts of the lesion by the average non-lesion uptake. There were two cases in which the tumor (lesion) and background breast tissue (non-lesion) were not distinguishable. The L/N ratio for these cases was regarded as 1.
All MR examinations were performed by using two 3.0-T MR imagers with a dedicated 8-channel breast coil (n = 20, Achieva, Philips, Best, Netherlands) or with a dedicated 16-channel breast coil (n = 26, Ingenia, Philips). The DCE-MR images were obtained by using the following image parameters: T1-weighted fast spoiled gradient-echo sequence (5.4/2.5; matrix, 440 × 426; flip angle, 12°; field of view, 320 × 320 mm; section thickness, 1 mm; no gap) with one pre-contrast and four post-contrast dynamic series obtained at 100, 200, 300, and 400 seconds after injection of a contrast agent. A 0.2 mmol/kg dose of gadobutrol (Gadovist; Bayer-Healthcare, Berlin, Germany) was injected into an antecubital vein using an automated injector at a rate of 2 mL/sec, followed by a 20-mL saline flush after the contrast agent was injected.
The CAD analysis was performed by one radiologist who specialized in breast imaging and had 6 years of experience. For measurement of dynamic MR image parameters, pre-contrast and four post-contrast T1-weighted image series were transferred to a computer-aided evaluation system (CADstream, version 4.1.3; Confirma, Kirkland, WA, USA). The system automatically segmented the tumors into three dimensions and calculated the tumor diameter (maximal size of an enhancing lesion); angio-volume (total enhancing lesion volume); peak enhancement value (highest pixel signal intensity at the first post-contrast series); and proportions of persistent, plateau, and washout enhancement components within a tumor.
Correlations between the L/N ratio and DCE-MRI parameters assessed by a computer-aided evaluation program, including tumor diameter (cm), angio-volume (cc), degree of initial peak enhancement (%), persistent enhancement proportion (%), and washout enhancement proportion (%), were then analysed.
We used linear regression analysis to evaluate the association between the L/N ratio and DCE-MRI parameters. Variables with p < 0.05 in the univariate analysis were applied to a multivariate analysis to determine which variables were independently associated with L/N ratio.
The data were analysed using statistical software (SAS, version 9.2; SAS Institute, Cary, NC, USA). Statistical significance was defined as p < 0.05.
The mean L/N ratio of the 47 tumors was 3.63 ± 2.19 (range: 1–13.1). The DCE-MRI parameters assessed by a computer-aided evaluation program of the 47 tumors were as follows: the mean diameter of the tumor, 2.65 ± 1.83 cm (range 0.5–8.1, median 2.3); angio-volume, 7.7 ± 12.60 cc (range 0.04–57.9, median 2.2); degree of initial peak enhancement, 313.3 ± 271.85% (range 99–1763, median 247); wash out enhancement proportion, 18.1 ± 20.71% (range 0–69, median 7.5), and rapid enhancement proportion, 68.9 ± 32.39% (range 0–100, median 84).
In the univariate analysis, the L/N ratio was significantly higher in tumors with larger diameter (p < 0.001), increased angio-volume (p < 0.001), higher degree of initial peak enhancement (p = 0.005), increased washout enhancement proportion (p = 0.004) and higher rapid enhancement proportion (p = 0.047) (Table 1).
In the multivariate regression analysis, angio-volume (cc) and washout enhancement proportion (%) were significantly associated with L/N ratio (Table 2). The estimated L/N ratio increases 0.08 for each increase in angio volume (cc) and 0.03 for each increase in washout enhancement proportion (%), respectively.
In this study, we investigated whether a correlation exists between the semi-quantitative BSGI findings and DCE-MRI parameters assessed by a computer-aided evaluation program for 47 breast cancers. We observed that the L/N ratio was higher in tumors with larger diameter (p < 0.001) and increased angio-volume (p < 0.001). These results are similar to those of previously published studies: 99mTc-MIBI uptake has been found to be positively related to tumor size. In a previous study of 102 breast cancers, the value of the semi-quantitative index expressed as the L/N ratio was independently related to tumor size (p = 0.002) and infiltration degree (p = 0.016). The study showed that the sensitivity of BSGI in diagnosing breast cancer was related to tumor size: 100% for tumor size more than 2.0 cm and 89.1% for tumors less than or equal to 2.0 cm (15). Tadwalkar et al. also reported that BSGI detected all invasive breast cancers that measured ≥ 7 mm regardless of grade (16). We had two pathology-confirmed breast cancer cases, considering no uptake in the MIBI scan; in other words, the L/N ratio was 1. These two breast cancer tumors were 0.6 cm and 0.8 cm in diameter. The sensitivity for subcentimeter cancer was 71.4% (5/7) in our study. Further studies are needed for a more comprehensive analysis of BSGI results with respect to tumor characteristics.
There are several studies regarding the existence of a correlation between the kinetic parameters on DCE-MRI and histopathologic prognostic factors of breast cancer. Baltzer et al. (17) reported that higher lesion volume and higher and earlier initial enhancement were independent covariates that are predictive of higher tumor aggressiveness. In another study, numeric ratios obtained on gadolinium-enhanced dynamic MRI of the breast may be useful in predicting histologic-type breast carcinomas, and there are three histologic prognostic factors used for invasive ductal carcinoma: modified Scarff-Bloom-Richardson grade, microvessel density, and fibrotic focus (18). Bekiş et al. (19) reported that there was no statistically significant correlation between the degree of angiogenesis and early or delayed 99mTc-MIBI uptake and the washout index of 99mTc-MIBI in 24 invasive MIBI-positive breast lesions although there was a high correlation between angiogenesis and the washout index of 99mTc-MIBI in seven noninvasive breast lesions. We evaluated multiple DCE-MRI parameters that were assessed by CAD, such as tumor diameter, angio-volume, peak enhancement value, and proportions of persistent, plateau and washout enhancement components. Among these CAD parameters, increased uptake of 99mTc-MIBI in BSGI correlated with angio-volume (cc) and washout enhancement proportion (%) in the multivariate regression analysis. To the best of our knowledge, the present study is the first to correlate 99mTc-MIBI uptake in BSGI and DCE-MRI parameters for breast cancer. Further studies such as the relationship between 99mTc-MIBI uptake or ADC values and immunohistochemical prognostic factors are needed to investigate whether BSGI can be used as a non-invasive tool to study the aggressiveness of breast cancer.
The main limitation of the present study is its retrospective design, which unavoidably introduces selection bias and limits the general application of the results. The small sample size is also a limitation, as it is not sufficient for accurate statistical analysis. In addition, this study lacked histopathologic correlation for breast cancer such as subtype, hormone receptor status or histologic grade which could have affected the result of 99mTc-MIBI uptake in BSGI or DCE-MRI parameters. Further research for correlation between 99mTc-MIBI uptake and histopathology of breast cancer could be valuable. Limitations of this study include the fact that MRI was acquired on two different scanner-coil systems. The differences in spatial resolution and boundary detection for delineating ROIs of lesion could have affected the results of CAD parameters.
In conclusion, there was a correlation between the semi-quantitative L/N ratio in BSGI with DCE-MRI parameters assessed by a computer-aided evaluation program for breast cancer. We observed that increased uptake of 99mTc-MIBI in BSGI was associated with increased angio-volume (cc) and washout enhancement proportion (%) in DCE-MRI.
Figures and Tables
Fig. 1
Lesion to non-lesion ratio of breast cancer in the left upper outer breast. For lesions, the maximum ROI covering the lesion is drawn at the craniocaudal view and the mediolateral oblique view. For non-lesions, another three circular ROIs, approximately 1 cm in diameter, are drawn in the contralateral trisectioned breast, parallel to the base of the breast (anterior/middle/posterior).
ROI = region of interest
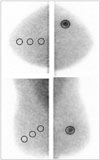
Fig. 2
Scatter plot of L/N ratio in breast-specific gamma imaging and angio-volume (coefficient of determination, R2 = 0.58).
L/N = lesion to non-lesion
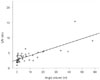
Fig. 3
Scatter plot of L/N ratio in breast-specific gamma imaging and washout enhancement proportion (coefficient of determination, R2 = 0.16).
L/N = lesion to non-lesion
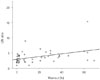
Fig. 4
Invasive ductal carcinoma in a 47 year-old woman.
A. Rt. cranio-caudal view of breast-specific gamma imaging. Lesion to non-lesion ratio is 3.97.
B. Angiomap and volume rendering image of the tumor obtained by computer-aided evaluation program in DCE-MRI (diameters: 2.9 × 2.0 × 2.2 cm, angio volume: 5.0 cc and washout enhancement proportion: 39%).
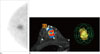
Fig. 5
Invasive ductal carcinoma in a 54 year-old woman.
A. Rt. cranio-caudal view of breast-specific gamma imaging. Lesion to non-lesion ratio is 8.99.
B. Angiomap and volume rendering image of the tumor obtained by computer-aided evaluation program in DCE-MRI (diameters: 6.9 × 5.1 × 8.1 cm, angio volume: 57.9 cc, washout enhancement proportion: 76%).
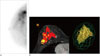
Table 1
Result of Univariate Regression Analysis between CAD Parameter and Lesion to Non-Lesion Ratio on Breast-Specific Gamma Imaging

Table 2
Results of Multivariate Regression Analysis between Computer-Aided Detection Parameter and Lesion to Non-Lesion Ratio on Breast-Specific Gamma Imaging
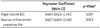
Regression Coefficient (95% CI) | p-Value* | |
---|---|---|
Angio volume (cc) | 0.084 (0.025–0.143) | 0.007 |
Washout enhancement proportion (%) | 0.027 (0.004–0.049) | 0.024 |
References
1. Brem RF, Rechtman LR. Nuclear medicine imaging of the breast: a novel, physiologic approach to breast cancer detection and diagnosis. Radiol Clin North Am. 2010; 48:1055–1074.


2. Yoon HJ, Kim Y, Chang KT, Kim BS. Prognostic value of semi-quantitative tumor uptake on Tc-99m sestamibi breast-specific gamma imaging in invasive ductal breast cancer. Ann Nucl Med. 2015; 29:553–560.


3. Yu X, Hu G, Zhang Z, Qiu F, Shao X, Wang X, et al. Retrospective and comparative analysis of (99m)Tc-Sestamibi breast specific gamma imaging versus mammography, ultrasound, and magnetic resonance imaging for the detection of breast cancer in Chinese women. BMC Cancer. 2016; 16:450.


4. Specht JM, Mankoff DA. Advances in molecular imaging for breast cancer detection and characterization. Breast Cancer Res. 2012; 14:206.


5. Sun Y, Wei W, Yang HW, Liu JL. Clinical usefulness of breast-specific gamma imaging as an adjunct modality to mammography for diagnosis of breast cancer: a systemic review and meta-analysis. Eur J Nucl Med Mol Imaging. 2013; 40:450–463.


6. Tan H, Jiang L, Gu Y, Xiu Y, Han L, Wu P, et al. Visual and semi-quantitative analyses of dual-phase breast-specific gamma imaging with Tc-99m-sestamibi in detecting primary breast cancer. Ann Nucl Med. 2014; 28:17–24.


7. Kuhl CK, Mielcareck P, Klaschik S, Leutner C, Wardelmann E, Gieseke J, et al. Dynamic breast MR imaging: are signal intensity time course data useful for differential diagnosis of enhancing lesions? Radiology. 1999; 211:101–110.


8. Orel SG, Schnall MD. MR imaging of the breast for the detection, diagnosis, and staging of breast cancer. Radiology. 2001; 220:13–30.


9. Jena A, Taneja S, Singh A, Negi P, Mehta SB, Sarin R. Role of pharmacokinetic parameters derived with high temporal resolution DCE MRI using simultaneous PET/MRI system in breast cancer: a feasibility study. Eur J Radiol. 2017; 86:261–266.


10. Aghaei F, Tan M, Hollingsworth AB, Qian W, Liu H, Zheng B. Computer-aided breast MR image feature analysis for prediction of tumor response to chemotherapy. Med Phys. 2015; 42:6520–6528.


11. Dorrius MD, Jansen-van der Weide MC, van Ooijen PM, Pijnappel RM, Oudkerk M. Computer-aided detection in breast MRI: a systematic review and meta-analysis. Eur Radiol. 2011; 21:1600–1608.


12. Kim BS. Usefulness of breast-specific gamma imaging as an adjunct modality in breast cancer patients with dense breast: a comparative study with MRI. Ann Nucl Med. 2012; 26:131–137.


13. Johnson N, Sorenson L, Bennetts L, Winter K, Bryn S, Johnson W, et al. Breast-specific gamma imaging is a cost effective and efficacious imaging modality when compared with MRI. Am J Surg. 2014; 207:698–701. discussion 701.


14. Brem RF, Floerke AC, Rapelyea JA, Teal C, Kelly T, Mathur V. Breast-specific gamma imaging as an adjunct imaging modality for the diagnosis of breast cancer. Radiology. 2008; 247:651–657.


15. Tan H, Zhang H, Yang W, Fu Y, Gu Y, Du M, et al. Breast-specific gamma imaging with Tc-99m-sestamibi in the diagnosis of breast cancer and its semiquantitative index correlation with tumor biologic markers, subtypes, and clinicopathologic characteristics. Nucl Med Commun. 2016; 37:792–799.


16. Tadwalkar RV, Rapelyea JA, Torrente J, Rechtman LR, Teal CB, McSwain AP, et al. Breast-specific gamma imaging as an adjunct modality for the diagnosis of invasive breast cancer with correlation to tumour size and grade. Br J Radiol. 2012; 85:e212–e216.


17. Baltzer PA, Vag T, Dietzel M, Beger S, Freiberg C, Gajda M, et al. Computer-aided interpretation of dynamic magnetic resonance imaging reflects histopathology of invasive breast cancer. Eur Radiol. 2010; 20:1563–1571.

