Abstract
The field of neuro-oncology is evolving rapidly. Many important advances have recently been reported, and other promising investigations have the potential to soon make substantial impacts in the field, especially in the areas of high-grade gliomas and brain metastases. We present an overview of the current status of this field, highlighting the key recent advances as well as representative work of key clinical investigations, since these concepts have the potential to influence clinical management if they are demonstrated to be safe and efficacious. This overview includes some work that has only appeared in abstract form in order to provide a timely understanding of how the field is actively changing and what may lie on the horizon. We focus on both medical and surgical neuro-oncology advances in this highly multidisciplinary subspecialty.
The field of neuro-oncology is evolving rapidly, with promising ongoing investigations having the potential to influence clinical management within the short- to medium-term future. This review focuses on both the surgical and medical advances in this field. The disciplines of neurosurgical oncology and medical neuro-oncology are being advanced by investigators from both these and other backgrounds. The success of these and other research endeavors, as well as in clinical care, is facilitated by the close integration of multiple disciplines. This review attempts to be comprehensive, but it is impossible to cover all of the exciting developments in this field.
Neurosurgical intervention has been an essential diagnostic and therapeutic component of the management of central nervous system (CNS) tumors. The goal of neurosurgical intervention for CNS malignancies is threefold: 1) obtaining tissue for diagnosis and for developing personalized therapies, 2) complete removal of the lesion when possible, and 3) local delivery of therapeutics. In this update, we first discuss ongoing and future developments in the neurosurgical management of both metastatic and primary CNS tumors, before shifting to the medical management of high-grade gliomas (HGG). The overall management of CNS metastases1 and gliomas2 has been reviewed recently, and so here we focus on how neurosurgical interventions will feature within the wider context of the ongoing advances in neuro-oncology.3
The current standard of care for multiple brain metastases primarily involves radiation therapy. This is delivered either as whole-brain radiation therapy (WBRT), which is administered in multiple fractions typically over 1–3 weeks, or as stereotactic radiosurgery (SRS), where single larger doses are focused on smaller target volumes. While there is no absolute cutoff for the number or volume of brain metastases appropriate for SRS, randomized data support its utilization in patients with four or fewer lesions. Either surgical resection or SRS is considered in patients with a single brain metastasis. Several factors influence the decision-making, including the size of the lesion, symptomatology, comorbid medical conditions, the age of the patient, and the underlying histology.1
Surgical resection has played a major role for many years in the treatment of single brain metastases. Significant improvements in both overall survival (OS) and functional independence have been achieved by using surgery in conjunction with postoperative radiation versus WBRT alone.4 Recent advancements in the technologies used in the field of neuroimaging and for the intraoperative monitoring of vital structures have further expanded the role of neurosurgery to the safe removal of lesions involving eloquent locations. Imaging modalities such as magnetic resonance imaging (MRI)-based diffusion-tensor imaging (DTI), functional MRI (fMRI), and MRI-guided electrical targeting and electromyography (transcranial magnetic stimulation) enhance preoperative planning by delineating the lesion from major tracts and eloquent cortical areas.5 In addition, sophisticated intraoperative monitoring using direct cortical stimulation can help overcome the limitation of static preoperative imaging, which does not account for intraoperative changes in the brain position. These modalities can also be used in combination to safely remove lesions from the eloquent cortex without causing major neurological deficits.6
The role of neurosurgical intervention in the setting of multiple metastases is less clear. However, important recent work has delineated a branched evolution with distinct mutations in the primary tumor, its brain metastases, and its extra-CNS metastases. Approximately half of all solid-tumor brain metastases are associated with clinically informative genomic alterations that are not found in the primary tumor. Of potentially equal importance, the genomic profiles of multiple brain metastases that are temporally and spatially distinct are relatively homogeneous.7 While the genomic signature of the brain metastases does not yet routinely influence clinical management, this may change in the future. Investigations of agents that can impact actionable targets in brain metastases may make it necessary to obtain tissue from these metastases for genetic and epigenetic profiling, as well as protein expression. A targeted therapy could be similarly effective throughout the brain compartment due to the homogeneity of brain metastases in a specific patient. It will be beneficial to further integrate neurosurgical care with the other components of multidisciplinary decision-making and care. There are ongoing investigations of treatments addressing specific mutational targets, with substantial interest regarding the initiation of these studies and their future results. Additionally, the validation of predictive biomarkers for how metastases respond to immunotherapies, such as checkpoint inhibitors including programmed death 1 (PD-1) and its ligand (PD-L1) antibodies, will increase the utility of obtaining tissue from brain metastases in guiding management. There is a long but interesting road ahead involving the investigation and validation of these concepts.
In addition to the key diagnostic role, there have been interesting advances in neurosurgical therapeutic interventions for brain metastases. One particular technique that was initially evaluated several decades ago is becoming more widely utilized: laser-induced thermal therapy (LITT), sometimes called interstitial laser ablation, involves the stereotactic placement of a catheter that precisely heats the target lesion under direct MRI guidance.8 This modality is used in the management of both brain metastases and the radiation-induced necrosis resulting from their treatment.9 A retrospective single-institution study of 102 procedures demonstrated the feasibility and relative safety of this modality, but it also highlighted a learning curve that needs to be overcome.10 While this therapeutic modality holds promise, the results of few prospective trials have been published thus far, and so larger and more rigorous studies are required to establish its role as an effective therapeutic modality. The median OS in that population of carefully selected patients with heterogeneous histologies was 19.8 months. As the use of LITT increases, familiarity with the radiographic changes indicating a transient increase in lesion size followed by a slower decrease in lesion size will be important.11 In addition to brain metastases, LITT is also being used in the management of both newly diagnosed12 and recurrent13 primary brain tumors.
Infiltrating gliomas compose a large and important group of primary brain tumors and are the focus of many neuro-oncology programs. The management of infiltrating gliomas entails the maximum safe surgical resection, often followed by radiation and chemotherapy. This has been studied the most in HGG, particularly glioblastoma (grade IV). In glioblastoma, surgery or biopsy is often followed by radiation with concomitant chemotherapy using the oral alkylating agent temozolomide, and then additional adjuvant cycles of temozolomide. The management is less clear for progressive disease. Systemic therapeutic options include the nitrosourea CCNU as well as the antiangiogenic antibody bevacizumab.2
As stated above, the first goal of surgery is to establish a diagnosis that helps guide subsequent management. The World Health Organization (WHO) has recently revised its classification system for CNS tumors.1415 The latest revision employs a layered diagnostic approach that combines histological and molecular features to define the diseases, thereby providing a better understanding of the tumor biology and its association with the prognosis. Some of the most important changes have taken place in the context of infiltrating gliomas. The mutational status of genes coding for isocitrate dehydrogenase (IDH), IDH1 and IDH2, yields a clear divide between two groups of infiltrating gliomas. IDH1 and IDH2 are the most commonly mutated metabolic genes in human cancers.16 When mutated, they simultaneously lead to loss of normal function, the production of alpha-ketoglutarate (αKG), the acquisition of neomorphic function, the reduction of αKG to D-2-hydroxyglutarate (2-HG), and a decrease in NADPH production.1718 IDH mutant gliomas are phenotypically and genetically distinct from their IDH wild-type counterparts.1920 Mutation of IDH1 or IDH2 is thought to be a very early genetic event in tumorgenesis in a subset of infiltrating gliomas, and it defines distinct epigenetic and biological subgroups in glioma.2122 Such mutations are present in most gliomas of grades II and III and in a smaller subset (~10%) of glioblastomas, and have been associated with a better prognosis in glioma.23 Although some studies have suggested that IDH-mutated gliomas respond better to chemotherapy than do those with wild-type IDH, the predictive values of IDH mutations has not been established. In addition to the prognostic value of IDH mutations, their functional effect on seizure frequency have recently been described. A 2-HG-mediated epileptogenesis has recently been described, which appears to be reversed by the N-methyl-D-aspartate (NMDA) receptor blockade.24 Findings such as these reflect historical observations (favorably prognostic low-grade gliomas presenting with seizures) and, more importantly, present therapeutic potential.
Another change in the new classification is the use of the 1p19q chromosomal codeletion as a defining feature of oligodendrogliomas. There is a subset of patients with tumors exhibiting oligodendroglial histology but lacking the 1p19q codeletion. In the past, these tumors would have been classified oligodendrogliomas, but now they would be categorized as astrocytomas. Molecular analyses would probably detect a molecular phenotype more consistent with an astrocytoma. While the category of oligoastrocytoma remains in the new classification system, the characteristics required for a tumor to qualify for this nebulous diagnosis are much tighter than in the past.1415 These above-described revisions in the classification for infiltrating gliomas will become incorporated into future clinical trials and will need to be taken into account when considering the results of both previous and ongoing trials.
Ongoing work in the realm of primary brain tumors is focused on optimizing the safety of achieving gross total resection in the setting of low-grade gliomas and effective tissue procurement, as well as drug delivery in the context of HGG. As discussed above, advanced imaging modalities, intraoperative monitoring, and intraoperative MRI (iMRI) have significantly improved our ability to achieve gross total resection of gliomas. Recent studies have supported that using iMRI for glioma resection can achieve a greater extent of resection in some patients and may provide significant survival benefits.2526
Recent advances in cortical mapping have been adopted in neuro-oncology. Preoperative imaging mapping technologies, including fMRI and DTI, provide surgical planning tools that surgeons may use to improve surgical safety in intra-axial tumor surgery. These functional imaging modalities along with intraoperative cortical stimulation mapping and performing surgery in awake patients are more commonly used in glioma surgery. These techniques, combined with intraoperative imaging, show promise in extending the extent of the safe resection of intra-axial tumors.
With the evolution of the concept of minimally invasive brain surgery and miniature tubular retraction systems as an alternative to stereotactic brain biopsy,2728 surgery is also being used to harvest a substantial amount of tumor tissue for the development of patient-specific vaccines, to deliver oncolytic viruses, and to administer viral vectors for gene therapy or stem cells as carriers of therapeutic agents. All of this work is occurring within the context of clinical trials. Since it is impossible to be completely inclusive, we highlight a few key studies that serve as exemplars of the concepts.
Surgery has the potential to play two roles within the context of vaccine-based therapies: 1) it can be used to obtain tumor tissue for creating personalized vaccines for use in tumor-derived vaccine strategies and 2) it can used for cytoreduction in all vaccine strategies. Cytoreduction is hypothesized to decrease the overall number of cells contributing to local immune suppression, potentially facilitating immune-modulating therapy. Cytoreduction also has the potential to create space intracranially that can accommodate any inflammation and associated swelling occurring when immunotherapy is applied. One of the best-studied tumor-derived vaccine therapies is the HSP peptide complex 96 vaccine (HSPPC-96, Prophage), which was created by combining patient-derived tumor-associated antigens and the chaperone protein HSP gp96. Studies of gross total resection of recurrent gliomas followed by intradermal injection of the vaccine found a favorable OS (42.6 weeks) and 12-month survival (29.3%) when compared to historical controls.29 A randomized phase II cooperative group trial (NCT01814813) evaluated the efficacy in recurrent glioblastoma either as a single agent or in combination with antiangiogenic therapy. That study randomized patients (after resection and vaccine development) to one of three arms: 1) vaccine plus the antiangiogenic agent bevacizumab, 2) vaccine followed by bevacizumab at progression, or 3) bevacizumab alone. An interim analysis unfortunately demonstrated no efficacy, but there are ongoing plans to explore this therapeutic modality in other combination regimens.
Surgery can also be used to deliver an oncolytic virus to the tumor.30 An example of this is the phase I trial of PVSRIPO for recurrent glioblastoma, in which patients undergo a stereotactic biopsy and intratumoral infusion of the recombinant nonpathogenic poliovirus:rhinovirus chimera via a catheter. In this dose-escalation study, the 24-month survival (23.3%) appears favorable when compared to historical controls, and it is suspected that outcomes could improve when the dose is optimized.31 A randomized phase II trial (NCT02986178) comparing PVSRIPO alone to PVSRIPO combined with the nitrosourea lomustine has recently commenced, which will provide additional safety and efficacy data and help clarify if oncolytic viral approaches should be pursued in conjunction with cytotoxic chemotherapies.
Surgery can also be used to deliver a viral vector for gene therapy. We discuss two approaches: 1) using a gene that induces the production of a compound that potentially has direct benefits and 2) using a gene that secretes a product that can convert a prodrug into its active metabolite within the tumor. Ad-RTS-hIL12, a modified adenoviral vector being investigated in recurrent grade-III and -IV gliomas, delivers a gene to intracranial tumor cells that induces them to secret the proinflammatory cytokine IL-12. That trial currently applies either a craniotomy with surgical resection followed by intraoperative freehand injection or the stereotactic injection of the viral vector. The gene is activated by the administration of the oral agent veledimex, which leads to the tumoral secretion of IL-12. This approach aims to overcome the strong immune suppression associated with HGG and circumvent the toxicity of systemically administered high-dose IL-12. Preliminary results have been presented recently at the annual meeting of the American Society for Clinical Oncology. The elevation of serum IL-12 and interferon gamma observed in patients treated with this strategy validates the proposed mechanism of action of the drug. Moreover, the therapy appears to be well tolerated. While there are currently few data on response rates and progression-free survival (PFS), the OS (12.7 months) findings are favorable when compared to historical controls.32
The second approach uses surgery as a means to deliver a viral vector containing a gene whose product converts the antifungal prodrug 5-fluorocytosine (5-FC) into the active 5-fluorouracil (5-FU), a potent chemotherapeutic agent, within the tumor.33 Toca 511 is a nonlytic retroviral replicating vector that transmits the transgene-encoding enzyme cytosine deaminase specifically to the replicating tumor cells. Cytosine deaminase (CD) converts 5-FC into 5-FU,34 and this approach is being investigated both in the context of newly diagnosed and recurrent gliomas. Several routes for vector delivery are being tested, including intratumoral delivery using a biopsy needle, direct injection into the resection cavity following surgical debulking, and intravenous systemic delivery. Although the preclinical studies have indicated robust survival benefits, outcome data from these clinical trials are not yet available.
While viral vectors are an effective way to transfer genes, this approach is restricted by the ability of the viral vector to diffuse away from the site of the initial injection. Using tumor-tropic neural stem cells (NSCs)–which are genetically modified to express various transgenes–can overcome this limitation with respect to diffusion. NSCs are emerging as a very attractive transgene delivery system due to their natural tumor tropism and impressive preclinical safety profile.353637 Genetically modified NSCs expressing enzyme CD, which converts the prodrug 5-FC into the active drug 5-FU, is currently being investigated in a clinical trial (NCT02015819) both in the context of newly diagnosed and recurrent gliomas. As part of that study, modified NSCs are initially directly injected into the tumor cavity following surgical resection and then readministered via a Rickham reservoir implanted during the surgery. Moreover, durable complete radiographic responses have been seen in a different phase-I trial. It is possible that these responses may be more likely in IDH-mutated tumors.38 Another recently initiated trial is using locally administered NSCs to deliver an oncolytic adenovirus to newly diagnosed HGG in conjunction with standard radiation and chemotherapy (NCT03072134). However, definitive clinical outcomes–in terms of the efficacy of these strategies in prolonging OS or PFS–have yet to be established.
We have already discussed surgical advances focusing on LITT as well as surgery as a delivery method for viral and gene therapies or as a method for collecting tissue for the creation of tumor-specific vaccines. We now focus on two types of nonsurgical therapeutic modalities: 1) tumor treating fields (TTF) and 2) systemically delivered immunotherapies.
The mitotic apparatus of dividing cells is disrupted when they are placed in strong electrical fields, which leads to cell-cycle arrest and apoptosis. Both the frequency of the electrical fields and the cell orientation with respect to the field influence the effects on the cells. This methodology has been applied to humans with brain tumors via the direct application of four arrays to the shaved scalp (Fig. 1). This treatment modality was initially evaluated using recurrent glioblastoma (EF-11 trial), followed by the newly diagnosed setting (EF-14 trial).39 Recently reported results for the full cohort of patients in the EF-14 randomized phase III trial40 have confirmed the published results for the initial 315 patients.41 The patients with newly diagnosed glioblastoma in this trial all received radiation with concomitant temozolomide followed by adjuvant temozolomide. They were randomized at a ratio of 1:2 to the therapy described above and the same regimen with the addition of TTF in the adjuvant phase. The outcomes for several clinically relevant endpoints were significantly better in the investigational arm, including OS (21 months vs. 16 months), PFS (6.7 months vs. 4.0 months), and 4-year survival (17% vs. 10%). All of these results occurred within the context of minimally increased toxicity, specifically low-grade cutaneous toxicity,40 and the improvement in the tail of the Kaplan-Maier curve is particularly encouraging.
Similar improvements seen in the tail of the survival curve for other malignancies when using immunotherapies has led to excitement about their potential in HGG. The FDA has not yet approved any immunotherapy for the treatment of primary brain tumors. Several concepts are currently being investigated in these patients, including active immunotherapy, passive immunotherapy, and immunomodulator therapy, with a focus on developing vaccine therapies and on the blockade of immune checkpoints. We have already discussed viral and stem-cell based therapies; we now shift to vaccine therapies, which are the best-studied immunotherapies in HGG, and consider immune-checkpoint inhibitors, followed by other modulators of the immune system.
Educating the immune system about specific targets that should be attacked is the main concept underlying immune-based therapies. These vaccines can be subclassified into tumor-specific versus “off the shelf” vaccines. We have already discussed tumor-specific vaccines in our discussion of the role of surgery in neuro-oncology, and so here we focus on therapies utilizing “off the shelf” vaccines, in which every patient receives the same agent. The best studied of these is rindopepimut (formerly CDX-110), which targets the epidermal growth factor receptor (EGFR) and its constitutively active mutant variant, EGFRvIII. This vaccine is administered intradermally and has been evaluated in both newly diagnosed and recurrent glioblastomas. The ReACT randomized phase-II trial for recurrent glioblastomas evaluated rindopepimut plus the antiangiogenic agent bevacizumab (Avastin) versus bevacizumab alone, and demonstrated improvements in OS (11.6 months vs. 9.3 months), 6-month PFS (28% vs. 16%), and overall response rate (30% vs. 18%). Additionally, the patients in the rindopepimut arm also exhibited robust antibody titers against EGFRvIII, with these titers being correlated with OS.42 Unfortunately, the planned interim analysis of the ACTIV randomized phase-III trial evaluating radiation with concomitant and adjuvant temozolomide versus the same regimen with the addition of rindopepimut found no evidence of efficacy.43 However, there is still interest in utilizing vaccine therapies in conjunction with other immunotherapies, particularly in light of the notable tolerability to vaccine-based approaches.
The inhibition of immune checkpoints, particularly PD-1 and its ligand PD-L1, blocks the signals that impede immune attacks on tumors, thereby facilitating an effective immune response that enhances tumor killing. PD-1 is expressed on immune cells while PD-L1 is expressed on tumor, nontumor, and immune cells. Antibodies that block both PD-1 and PD-L1 have demonstrated efficacy in other malignancies. Improvements in OS as well as prolonged sustained responses have been seen in aggressive metastatic cancers,43444546 which has led to these agents being investigated in HGG. The PD-1 antibodies nivolumab and pembrolizumab are being investigated in the various CHECKMATE and KEYNOTE trials, respectively. While the final results of these studies have not yet been published, preliminary analyses have demonstrated safety and tolerability of these agents in HGG.47484950 Unfortunately, the randomized phase-III CHECKMATE 143 trial (NCT02017717) evaluating single-agent nivolumab in recurrent glioblastoma did not demonstrate superiority relative to bevacizumab alone in this patient population; these results were presented at the 2017 World Federation of Neuro-Oncology societies (WFNOS) meeting.51 There are ongoing studies [CHECKMATE 498 (NCT02617589) and CHECKMATE 548 (NCT 02667587)] of nivolumab in newly diagnosed glioblastoma, the results of which are eagerly awaited. The use of PD-L1 antibodies in this patient population is in a less-advanced stage of investigation. Preliminary studies of the PD-L1 antibodies atezolizumab and durvalumab have demonstrated their safety and tolerability.5253 The inhibition of other promising contributors to the immunosuppressive microenvironment, such as indoleamine-2,3-dioxygenase (IDO) and colony-stimulating Factor 1 receptor (CSF1R), are also being investigated in HGG.5455
Numerous advances in surgical and medical neuro-oncology are actively shaping the current and future care standards for the management of brain metastases and HGG (Table 1). Neurosurgical interventions form an integral part of comprehensive neuro-oncology care. New advances in technology and scientific breakthroughs in advanced therapeutic options continue to refine and redefine the role of surgery in the care of patients with neuro-oncology problems. However, the results from the early-phase studies as discussed herein indicate that caution remains necessary and that we must not develop overly optimistic expectations. The promise of both rejuvenated old modalities and novel new ones should be tempered by the need to validate the currently available initial results.
There are many concepts currently being investigated that we hope will positively influence the therapeutic management of metastatic and primary CNS tumors. Recent efficacy data for TTF in newly diagnosed glioblastoma has led to FDA approval of this approach. There are ongoing considerations regarding how this relatively nontoxic therapy will integrate with ongoing and prospective trials of other agents for both newly diagnosed disease and progressive disease. When contemplating the panoply of options in the wide armamentarium of immunotherapies, it will be interesting to learn which agents–or, more likely, a combination of agents–prove beneficial in treating HGG. It will also be of interest to see how this promising class of agents will be incorporated into treatment paradigms involving radiation, cytotoxic chemotherapies, antiangiogenic agents, and now TTF.
References
1. Lukas RV, Gabikian P, Garza M, Chmura SJ. Treatment of brain metastases. Oncology. 2014; 87:321–329. PMID: 25227433.


2. Lukas RV, Mrugala MM. Pivotal therapeutic trials for infiltrating gliomas and how they affect clinical practice. Neuro-Oncol Pract. 2016; DOI: 10.1093/nop/npw016.


3. Desjardins A, Vlahovic G, Friedman HS. Vaccine therapy, oncolytic viruses, and gliomas. Oncology (Williston Park). 2016; 30:211–218. PMID: 26984213.
4. Patchell RA, Tibbs PA, Walsh JW, Dempsey RJ, Maruyama Y, Kryscio RJ, et al. A randomized trial of surgery in the treatment of single metastases to the brain. N Engl J Med. 1990; 322:494–500. PMID: 2405271.


5. Coburger J, Musahl C, Henkes H, Horvath-Rizea D, Bittl M, Weissbach C, et al. Comparison of navigated transcranial magnetic stimulation and functional magnetic resonance imaging for preoperative mapping in rolandic tumor surgery. Neurosurg Rev. 2013; 36:65–75. discussion 75-76. PMID: 22886323.


6. Nimsky C, Bauer M, Carl B. Merits and limits of tractography techniques for the uninitiated. Adv Tech Stand Neurosurg. 2016; (43):37–60. PMID: 26508405.


7. Brastianos PK, Carter SL, Santagata S, Cahill DP, Taylor-Weiner A, Jones RT, et al. Genomic characterization of brain metastases reveals branched evolution and potential therapeutic targets. Cancer Discov. 2015; 5:1164–1177. PMID: 26410082.
8. Missios S, Bekelis K, Barnett GH. Renaissance of laser interstitial thermal ablation. Neurosurg Focus. 2015; 38:E13.


9. Sharma M, Balasubramanian S, Silva D, Barnett GH, Mohammadi AM. Laser interstitial thermal therapy in the management of brain metastasis and radiation necrosis after radiosurgery: an overview. Expert Rev Neurother. 2016; 16:223–232. PMID: 26731270.


10. Patel P, Patel NV, Danish SF. Intracranial MR-guided laser-induced thermal therapy: single-center experience with the Visualase thermal therapy system. J Neurosurg. 2016; 125:853–860. PMID: 26722845.


11. Carpentier A, McNichols RJ, Stafford RJ, Guichard JP, Reizine D, Delaloge S, et al. Laser thermal therapy: real-time MRI-guided and computer-controlled procedures for metastatic brain tumors. Lasers Surg Med. 2011; 43:943–950. PMID: 22109661.


12. Ivan ME, Mohammadi AM, De Deugd N, Reyes J, Rodriguez G, Shah A, et al. Laser ablation of newly diagnosed malignant gliomas: a meta-analysis. Neurosurgery. 2016; 79(Suppl 1):S17–S23. PMID: 27861322.
13. Lee I, Kalkanis S, Hadjipanayis CG. Stereotactic laser interstitial thermal therapy for recurrent high-grade gliomas. Neurosurgery. 2016; 79(Suppl 1):S24–S34. PMID: 27861323.


14. Louis DN, Ohgaki H, Wiestler OD, Cavenee WK. WHO Classification of Tumours of the Central Nervous System WHO/IARC Classification of Tumours. 4th rev. ed. Lyon: International Agency for Research on Cancer;2016.
15. Louis DN, Perry A, Reifenberger G, von Deimling A, Figarella-Branger D, Cavenee WK, et al. The 2016 World Health Organization Classification of Tumors of the Central Nervous System: a summary. Acta Neuropathol. 2016; 131:803–820. PMID: 27157931.


16. Dang L, White DW, Gross S, Bennett BD, Bittinger MA, Driggers EM, et al. Cancer-associated IDH1 mutations produce 2-hydroxyglutarate. Nature. 2009; 462:739–744. PMID: 19935646.


17. Zhao S, Lin Y, Xu W, Jiang W, Zha Z, Wang P, et al. Glioma-derived mutations in IDH1 dominantly inhibit IDH1 catalytic activity and induce HIF-1α. Science. 2009; 324:261–265. PMID: 19359588.
18. Dang L, Yen K, Attar EC. IDH mutations in cancer and progress toward development of targeted therapeutics. Ann Oncol. 2016; 27:599–608. PMID: 27005468.


19. Frezza C, Tennant DA, Gottlieb E. IDH1 mutations in gliomas: when an enzyme loses its grip. Cancer Cell. 2010; 17:7–9. PMID: 20129244.


20. Sanson M, Marie Y, Paris S, Idbaih A, Laffaire J, Ducray F, et al. Isocitrate dehydrogenase 1 codon 132 mutation is an important prognostic biomarker in gliomas. J Clin Oncol. 2009; 27:4150–4154. PMID: 19636000.


21. Sturm D, Witt H, Hovestadt V, Khuong-Quang DA, Jones DT, Konermann C, et al. Hotspot mutations in H3F3A and IDH1 define distinct epigenetic and biological subgroups of glioblastoma. Cancer Cell. 2012; 22:425–437. PMID: 23079654.
22. Ceccarelli M, Barthel FP, Malta TM, Sabedot TS, Salama SR, Murray BA, et al. Molecular profiling reveals biologically discrete subsets and pathways of progression in diffuse glioma. Cell. 2016; 164:550–563. PMID: 26824661.
23. Yan H, Parsons DW, Jin G, McLendon R, Rasheed BA, Yuan W, et al. IDH1 and IDH2 mutations in gliomas. N Engl J Med. 2009; 360:765–773. PMID: 19228619.
24. Chen H, Judkins J, Thomas C, Wu M, Khoury L, Benjamin CG, et al. Mutant IDH1 and seizures in patients with glioma. Neurology. 2017; 88:1805–1813. PMID: 28404805.


25. Kuhnt D, Becker A, Ganslandt O, Bauer M, Buchfelder M, Nimsky C. Correlation of the extent of tumor volume resection and patient survival in surgery of glioblastoma multiforme with high-field intraoperative MRI guidance. Neuro Oncol. 2011; 13:1339–1348. PMID: 21914639.


26. Napolitano M, Vaz G, Lawson TM, Docquier MA, van Maanen A, Duprez T, et al. Glioblastoma surgery with and without intraoperative MRI at 3.0T. Neurochirurgie. 2014; 60:143–150. PMID: 24975207.


27. Herrera SR, Shin JH, Chan M, Kouloumberis P, Goellner E, Slavin KV. Use of transparent plastic tubular retractor in surgery for deep brain lesions: a case series. Surg Technol Int. 2010; 19:47–50. PMID: 20437344.
28. Recinos PF, Raza SM, Jallo GI, Recinos VR. Use of a minimally invasive tubular retraction system for deep-seated tumors in pediatric patients. J Neurosurg Pediatr. 2011; 7:516–521. PMID: 21529192.


29. Bloch O, Crane CA, Fuks Y, Kaur R, Aghi MK, Berger MS, et al. Heat-shock protein peptide complex-96 vaccination for recurrent glioblastoma: a phase II, single-arm trial. Neuro Oncol. 2014; 16:274–279. PMID: 24335700.


30. Lawler SE, Speranza MC, Cho CF, Chiocca EA. Oncolytic viruses in cancer treatment: a review. JAMA Oncol. 2017; 3:841–849. PMID: 27441411.
31. Desjardins A, Sampson JH, Peters KB, Vlahovic G, Randazzo D, Threatt S, et al. Patient survival on the dose escalation phase of the Oncolytic Polio/Rhinovirus Recombinant (PVSRIPO) against WHO grade IV malignant glioma (MG) clinical trial compared to historical controls. J Clin Oncol. 2016; 34(suppl 15):2061. PMID: 27069080.


32. Chiocca EA, Yu J, Phuphanich S, Lukas RV, Kumthekar P, Yang Y, et al. Expanded phase I study of intratumoral Ad-RTS-hIL-12 plus oral veledimex: tolerability and survival in recurrent glioblastoma. J Clin Oncol. 2017; 35(suppl 15):2044. PMID: 28459607.


33. Ostertag D, Cloughesy T, Kalkanis S, Mikkelsen T, Landolfi J, Carter B, et al. ATIM-17. Investigation of anti-tumor cellular immune response elicited by Toca 511 & Toca FC therapy in a recurrent HGG phase 1 trial. Neuro Oncol. 2016; 18(suppl 6):vi21.
34. Huang TT, Hlavaty J, Ostertag D, Espinoza FL, Martin B, Petznek H, et al. Toca 511 gene transfer and 5-fluorocytosine in combination with temozolomide demonstrates synergistic therapeutic efficacy in a temozolomide-sensitive glioblastoma model. Cancer Gene Ther. 2013; 20:544–551. PMID: 23969884.


35. Ahmed AU, Thaci B, Tobias AL, Auffinger B, Zhang L, Cheng Y, et al. A preclinical evaluation of neural stem cell-based cell carrier for targeted antiglioma oncolytic virotherapy. J Natl Cancer Inst. 2013; 105:968–977. PMID: 23821758.


36. Ahmed AU, Ulasov IV, Mercer RW, Lesniak MS. Maintaining and loading neural stem cells for delivery of oncolytic adenovirus to brain tumors. Methods Mol Biol. 2012; 797:97–109. PMID: 21948472.


37. Ahmed AU, Thaci B, Alexiades NG, Han Y, Qian S, Liu F, et al. Neural stem cell-based cell carriers enhance therapeutic efficacy of an oncolytic adenovirus in an orthotopic mouse model of human glioblastoma. Mol Ther. 2011; 19:1714–1726. PMID: 21629227.


38. Cloughesy TF, Ostertag D, Landolfi JC, Walbert T, Vogelbaum MA, Elder JB, et al. Durable complete responses observed in IDH1 mutated high grade glioma at first recurrence undergoing treatment with Toca 511 and Toca FC. J Clin Oncol. 2017; 35(Suppl 15):e13504.


39. Hottinger AF, Pacheco P, Stupp R. Tumor treating fields: a novel treatment modality and its use in brain tumors. Neuro Oncol. 2016; 18:1338–1349. PMID: 27664860.


40. Stupp R, Idbaih A, Steinberg DM, Read W, Toms S, Barnett G, et al. LTBK-01: Prospective, multi-center phase III trial of tumor treating fields together with temozolomide compared to temozolomide alone in patients with newly diagnosed glioblastoma. Neuro Oncol. 2016; 18(suppl 6):i1. PMID: 26705298.


41. Stupp R, Taillibert S, Kanner AA, Kesari S, Steinberg DM, Toms SA, et al. Maintenance therapy with tumor-treating fields plus temozolomide vs temozolomide alone for glioblastoma: a randomized clinical trial. JAMA. 2015; 314:2535–2543. PMID: 26670971.
42. Reardon DA, Desjardins A, Schuster J, Tran DD, Fink KL, Nabors LB, et al. IMCT-08 ReACT: long-term survival from a randomized phase II study of rindopepimut (CDX-110) plus bevacizumab in relapsed glioblastoma. Neuro Oncol. 2015; 17:v109.
43. Weller M, Butowski M, Trand DD, Recht LD, Lim M, Hirte H, et al. Rindopepimut with temozolomide in patients with newly diagnosed, EGFRvIII-expressing glioblastoma (ACT IV): a randomised, double-blind, international phase 3 trial. Lancet Oncol. 2017; 18:1373–1385. PMID: 28844499.
44. Borghaei H, Paz-Ares L, Horn L, Spigel DR, Steins M, Ready NE, et al. Nivolumab versus docetaxel in advanced nonsquamous non-small-cell lung cancer. N Engl J Med. 2015; 373:1627–1639. PMID: 26412456.


45. Robert C, Schachter J, Long GV, Arance A, Grob JJ, Mortier L, et al. Pembrolizumab versus ipilimumab in advanced melanoma. N Engl J Med. 2015; 372:2521–2532. PMID: 25891173.


46. Powles T, Eder JP, Fine GD, Braiteh FS, Loriot Y, Cruz C, et al. MPDL3280A (anti-PD-L1) treatment leads to clinical activity in metastatic bladder cancer. Nature. 2014; 515:558–562. PMID: 25428503.


47. Omuro A, Vlahovic G, Baehring J, Butowski NA, Reardon DA, Cloughesy T, et al. ATIM-16. Nivolumab combined with radiotherapy with or without temozolomide in patients with newly diagnosed glioblastoma: results from phase 1 safety cohorts in CHECKMATE 143. Neuro Oncol. 2016; 18(suppl 6):vi21.


48. Reardon DA, Kim TM, Frenel JS, Santoro A, Lopez J, Subramaniam DS, et al. ATIM-35. Results of the phase IB KEYNOTE-028 multi-cohort trial of pembrolizumab monotherapy in patients with recurrent PD-L1-positive glioblastoma multiforme (GBM). Neuro Oncol. 2016; 18(suppl 6):vi25–vi26.


49. Sahebjam S, Johnstone PA, Forsyth P, Arrington J, Jaglal M, Tran ND, et al. ATIM-15. A phase I trial of hypofractionated stereotactic irradiation (HFSRT) with pembrolizumab and bevacizumab in patients with recurrent high grade gliomas. Neuro Oncol. 2016; 18(suppl 6):vi21.
50. Dixit K, Kumthekar P, Kruser T, Bloch O, Chandler J, Tate M, et al. ATIM-10. Phase I/II trial of radiation therapy, temozolomide and pembrolizumab followed by temozolomide and pembrolizumab in patients with newly diagnosed glioblastoma. Neuro Oncol. 2016; 18(suppl 6):vi19.


51. Reardon DA, Omuroa A, Brandes AA, Rieger J, Wick A, Sepulveda J, et al. OS10.3 Randomized phase 3 study evaluating the efficacy and safety of nivolumab vs bevacizumab in patients with recurrent glioblastoma: CheckMate 143. Neuro-Oncology. 2017; 19(suppl 3):iii21.


52. Reardon D, Kaley T, Dietrich J, Lim M, Dunn G, Gan H, et al. ATIM-04. Phase 2 study to evaluate the clinical efficacy and safety of MEDI4736 (durvalumab [DUR]) in patients with glioblastoma (GBM): results for cohort B (dur monotherapy), bevacizumab (bev) naïve patients with recurrent GBM. Neuro Oncol. 2016; 18(suppl 6):vi18.


53. Lukas RV, Rodon J, Shih K, Wong ET, Becker K, Fasso M, et al. Clinical activity and safety of atezolizumab (anti-PDL1) in patients with glioblastoma (GBM). In : 20th Ann Sci Meeting Edu Day Soc Neuro-Oncol; 2015 Nov 19-22; San Antonio, TX. Houston (TX): Society for Neuro-Oncology;2015.
54. Zakharia Y, Colman H, Mott F, Lukas R, Vahanian N, Link C, et al. IMCT-21 Updates on phase 1B/2 combination study of the IDO pathway ihibitor indoximod with temozolomide for adult patients with temozolomide-refractory primary malignant brain tumors. Neuro Oncol. 2015; 17:v112.
55. Butowski N, Colman H, De Groot JF, Omuro AM, Nayak L, Wen PY, et al. Orally administered colony stimulating factor 1 receptor inhibitor PLX3397 in recurrent glioblastoma: an Ivy Foundation Early Phase Clinical Trials Consortium phase II study. Neuro Oncol. 2016; 18:557–564. PMID: 26449250.


Fig. 1
Arrays for TTF applied directly to the shaved scalp. The patient consented to the use of this photograph. TTF: tumor treating fields.
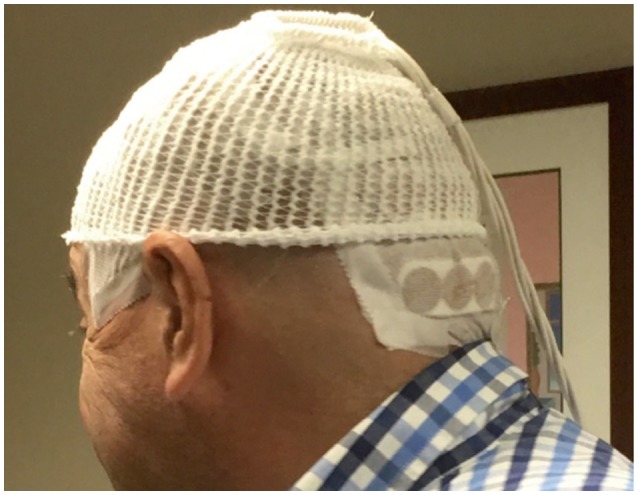
Table 1
Advances in the management of HGG
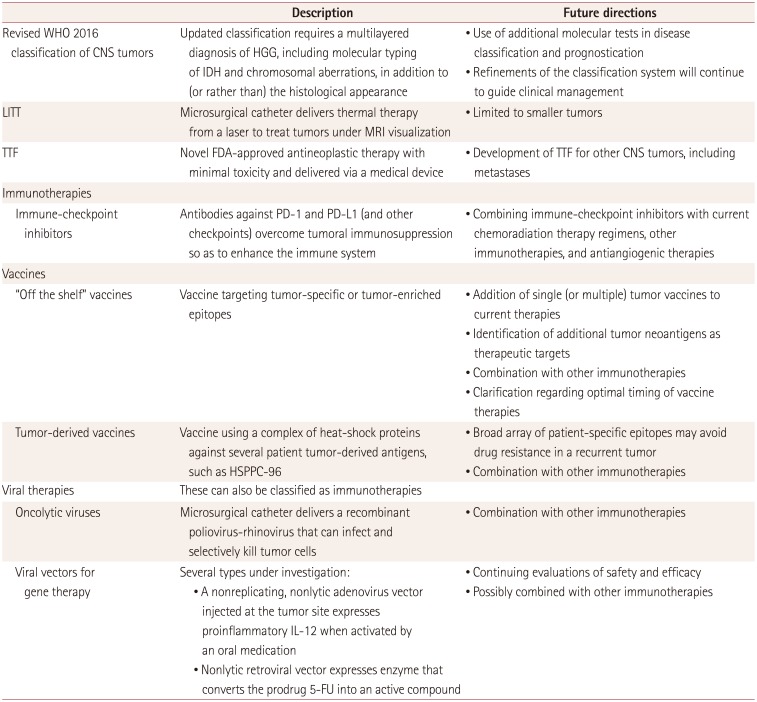