Abstract
Background
Connective tissue growth factor (CTGF) is a multifunctional cellular protein and playing a role as a central mediator in tissue remodeling and fibrosis. The physiological function of CTGF in psoriasis is unknown.
Objective
The purpose of this study was to investigate the function of CTGF in psoriasis using the established imiquimod (IMQ)-induced psoriasis murine model and psoriasis patients.
Methods
Anti-CTGF monoclonal antibody was applied to IMQ induced psoriasis mice and those skin were clinically, pathologically and immunologically analyzed. Additionally, CTGF expression was analyzes using skin samples and plasma from psoriasis patients.
Results
CTGF expression was observed in the dermis from both IMQ-induced psoriatic mice and psoriasis patients. CTGF inhibition using an anti-CTGF antibody slightly worsened IMQ-induced dermatitis. In addition, the increase of CTGF showed tendency to suppress the psoriatic dermatitis through inhibition of suprabasal cells proliferation and macrophage infiltration in the skin. CTGF was also detected significantly higher in plasma from psoriasis patients comparing with healthy control.
Connective tissue growth factor (CTGF, also known as CCN2) is a member of the CCN family of proteins that contribute to a variety of cellular processes, including angiogenesis, chondrogenesis, wound healing, and organ fibrosis1. CTGF is known to be a full-length molecule consisting of four conserved domains, i.e., insulin-like growth factor-binding protein domain (module 1: M1), von Willebrand factor type C domain (module 2: M2), thrombospondin type I repeat domain (module 3: M3) and C-terminal cysteine-knot I repeat domain (module 4: M4). CTGF overexpression is induced by transforming growth factor-β in many pro-fibrotic conditions such as scleroderma12. In articular tissue, CTGF is produced by chondrocytes and maintains cartilage tissue homeostasis via an autocrine process3. We previously demonstrated that tumor necrosis factor (TNF)-α inhibited CTGF production in human chondrocytes, whereas TNF-α induced CTGF in human synovial cells4. Based on those results, we found that a neutralizing anti-CTGF monoclonal antibody significantly ameliorated arthritis in collagen-induced mice5.
Psoriasis is a chronic inflammatory skin disease characterized by epidermal hyperplasia and dermal infiltration of immune cells. Cytokines, such as interleukin-17A and TNF-α, produced by T helper cells are implicated in disease pathogenesis6. Since the development of biological agents, anti-TNF-α antibodies have been used for the treatment of psoriasis7. Anti-TNF-α antibody therapy also improved the treatment of rheumatoid arthritis (RA). Psoriasis and RA have a commonality in terms of pathogenesis and treatment8. However, the function and role of CTGF are unknown in psoriasis.
In this study, we investigated the function of CTGF in psoriasis using the established imiquimod (IMQ)-induced psoriasis murine model9 and samples from psoriasis patients.
BALB/c mice were obtained from Charles River Japan (Yokohama, Japan). Experiments were performed in compliance with institutional guidelines and were approved by the Institutional Animal Care and Use Committee (IACUC) of Juntendo University (Approval no. 2900053). At eight weeks of age, mice were shaved on the back skin and left ear, and 62.5 µl and 3.125 µl of 5% IMQ cream (Beselna Cream; Mochida Pharmaceutical, Tokyo, Japan) were respectively applied to these areas for six days. One day before IMQ application, 200 µg of anti-CTGF monoclonal antibody (Nosan Corporation, Yokohama, Japan) was intraperitoneally injected. An objective scoring system called the psoriasis severity index, which considers erythema, scaling, and thickness, was used, and skin lesions were scored from 0 to 4. The cumulative score (erythema plus scaling plus thickening) was also employed for measuring severity (scored from 0 to 12)9.
The human subject research component was approved by the Juntendo University Urayasu Hospital Institutional Review Board (IRB no. 2009-009). After informed consent, 4-mm punch biopsies were obtained from both psoriasis patients (n=6) and other disease patients with normal condition of skin (n=4). The diagnosis was confirmed by both pathologists and dermatologists. Plasma was also collected from patients with psoriasis (n=28) and healthy control (n=8).
Mouse and human skin samples were fixed in a 20% formalin solution and embedded in paraffin, sectioned, and subjected to hematoxylin-eosin (H&E) staining, immunofluorescence, and immunohistochemistry. Epidermal and dermal thickness were determined at five positions per section by measuring the average distance from the basal lamina to the bottom of the stratum corneum using a BZ-II Analyzer ver. 1.1 (Keyence, Osaka, Japan). For immunofluorescence staining, back skin samples were blocked and stained using goat anti-CTGF antibody (Santa Cruz Biotechnology, Santa Cruz, CA, USA), rat anti-Ki-67 antibody (eBioscience, Sun Diego, CA, USA), rat anti-CD3 antibody (Abcam plc, Cambridge, UK), and biotin conjugated Armenian hamster anti-Vγ3 antibody (Leinco Technologies Inc., St. Louis, MO, USA). Sections were further incubated with Alexa Fluor 488 conjugated anti-goat IgG (Invitrogen, Carlsbad, CA, USA) or anti-rat IgG (Invitrogen), and Alexa Fluor 594 conjugated streptavidin (BioLegend, Sun Diego, CA, USA). Nuclei were stained with 4′,6-diamidino-2-phenylindole. For immunohistochemical staining, sections were incubated with primary rat anti-F4/80 antibody (Abcam plc, Cambridge, UK), rat anti-Ly-6G antibody (BD Biosciences, San Diego, CA, USA), Armenian hamster anti-CD11c antibody (BioLegend), and rat anti-PDCA1 antibody (DENDRITICS, Lyon, France). Sections were further incubated with horseradish peroxidase-conjugated anti-rat IgG (Dako, Glostrup, Denmark) and anti-Armenian hamster IgG secondary antibody (Jackson Immunoresearch Laboratories, West Grove, PA, USA). Immunoreactivity was detected by incubating with a DAB+ Liquid kit (Dako). Sections were then counterstained with hematoxylin. For evaluation of leukocyte infiltration in the skin, three researchers quantified the staining of three sections from all mice. The numbers of cells positive for F4/80, Ly-6G, PDCA-1, CD3, and Vγ3 were quantified in each section. Photomicrographs were taken using a BZ-9000 microscope (Keyence).
Skin tissues were collected and homogenized. Ten micrograms of total protein was loaded in each well. The primary antibodies used were anti-CTGF antibody (Santa Cruz) and anti-GAPDH antibody (Millipore, Billerica, MA, USA). Densitometric analysis was performed using ImageJ software (National Institute of Health, Bethesda, MD, USA).
Human CTGF enzyme-linked immunosorbent assay (ELISA) analysis was performed as described before1011. Briefly, plasma samples were added to 96 well-plates (ThemoFisher Scientific, Waltham, MA, USA) that were coated with a monoclonal antibody against human CTGF modules. A biotin–labeled anti-human CTGF monoclonal antibody and a peroxidase-conjugated streptavidin (Jackson Immuno Research, West Grove, PA, USA) were added, Substrate and stop solution were sequentially added. The optical density at 450 nm was read to microplate reader (ARVO SX; Perkin Elmer, MA, USA), and measure the CTGF concentration.
Data were expressed as the mean±standard deviation and analyzed using GraphPad Prism 6 software (La Jolla, CA, USA). Statistical significance was determined by the Mann-Whitney U test, 2-way ANOVA, and unpaired t-test with Welch's correction. A p-value <0.05 was considered statistically significant.
To identify CTGF expression in IMQ-induced psoriatic mice, we used immunohistological analyses and Western blotting (Fig. 1). Immunofluorescence tissue staining revealed that CTGF expression was increased in IMQ-induced psoriatic back dermal lesions compared with control IMQ untreated mice (Fig. 1A). To confirm CTGF protein expression levels, we performed a Western blot analysis using back skin samples. CTGF protein expression showed a tendency to increase in IMQ-induced psoriatic mice relative to untreated mice (Fig. 1B, left). However, the increased expression of CTGF was not statistically significant using this method (Fig. 1B, right).
To confirm the involvement of CTGF in this disease model, we neutralized CTGF using an anti-CTGF antibody. After starting the IMQ application, the scoring of erythema, scaling, and thickening was performed using the back skin of mice. Anti-CTGF administration did not affect the systemic immune system; for example, IMQ-induced splenomegaly was not affected (data not shown). Representative skin condition of anti-CTGF treated and untreated mice are exhibited in Fig. 2A. Anti-CTGF treated mice demonstrated declining trend comparing with untreated mice. The independent skin scores in a representative experiment are shown in Fig. 2B. Skin scores were slightly but significantly increased in CTGF-blocked mice several days after IMQ application. Pathological analysis demonstrated that the epidermal and dermal thickness of back skin (Fig. 2C) and ears (Fig. 2D) were not changed by CTGF blocking. However, more Ki-67 positive cells were detected at the epidermis boundary in dermis of anti-CTGF antibody-treated IMQ-induced psoriatic mice (Fig. 2E) and the difference between anti-CTGF treated and non-treated mice was statistically significant (Fig. 2F). Thus, these data based on the effect of anti-CTGF suggest CTGF slightly suppressed the proliferation of suprabasal cells.
Next, we evaluated the infiltration of immune cells in IMQ-induced psoriatic skin lesions. The numbers of macrophages, neutrophils, pan-dendritic cells (DCs), plasmacytoid DCs, pan-T cells, Vγ3− T cells (most of αβ T cells), and Vγ3+ T cells (γδ T cells) were counted. Macrophages were tended to increase in IMQ induced mice and showed significant increase in only CTGF-blocked mice (Fig. 3).
CTGF expression also increased in skin lesions from psoriasis patients comparing with healthy control (Fig. 4A). We previously reported that plasma CTGF levels from RA patients were significantly higher from healthy control4. The same analyzing methods demonstrated that CTGF levels from psoriasis patients were significantly higher from healthy control (Fig. 4B).
Overall, our results indicated that CTGF is involved in IMQ-induced psoriatic dermatitis and the neutralizing CTGF by anti-CTGF treatment could worsen this condition. CTGF expression in skin lesions showed similarity between IMQ-induced psoriatic mice and psoriasis patients. In addition, plasma CTGF levels increased in psoriasis patients comparing with healthy control.
The role of CTGF in psoriasis has not been previously reported. Genome-wide expression analysis has shown that IMQ induced-psoriasis does not fully mimic human psoriasis12. In this sense, the analysis of our study resulted in reflecting the limited condition in psoriasis. However, IMQ induced mice is the representative model of psoriasis about clinical and immunological status in the dermatitis. In the current study, we described novel functions of CTGF in psoriatic dermatitis using IMQ-treated mice and found that CTGF tended to increase in the dermis (Fig. 1). It is likely that the enhanced CTGF expression observed with IMQ treatment was associated with dermal fibroblasts1. As a result of neutralizing CTGF by anti-CTGF in IMQ-treated mice, the psoriatic score worsened (Fig. 2B). In skin lesions, suprabasal cells proliferated (Fig. 2E, F), and infiltration of immune cells, notably macrophages, significantly increased (Fig. 3).
Macrophages upregulate healing through their inflammatory and reparative phenotypes and the balance between inflammatory and repair phenotype of macrophages is crucial for successful wound healing13. The application of macrophages or stem cells onto diabetic rodent wounds has been shown to accelerate wound healing and epithelial closure14. In the current study, macrophages were tended to increase in IMQ dermatitis induced mice and showed significant increase in only CTGF-blocked mice using anti-CTGF (Fig. 3). This result suggested that CTGF inhibition may delay the process of wound healing and CTGF could induce repair phenotype of macrophages to improve skin lesions in psoriasis. Recent reports showed CTGF contribute wound repair,1516 and both CTGF and macrophages are involved in tissue remodeling17. However, another reports showed CTGF function as chemotactic factor for some lymphocytes18. In vivo CTGF function is still unclear. At least, our results suggested that reduction of CTGF by anti-CTGF could have an effect on worsening skin condition in psoriasis.
We demonstrated CTGF expression increased in skin lesions and plasma from psoriasis patients comparing with healthy control (Fig. 4). Taken together, CTGF could contribute to improve psoriatic skin lesions through inducing repair phenotype of macrophage in both IMQ-induced psoriatic mice and psoriasis patient.
In summary, the present data based on the effect of anti-CTGF treatment demonstrated that CTGF suppressed the progression of IMQ-induced psoriatic dermatitis. Additionally, CTGF expression was increased in skin lesions and plasma from psoriasis patients. Our findings suggest that CTGF could contribute to the healing rather than the worsening of psoriasis skin lesions including the induction of reparative macrophage.
Figures and Tables
Fig. 1
Connective tissue growth factor (CTGF) expression was increased in back skin lesions from imiquimod (IMQ)-induced psoriatic-like mice compared with control IMQ-untreated mice. BALB/c mice (n=4~6 per groups) were treated with IMQ cream on shaved back skin. On day 6 after IMQ treatment, samples were collected from back skin. (A) Representative immunofluorescent staining of CTGF (green) in back skins of control and IMQ-treated mice. Nuclei were stained with 4′,6-diamidino-2-phenylindole (blue). Arrowheads showed CTGF-expressing cells; bar: 50 µm. White line represents the border between the epidermis and dermis. (B) CTGF expression in back skins of control and IMQ-treated mice was evaluated by western blot analysis; scatterplot showing the results of densitometric analysis. IMQ(−): IMQ-untreated control mice, IMQ(+): IMQ-treated mice, GAPDH: glyceraldehyde-3-phosphate dehydrogenase.
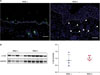
Fig. 2
Psoriatic scores were worsened because of blocking connective tissue growth factor (CTGF) in imiquimod (IMQ)-treated mice. BALB/c mice were treated daily with IMQ cream on shaved back skin and the left ear. One day before IMQ application, 200 µg of anti-CTGF monoclonal antibody was intraperitoneally injected (n=4~6 per groups). (A) Representative skin condition of anti-CTGF treated and untreated mice. These photos were taken on day 5 after IMQ application. (B) Erythema, scaling, and thickness of back skin was scored daily using a scale from 0 to 4; the cumulative score (0~12) is depicted. Symbols indicate mean score+standard deviation (n=4~6). **p<0.01, ***p<0.001, ****p<0.0001, by 2-way ANOVA (IMQ[+] vs. IMQ[+], anti-CTGF). (C) Epidermal and dermal thicknesses were measured in back skin samples. (D) Epidermal thickness in ear samples. (E) Immunohistochemically staining of Ki-67 (green) in back skin. Nuclei were stained with 4′,6-diamidino-2-phenylindole (blue); bar: 50 µm. Yellow line represents the border between the epidermis and dermis. (F) Scatterplot of epidermal Ki-67+ cell number. *p<0.05 by Mann-Whitney U test (IMQ[+] vs. IMQ[+], anti-CTGF).
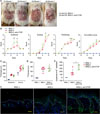
Fig. 3
Immune cell infiltration into imiquimod (IMQ)-induced psoriatic skin lesions was altered by connective tissue growth factor (CTGF) blocking. The composition of the leukocyte infiltration was analyzed using the markers F4/80 (macrophage), Ly-6G (neutrophil), CD11c (pan-dendritic cell ([DC]), PDCA-1 (plasmacytoid DC [pDC]), CD3 (pan-T cell), and Vγ3 (γδ T cell). The numbers of macrophages, neutrophils, pan-DC, pDC, pan-T cells, Vγ3− T cells (most of αβ T cells), and Vγ3+ T cells were counted on three fields per visual field. Values are shown as the mean±standard deviation of the mean (n=4~6). *p<0.05 by Mann-Whitney U test (IMQ[+] vs. IMQ[+], anti-CTGF).
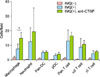
Fig. 4
Connective tissue growth factor (CTGF) expression was increased in skin lesions and plasma from psoriasis patients. (A) Representative immunofluorescent staining of CTGF (green) in skins of healthy control (n=4) and psoriasis patients (n=6). Nuclei were stained with 4′,6-diamidino-2-phenylindole (blue). Arrowheads showed CTGF-expressing cells; bar: 50 µm. White line represents the border between the epidermis and dermis. (B) CTGF in plasma from healthy control (HC) and psoriasis patients was detected by a sandwich enzyme-linked immunosorbent assay (ELISA) analysis. Blue circle: HC (n=10), red rectangle: psoriasis patients (psoriasis, n=28). Values are shown as the mean±standard deviation of the mean. ****p<0.0001 by unpaired t-test with Welch's correction.
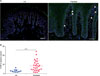
ACKNOWLEDGMENT
This study was supported in part by a Grant-in-Aid (S1311011) from MEXT (Ministry of Education, Culture, Sports, Science and Technology) Supported Program for the Strategic Research Foundation at Private University and by a JSPS (Japan Society for the Promotion of Science) KAKENHI grant numbers JP26860265 (to KH) and JP15K08107 (to KI). The authors would like to thank Ms. Megumi Morioka (Nosan Corporation) for the provision of anti-CTGF monoclonal antibody, Dr. Mitsutoshi Tominaga, Dr. Utako Kimura and the members of our laboratory for technical support and helpful discussion.
References
1. Leask A, Denton CP, Abraham DJ. Insights into the molecular mechanism of chronic fibrosis: the role of connective tissue growth factor in scleroderma. J Invest Dermatol. 2004; 122:1–6.


2. Igarashi A, Nashiro K, Kikuchi K, Sato S, Ihn H, Grotendorst GR, et al. Significant correlation between connective tissue growth factor gene expression and skin sclerosis in tissue sections from patients with systemic sclerosis. J Invest Dermatol. 1995; 105:280–284.


3. Takigawa M, Nakanishi T, Kubota S, Nishida T. Role of CTGF/HCS24/ecogenin in skeletal growth control. J Cell Physiol. 2003; 194:256–266.


4. Nozawa K, Fujishiro M, Kawasaki M, Kaneko H, Iwabuchi K, Yanagida M, et al. Connective tissue growth factor promotes articular damage by increased osteoclastogenesis in patients with rheumatoid arthritis. Arthritis Res Ther. 2009; 11:R174.


5. Nozawa K, Fujishiro M, Kawasaki M, Yamaguchi A, Ikeda K, Morimoto S, et al. Inhibition of connective tissue growth factor ameliorates disease in a murine model of rheumatoid arthritis. Arthritis Rheum. 2013; 65:1477–1486.


6. Lowes MA, Suárez-Fariñas M, Krueger JG. Immunology of psoriasis. Annu Rev Immunol. 2014; 32:227–255.


7. Reich K, Nestle FO, Papp K, Ortonne JP, Evans R, Guzzo C, et al. Infliximab induction and maintenance therapy for moderate-to-severe psoriasis: a phase III, multicentre, double-blind trial. Lancet. 2005; 366:1367–1374.


8. Coates LC, FitzGerald O, Helliwell PS, Paul C. Psoriasis, psoriatic arthritis, and rheumatoid arthritis: is all inflammation the same? Semin Arthritis Rheum. 2016; 46:291–304.


9. van der Fits L, Mourits S, Voerman JS, Kant M, Boon L, Laman JD, et al. Imiquimod-induced psoriasis-like skin inflammation in mice is mediated via the IL-23/IL-17 axis. J Immunol. 2009; 182:5836–5845.


10. Miyazaki O, Kurashita S, Fukamachi I, Endo K, Ng PS, Takehara K. Subtraction method for determination of N-terminal connective tissue growth factor. Ann Clin Biochem. 2010; 47:205–211.


11. Fujishiro M, Yamaguchi A, Kawasaki M, Nozawa K, Takasaki Y, Takamori K, et al. The detection of plasma levels of connective tissue growth factor in rheumatoid arthritis patients. Clin Exp Rheumatol. 2012; 30:145–146.
12. Swindell WR, Johnston A, Carbajal S, Han G, Wohn C, Lu J, et al. Genome-wide expression profiling of five mouse models identifies similarities and differences with human psoriasis. PLoS One. 2011; 6:e18266.


13. DiPietro LA. Wound healing: the role of the macrophage and other immune cells. Shock. 1995; 4:233–240.
14. Waugh HV, Sherratt JA. Macrophage dynamics in diabetic wound dealing. Bull Math Biol. 2006; 68:197–207.


15. Alfaro MP, Deskins DL, Wallus M, DasGupta J, Davidson JM, Nanney LB, et al. A physiological role for connective tissue growth factor in early wound healing. Lab Invest. 2013; 93:81–95.


16. Henshaw FR, Boughton P, Lo L, McLennan SV, Twigg SM. Topically applied connective tissue growth factor/CCN2 improves diabetic preclinical cutaneous wound healing: potential role for CTGF in human diabetic foot ulcer healing. J Diabetes Res. 2015; 2015:236238.

