Abstract
The cerebellum plays vital roles in balance control and motor learning, including in saccadic adaptation and coordination. It consists of the vermis and two hemispheres and is anatomically separated into ten lobules that are designated as I–X. Although neuroimaging and clinical studies suggest that functions are compartmentalized within the cerebellum, the function of each cerebellar lobule is not fully understood. Electrophysiological and lesion studies in animals as well as neuroimaging and lesion studies in humans have revealed that vermian lobules VI and VII (declive, folium, and tuber) are critical for controlling postural balance, saccadic eye movements, and coordination. In addition, recent structural magnetic resonance imaging studies have revealed that these lobules are larger in elite basketball and short-track speed skaters. Furthermore, in female short-track speed skaters, the volume of this region is significantly correlated with static balance. This article reviews the function of vermian lobules VI and VII, focusing on the control of balance, eye movements, and coordination including coordination between the eyes and hands and bimanual coordination.
The cerebellum, located in the posterior cranial fossa, is a major motor structure of the brain. It controls motor-related functions, such as maintaining balance and posture, and motor learning including coordination of movements through complex regulatory and feedback mechanisms. Cerebellar lesions cause dysmetria, ataxia, and intention tremors.123 The cerebellum is also associated with the control of cognition and emotion, and patients with cerebellar dysfunction experience cognitive and affective symptoms.456
Mediolaterally, the cerebellum consists of the midline vermis and two cerebellar hemispheres. 7 In the anterior-posterior division, it can be divided into anterior, posterior, and flocculonodular lobes, which are further separated into ten lobules that are designated as I–X.8 The anterior lobe (comprising lobules I–V) is divided from the posterior lobe by the primary fissure, and the posterior lobe (comprising lobules VI–IX) is divided from the flocculonodular lobe (lobule X) by the posterolateral fissure.9
The medial zone consists of the vermis and nuclei fastigii (NF),7 which is primarily associated with the control of posture and locomotion. Lesions in the vermis in humans and inactivation of the NF in monkeys induce deficits in sitting, standing, and walking.101112 The nucleus interpositus (NI) and those portions of the paravermal cortex that project to these nuclei form the intermediate zone.7 Transitory inactivation of the NI and the adjacent portion of the dentate nucleus (DN) in monkeys results in tremor11 and deficits in reaching and grasping motions.131415 The lateral cerebellar zone consists of the cerebellar hemispheres and most of the DN.7 Its anterior portion participates in motor control, while the posterolateral portion is involved in motor planning, language production, and cognitive processes such as memory function.161718
Different regions of the cerebellar cortex form microcomplexes that process information delivered by the inputs of each microcomplex' equally.319 This means that different regions of the cerebellum are involved depending on the nature of the task, and they mediate different functions based on their inputs and outputs.32021 Although functions are compartmentalized within the cerebellum, the function of each cerebellar lobule is not well understood.
Structural anomalies and agenesis of the posterior vermis are related to cognitive deficiency and emotional symptoms.2223 The volume of vermian lobules VI and VII (also called the declive, folium, and tuber) is significantly correlated with the performance in cognitive testing.24 Vermian lobule VI is related to retrieval in the Sternberg working memory task,25 and vermian lobule VII is involved in the affective features of cerebellar cognitive affective syndrome and posterior fossa syndrome.2627 In addition, vermian lobules VI and VII have been found to be significantly smaller in patients with autism.28 These lobules might play roles in the regulation of cognition and emotion. There is evidence from recent neuroimaging and clinical studies that these cerebellar regions are critically associated with balance control and saccadic adaptation.
The function of the human cerebellum was traditionally elucidated using lesion studies. Cerebellar lesions induce specific behavioral impairments. Conversely, experience-dependent structural plasticity is helpful for providing insights into structure-function relationships in the human brain (Fig. 1). Longitudinal studies of juggling training demonstrated that motor learning induces structural changes in brain regions demanded by the task and suggest a causal relationship between motor learning and morphological plasticity.2930 Imaging studies have revealed structural alterations in the cerebellum of sportspeople after they have undergone intense training of complex motor skills (Table 1).313233343536373839 Such sports related structural changes in the cerebellum of sportspeople provide useful information for comprehending the specific function of each cerebellar lobule as well as neural mechanisms in motor experts. This review focuses on the function of vermian lobules VI and VII associated with balance control and eye movements, including in sportspeople.
Lesion studies of both animals and humans suggest that the cerebellum, especially the medial zone (vermis and NF), plays a vital role in balance control.1112404142434445 The medial zone has inputs from the primary vestibular afferents and vestibular nuclei, reticular nuclei, pontine nuclei, and the spinal cord.46474849 The medial zone projects mainly to vestibular and reticular nuclei through the NF.4950 Animal studies have shown that the medial zone is essential for maintaining balance and postural tone and for walking.1140414551 In contrast, lesions in the lateral cerebellum (including the DN) cause no balance anomalies, very mild gait defects, but severe defects in the control of voluntary limb movements.1152 The lateral zone has inputs mainly from cerebral cortices with the thickest projections from the primary motor, premotor, primary somatosensory, posterior parietal, prefrontal cortices, and temporal lobe regions.4953 The lateral zone projects to the red nucleus through the DN and to cerebral cortices including primary motor, premotor, parietal, and prefrontal cortices through the thalamus.49505455 Recent track tracing investigation in non-human primates found that the primary motor cortex and several cortical motor areas (the supplementary motor, dorsal cingulate motor, and the ventral cingulate motor areas) project to lobules V–VIII of the vermis.56 This suggests that these regions of the vermis are where the cortical motor areas affect the descending control systems associated with the control of whole-body posture.
A human study using magnetic resonance imaging (MRI) revealed that lesions containing the NF and neighboring NI are involved in balance-control deficits.424357 Lesions in the NF that also include the NI are correlated with posture ataxia and gait deficits.58 Furthermore, the volume of the medial and neighboring intermediate cerebellum is negatively correlated with posture and gait deficits.59 Previous animal studies showed that the NF is involved in responses to vestibular stimulation6061 and controls limb extensor muscle tone for maintaining posture.4162 This suggests that the cerebellar vermis and fastigial nuclei play an essential role in balance control.
MRI-based lesion symptom mapping has also revealed that lesions involving the NF, anterior vermal lobules I–III, and posterior vermal lobules VIII and IX are associated with disorders of balance control.424357 Lesions of the vermal and paravermal lobules II, III, and IV are correlated with posture and gait ataxia.58 Pathology of the anterior superior cerebellar vermis induces balance and gait deficits in detoxified alcoholics.636465 In addition, the sway path length and sway prominence in the 2–5 Hz band are associated with the volume of the anterior-superior vermis in alcoholics.66
It is thought that tasks requiring sophisticated control of balance, such as tandem stance and standing on a cushion, are associated with the posterior lobe of the cerebellar vermis, whereas pathology in the anterior lobe of the cerebellar vermis is involved in balance deficits. Parallel fibers cross the midline cerebellar cortex and attach to Purkinje cells on either side of the posterior vermis. Purkinje cells might be critical for bilateral coordination of the legs and trunk, which is an essential function in balance control.45 In addition, the posterior vermis gets sparse peripheral somatosensory inputs, vestibular inputs, and some corticopontine inputs from the visual areas, whereas the anterior vermis takes more somatosensory input and little vestibular input.6768 Because the posterior vermis takes principally vestibular information, it has been thought to control balance and gait.
Deficits in tandem stance with the eyes open and standing on a cushion with the eyes closed are associated with lesions in vermal lobules VII and VIII, including the NF and NI.44 Five children with posterior inferior vermal splits ranging from lobules VI to X showed deficits in tandem gait and only slight anomalies in self-paced gait and hopping on one leg.12 Meanwhile, lesions involving vermian lobules VII, IX, and X cause lateropulsion.69
It is plausible that maintaining postural balance during unipedal gliding on smooth ice involves a more precise coordinating response to postural modification for shifting the center of gravity as in tandem stance than normal bipedal standing.7071 Recent structural MRI studies involving elite short-track speed skaters, who need exceptional balance control, indicated that their vermian lobules VI and VII are larger than in control subjects,3334 and that the volume of this region is correlated with the static balancing ability of the left leg in female skaters.34 This suggests that vermian lobules VI and VII play an essential role in controlling postural balance and reflect that this brain region is essential to balance function.
Saccadic eye movements form an excellent motor learning model of the cerebellum because it is possible to slowly alter the amplitude of a saccade if the target moves constantly while the saccade is being performed.727374 Physiological and lesion researches involving non-human primates as well as imaging and lesion researches involving humans have revealed that the vermis especially vermian lobules VI and VII (declive, folium, and tuber) is a central area controlling saccadic adaptation. Alterations in the spike activities of Purkinje cells in vermian lobules VI and VII are correlated with the level of saccadic adaptation in primates.74 The saccadic area within vermian lobules VI and VII has been defined as the oculomotor vermis.7576
There is very strong evidence that vermian lobules VI and VII participate in the control of eye movements. The Purkinje cells of these lobules project to the caudal part of the NF, which in turn projects to the vestibular nuclei and saccade-related brainstem nuclei;77 indeed, this cerebellar region contains saccade-related neurons.78 Stimulation of this region of the vermis can evoke saccadic eye movements in both monkeys767980 and humans,81 whereas lesions in this region result in a permanent inability to adjust the amplitude of saccades.8283 Numerous studies performed over the past decade that have employed functional MRI (fMRI) and positron emission tomography have revealed the cerebellum to be associated with the control of eye movements. In humans, visually guided saccades activate vermian lobules VI and VII,848586 and saccadic adaptation is correlated with increased blood flow in these lobules.8788
Transiently impairment of the function of the posterior cerebellum (including vermian lobules VI and VII) using repetitive transcranial magnetic stimulation in healthy human subjects significantly weakened the ability to adjust the amplitude of saccades.89 Clinical studies have revealed that patients with focal cerebellar lesions or cerebellar degeneration are less able to adjust saccades.909192 In addition, lesions involving vermian lobules VII, IX, and X cause nystagmus.69
Motor learning probably underlies coordination between the eyes and hands and bimanual coordination.9394 Therefore, interactions between these two systems can be controlled exactly. An fMRI study demonstrated bimanual coordination-related activity within vermian lobule VI, suggesting its involvement in the executive function of bimanual coordination and the control of spatiotemporal complexity of coordination patterns.95 Coordination between eye and hand movements involves activation of vermian lobule VII.93 In addition, a three-dimensional MRI volumetric study proposed that greater volume of vermian lobules VI and VII in basketball players than in control subjects might be involved in the coordination between the eyes and hands and bimanual coordination, which are required for shooting and dribbling the ball.31
Several studies have revealed that learning acrobatic tasks that require substantial coordination of gross and fine motor skills not merely simple motor activity such as in locomotion or running drives synaptogenesis in the cerebellar cortex between the parallel fibers and dendritic spines of Purkinje cells.96979899100 In addition, increases in the astrocytic glial volume per Purkinje cell,101102 the volume of the molecular layer per Purkinje cell,96 and the dendritic spine density and length of Purkinje cells,103 as well as dendritic hypertrophy of stellate cells104 in the cerebellum have been observed after complex motor skill learning. Alterations in white matter involve the number and diameter of axons, myelin thickness, axon branching, axon trajectories, and myelination, and they effect the speed of impulse transmission.105 These might underlie the increased volume of vermian lobules VI and VII in sportspeople at the structural level (Fig. 2). Furthermore, this lobule might be closely associated with the acquisition of exceptional, specialized skills under broad activation of other motor movements.
Both human and animal studies indicate that vermian lobules VI and VII (declive, folium, and tuber) of the cerebellum might be associated with various features of balance control and motor learning, such as visually guided saccades and motor coordination, including coordination between the eyes and hands and bimanual coordination. Understanding the functional roles of vermian lobules VI and VII may lead to insights into the anatomical foundations and clinical signs. The findings of the present review may help understand neuroimaging findings in the context of cerebellar contribution in a wide range of motor functions.
Acknowledgements
This work was supported by the National Research Foundation of Korea Grant funded by the Korean Government (NRF-2011-354-G00076) to ISP, Korea University Grant to NJL, and Brain Korea 21 Plus project to IJR.
References
1. Dow RS, Moruzzi G. The Physiology and Pathology of the Cerebellum. Minneapolis, MN: University of Minnesota Press;1958.
3. Ito M. The modifiable neuronal network of the cerebellum. Jpn J Physiol. 1984; 34:781–792. PMID: 6099855.


4. Levisohn L, Cronin-Golomb A, Schmahmann JD. Neuropsychological consequences of cerebellar tumour resection in children: cerebellar cognitive affective syndrome in a paediatric population. Brain. 2000; 123:1041–1050. PMID: 10775548.
5. Riva D, Giorgi C. The cerebellum contributes to higher functions during development: evidence from a series of children surgically treated for posterior fossa tumours. Brain. 2000; 123:1051–1061. PMID: 10775549.


6. Schmahmann JD, Sherman JC. The cerebellar cognitive affective syndrome. Brain. 1998; 121:561–579. PMID: 9577385.


7. Jansen J, Brodal A. Experimental studies on the intrinsic fibers of the cerebellum. II. The cortico-nuclear projection. J Comp Neurol. 73:1940; 267–321.


8. Larsell O, Jansen J. The comparative anatomy and histology of the cerebellum: the human cerebellum, cerebellar connections, and cerebellar cortex. Minneapolis, MN: The University of Minnesota Press;1972.
10. Dichgans J, Mauritz KH. Patterns and mechanisms of postural instability in patients with cerebellar lesions. Adv Neurol. 1983; 39:633–643. PMID: 6660113.
11. Thach WT, Kane SA, Mink JW, Goodkin HP. Cerebellar output: multiple maps and modes of control in movement coordination. In : Llinás R, Sotelo C, editors. The Cerebellum Revisited. New York, NY: Springer-Verlag;1992. p. 283–300.
12. Bastian AJ, Mink JW, Kaufman BA, Thach WT. Posterior vermal split syndrome. Ann Neurol. 1998; 44:601–610. PMID: 9778258.


13. Growdon JH, Chambers WW, Liu CN. An experimental study of cerebellar dyskinesia in the rhesus monkey. Brain. 1967; 90:603–632. PMID: 4964525.


14. Mackel R. The role of the monkey sensory cortex in the recovery from cerebellar injury. Exp Brain Res. 1987; 66:638–652. PMID: 3609206.


15. Mason CR, Miller LE, Baker JF, Houk JC. Organization of reaching and grasping movements in the primate cerebellar nuclei as revealed by focal muscimol inactivations. J Neurophysiol. 1998; 79:537–554. PMID: 9463420.


16. Leiner HC, Leiner AL, Dow RS. Cognitive and language functions of the human cerebellum. Trends Neurosci. 1993; 16:444–447. PMID: 7507614.


17. Middleton FA, Strick PL. Cerebellar output: motor and cognitive channels. Trends Cogn Sci. 1998; 2:348–354. PMID: 21227231.


18. Dum RP, Li C, Strick PL. Motor and nonmotor domains in the monkey dentate. Ann N Y Acad Sci. 2002; 978:289–301. PMID: 12582061.


19. Ito M. Bases and implications of learning in the cerebellum--adaptive control and internal model mechanism. Prog Brain Res. 2005; 148:95–109. PMID: 15661184.
20. Schmahmann JD. From movement to thought: anatomic substrates of the cerebellar contribution to cognitive processing. Hum Brain Mapp. 1996; 4:174–198. PMID: 20408197.


21. Stoodley CJ, Schmahmann JD. Evidence for topographic organization in the cerebellum of motor control versus cognitive and affective processing. Cortex. 2010; 46:831–844. PMID: 20152963.


22. Chheda MG, Sherman JC, Schmahmann JD. Neurologic, psychiatric and cognitive manifestations in cerebellar agenesis. Neurology. 2002; 58:356.
23. Tavano A, Grasso R, Gagliardi C, Triulzi F, Bresolin N, Fabbro F, et al. Disorders of cognitive and affective development in cerebellar malformations. Brain. 2007; 130:2646–2660. PMID: 17872929.


24. MacLullich AM, Edmond CL, Ferguson KJ, Wardlaw JM, Starr JM, Seckl JR, et al. Size of the neocerebellar vermis is associated with cognition in healthy elderly men. Brain Cogn. 2004; 56:344–348. PMID: 15522773.


25. Chen SH, Desmond JE. Temporal dynamics of cerebro-cerebellar network recruitment during a cognitive task. Neuropsychologia. 2005; 43:1227–1237. PMID: 15949507.


26. Famularo G, Corsi FM, Minisola G, De Simone C, Nicotra GC. Cerebellar tumour presenting with pathological laughter and gelastic syncope. Eur J Neurol. 2007; 14:940–943. PMID: 17662020.


27. Richter S, Schoch B, Kaiser O, Groetschel H, Dimitrova A, Hein-Kropp C, et al. Behavioral and affective changes in children and adolescents with chronic cerebellar lesions. Neurosci Lett. 2005; 381:102–107. PMID: 15882798.


28. Courchesne E, Yeung-Courchesne R, Press GA, Hesselink JR, Jernigan TL. Hypoplasia of cerebellar vermal lobules VI and VII in autism. N Engl J Med. 1988; 318:1349–1354. PMID: 3367935.


29. Draganski B, Gaser C, Busch V, Schuierer G, Bogdahn U, May A. Neuroplasticity: changes in grey matter induced by training. Nature. 2004; 427:311–312. PMID: 14737157.
30. Scholz J, Klein MC, Behrens TE, Johansen-Berg H. Training induces changes in white-matter architecture. Nat Neurosci. 2009; 12:1370–1371. PMID: 19820707.


31. Park IS, Lee KJ, Han JW, Lee NJ, Lee WT, Park KA, et al. Experience-dependent plasticity of cerebellar vermis in basketball players. Cerebellum. 2009; 8:334–339. PMID: 19259755.


32. Park IS, Lee YN, Kwon S, Lee NJ, Rhyu IJ. White matter plasticity in the cerebellum of elite basketball athletes. Anat Cell Biol. 2015; 48:262–267. PMID: 26770877.


33. Park IS, Lee NJ, Kim TY, Park JH, Won YM, Jung YJ, et al. Volumetric analysis of cerebellum in short-track speed skating players. Cerebellum. 2012; 11:925–930. PMID: 22351379.


34. Park IS, Yoon JH, Kim N, Rhyu IJ. Regional cerebellar volume reflects static balance in elite female short-track speed skaters. Int J Sports Med. 2013; 34:465–470. PMID: 23143696.


35. Wei G, Luo J, Li Y. Brain structure in diving players on MR imaging studied with voxel-based morphometry. Prog Nat Sci. 2009; 19:1397–1402.


36. Hüfner K, Binetti C, Hamilton DA, Stephan T, Flanagin VL, Linn J, et al. Structural and functional plasticity of the hippocampal formation in professional dancers and slackliners. Hippocampus. 2011; 21:855–865. PMID: 20572197.


37. Di X, Zhu S, Jin H, Wang P, Ye Z, Zhou K, et al. Altered resting brain function and structure in professional badminton players. Brain Connect. 2012; 2:225–233. PMID: 22840241.


38. Di Paola M, Caltagirone C, Petrosini L. Prolonged rock climbing activity induces structural changes in cerebellum and parietal lobe. Hum Brain Mapp. 2013; 34:2707–2714. PMID: 22522914.


39. Hänggi J, Langer N, Lutz K, Birrer K, Mérillat S, Jäncke L. Structural brain correlates associated with professional handball playing. PLoS One. 2015; 10:e0124222. PMID: 25915906.


40. Botterell EH, Fulton JG. Functional localization in the cerebellum of primates II. Lesions of midline structures (vermis) and deep nuclei. J Comp Neurol. 1938; 69:47–62.


41. Chambers WW, Sprague JM. Functional localization in the cerebellum. I. Organization in longitudinal cortico-nuclear zones and their contribution to the control of posture, both extrapyramidal and pyramidal. J Comp Neurol. 1955; 103:105–129. PMID: 13263445.


42. Ilg W, Giese MA, Gizewski ER, Schoch B, Timmann D. The influence of focal cerebellar lesions on the control and adaptation of gait. Brain. 2008; 131:2913–2927. PMID: 18835866.


43. Konczak J, Schoch B, Dimitrova A, Gizewski E, Timmann D. Functional recovery of children and adolescents after cerebellar tumour resection. Brain. 2005; 128:1428–1441. PMID: 15659424.


44. Schoch B, Hogan A, Gizewski ER, Timmann D, Konczak J. Balance control in sitting and standing in children and young adults with benign cerebellar tumors. Cerebellum. 2010; 9:324–335. PMID: 20352395.


45. Thach WT, Goodkin HP, Keating JG. The cerebellum and the adaptive coordination of movement. Annu Rev Neurosci. 1992; 15:403–442. PMID: 1575449.


46. Kotchabhakdi N, Walberg F. Primary vestibular afferent projections to the cerebellum as demonstrated by retrograde axonal transport of horseradish peroxidase. Brain Res. 1978; 142:142–146. PMID: 75045.


47. Clendenin M, Ekerot CF, Oscarsson O, Rosén I. Functional organization of two spinocerebellar paths relayed through the lateral reticular nucleus in the cat. Brain Res. 1974; 69:140–143. PMID: 4817908.


48. Matsushita M, Okado N. Spinocerebellar projections to lobules I and II of the anterior lobe in the cat, as studied by retrograde transport of horseradish peroxidase. J Comp Neurol. 1981; 197:411–424. PMID: 6163798.


49. Morton SM, Bastian AJ. Cerebellar control of balance and locomotion. Neuroscientist. 2004; 10:247–259. PMID: 15155063.


50. Asanuma C, Thach WT, Jones EG. Brainstem and spinal projections of the deep cerebellar nuclei in the monkey, with observations on the brainstem projections of the dorsal column nuclei. Brain Res. 1983; 286:299–322. PMID: 6189563.


51. Sprague JM, Chambers WW. Regulation of posture in intact and decerebrate cat. I. Cerebellum, reticular formation, vestibular nuclei. J Neurophysiol. 1953; 16:451–463. PMID: 13097194.


52. Botterell EH, Fulton JF. Functional localization in the cerebellum of primates III. Lesions of hemispheres (neocerebellum). J Comp Neurol. 1938; 69:63–87.


53. Glickstein M. How are visual areas of the brain connected to motor areas for the sensory guidance of movement? Trends Neurosci. 2000; 23:613–617. PMID: 11137151.


54. Middleton FA, Strick PL. Cerebellar projections to the prefrontal cortex of the primate. J Neurosci. 2001; 21:700–712. PMID: 11160449.


55. Dum RP, Strick PL. An unfolded map of the cerebellar dentate nucleus and its projections to the cerebral cortex. J Neurophysiol. 2003; 89:634–639. PMID: 12522208.


56. Coffman KA, Dum RP, Strick PL. Cerebellar vermis is a target of projections from the motor areas in the cerebral cortex. Proc Natl Acad Sci U S A. 2011; 108:16068–16073. PMID: 21911381.


57. Schoch B, Konczak J, Dimitrova A, Gizewski ER, Wieland R, Timmann D. Impact of surgery and adjuvant therapy on balance function in children and adolescents with cerebellar tumors. Neuropediatrics. 2006; 37:350–358. PMID: 17357037.


58. Schoch B, Dimitrova A, Gizewski ER, Timmann D. Functional localization in the human cerebellum based on voxelwise statistical analysis: a study of 90 patients. Neuroimage. 2006; 30:36–51. PMID: 16253526.


59. Brandauer B, Hermsdörfer J, Beck A, Aurich V, Gizewski ER, Marquardt C, et al. Impairments of prehension kinematics and grasping forces in patients with cerebellar degeneration and the relationship to cerebellar atrophy. Clin Neurophysiol. 2008; 119:2528–2537. PMID: 18835217.


60. Siebold C, Kleine JF, Glonti L, Tchelidze T, Büttner U. Fastigial nucleus activity during different frequencies and orientations of vertical vestibular stimulation in the monkey. J Neurophysiol. 1999; 82:34–41. PMID: 10400932.


61. Thach WT. Discharge of cerebellar neurons related to two maintained postures and two prompt movements. I. Nuclear cell output. J Neurophysiol. 1970; 33:527–536. PMID: 4988214.


62. Imperato A, Nicoletti F, Diana M, Scapagnini U, Di Chiara G. Fastigial influences on postural tonus as studied by kainate lesions and by local infusion of GABAergic drugs in the rat. Brain Res. 1984; 295:51–63. PMID: 6370373.


63. Mauritz KH, Dichgans J, Hufschmidt A. Quantitative analysis of stance in late cortical cerebellar atrophy of the anterior lobe and other forms of cerebellar ataxia. Brain. 1979; 102:461–482. PMID: 315255.


64. Diener HC, Dichgans J, Bacher M, Guschlbauer B. Characteristic alterations of long-loop “reflexes” in patients with Friedreich's disease and late atrophy of the cerebellar anterior lobe. J Neurol Neurosurg Psychiatry. 1984; 47:679–685. PMID: 6747644.


65. Baloh RW, Yee RD, Honrubia V. Late cortical cerebellar atrophy. Clinical and oculographic features. Brain. 1986; 109:159–180. PMID: 3484651.
66. Sullivan EV, Rose J, Pfefferbaum A. Effect of vision, touch and stance on cerebellar vermian-related sway and tremor: a quantitative physiological and MRI study. Cereb Cortex. 2006; 16:1077–1086. PMID: 16221930.


67. Bloedel JR, Courville J. Cerebellar afferent systems. In : Brooks VB, editor. Handbook of Physiology, the nervous system. Bethesda: American Physiological Society;1981. p. 735–829.
68. Brodal A. Afferent cerebellar connections. In : Jansen J, Brodal A, editors. Aspects of cerebellar anatomy. Oslo: Johan Grundt Tanum Forlag;1954. p. 82–177.
69. Ye BS, Kim YD, Nam HS, Lee HS, Nam CM, Heo JH. Clinical manifestations of cerebellar infarction according to specific lobular involvement. Cerebellum. 2010; 9:571–579. PMID: 20711853.


70. Mitchell J. Postural reactions of the lower limb to centre-of-gravity displacements in one-foot stance. Physiotherapy. 1971; 57:562–570. PMID: 5145738.
71. Richardson JK, Ashton-Miller JA, Lee SG, Jacobs K. Moderate peripheral neuropathy impairs weight transfer and unipedal balance in the elderly. Arch Phys Med Rehabil. 1996; 77:1152–1156. PMID: 8931527.


72. Doya K. Complementary roles of basal ganglia and cerebellum in learning and motor control. Curr Opin Neurobiol. 2000; 10:732–739. PMID: 11240282.


73. Hopp JJ, Fuchs AF. The characteristics and neuronal substrate of saccadic eye movement plasticity. Prog Neurobiol. 2004; 72:27–53. PMID: 15019175.


74. Kojima Y, Soetedjo R, Fuchs AF. Changes in simple spike activity of some Purkinje cells in the oculomotor vermis during saccade adaptation are appropriate to participate in motor learning. J Neurosci. 2010; 30:3715–3727. PMID: 20220005.


75. Noda H, Fujikado T. Involvement of Purkinje cells in evoking saccadic eye movements by microstimulation of the posterior cerebellar vermis of monkeys. J Neurophysiol. 1987; 57:1247–1261. PMID: 3585467.


76. Noda H, Fujikado T. Topography of the oculomotor area of the cerebellar vermis in macaques as determined by microstimulation. J Neurophysiol. 1987; 58:359–378. PMID: 3655873.


77. Noda H, Sugita S, Ikeda Y. Afferent and efferent connections of the oculomotor region of the fastigial nucleus in the macaque monkey. J Comp Neurol. 1990; 302:330–348. PMID: 1705268.


78. Helmchen C, Büttner U. Saccade-related Purkinje cell activity in the oculomotor vermis during spontaneous eye movements in light and darkness. Exp Brain Res. 1995; 103:198–208. PMID: 7789427.


79. Ron S, Robinson DA. Eye movements evoked by cerebellar stimulation in the alert monkey. J Neurophysiol. 1973; 36:1004–1022. PMID: 4202613.


80. Fujikado T, Noda H. Saccadic eye movements evoked by microstimulation of lobule VII of the cerebellar vermis of macaque monkeys. J Physiol. 1987; 394:573–594. PMID: 3443975.


81. Mottolese C, Richard N, Harquel S, Szathmari A, Sirigu A, Desmurget M. Mapping motor representations in the human cerebellum. Brain. 2013; 136:330–342. PMID: 22945964.


82. Barash S, Melikyan A, Sivakov A, Zhang M, Glickstein M, Thier P. Saccadic dysmetria and adaptation after lesions of the cerebellar cortex. J Neurosci. 1999; 19:10931–10939. PMID: 10594074.


83. Takagi M, Zee DS, Tamargo RJ. Effects of lesions of the oculomotor vermis on eye movements in primate: saccades. J Neurophysiol. 1998; 80:1911–1931. PMID: 9772249.


84. Hayakawa Y, Nakajima T, Takagi M, Fukuhara N, Abe H. Human cerebellar activation in relation to saccadic eye movements: a functional magnetic resonance imaging study. Ophthalmologica. 2002; 216:399–405. PMID: 12566881.


85. Nitschke MF, Arp T, Stavrou G, Erdmann C, Heide W. The cerebellum in the cerebro-cerebellar network for the control of eye and hand movements--an fMRI study. Prog Brain Res. 2005; 148:151–164. PMID: 15661188.
86. Stephan T, Mascolo A, Yousry TA, Bense S, Brandt T, Dieterich M. Changes in cerebellar activation pattern during two successive sequences of saccades. Hum Brain Mapp. 2002; 16:63–70. PMID: 11954056.


87. Desmurget M, Pélisson D, Urquizar C, Prablanc C, Alexander GE, Grafton ST. Functional anatomy of saccadic adaptation in humans. Nat Neurosci. 1998; 1:524–528. PMID: 10196552.


88. Desmurget M, Pélisson D, Grethe JS, Alexander GE, Urquizar C, Prablanc C, et al. Functional adaptation of reactive saccades in humans: a PET study. Exp Brain Res. 2000; 132:243–259. PMID: 10853949.


89. Jenkinson N, Miall RC. Disruption of saccadic adaptation with repetitive transcranial magnetic stimulation of the posterior cerebellum in humans. Cerebellum. 2010; 9:548–555. PMID: 20665254.


90. Golla H, Tziridis K, Haarmeier T, Catz N, Barash S, Thier P. Reduced saccadic resilience and impaired saccadic adaptation due to cerebellar disease. Eur J Neurosci. 2008; 27:132–144. PMID: 18184318.


91. Straube A, Deubel H, Ditterich J, Eggert T. Cerebellar lesions impair rapid saccade amplitude adaptation. Neurology. 2001; 57:2105–2108. PMID: 11739834.


92. Choi KD, Kim HJ, Cho BM, Kim JS. Saccadic adaptation in lateral medullary and cerebellar infarction. Exp Brain Res. 2008; 188:475–482. PMID: 18421449.


93. Miall RC, Jenkinson EW. Functional imaging of changes in cerebellar activity related to learning during a novel eye-hand tracking task. Exp Brain Res. 2005; 166:170–183. PMID: 16082535.


94. Debaere F, Wenderoth N, Sunaert S, Van Hecke P, Swinnen SP. Changes in brain activation during the acquisition of a new bimanual coodination task. Neuropsychologia. 2004; 42:855–867. PMID: 14998701.
95. Debaere F, Wenderoth N, Sunaert S, Van Hecke P, Swinnen SP. Cerebellar and premotor function in bimanual coordination: parametric neural responses to spatiotemporal complexity and cycling frequency. Neuroimage. 2004; 21:1416–1427. PMID: 15050567.


96. Black JE, Isaacs KR, Anderson BJ, Alcantara AA, Greenough WT. Learning causes synaptogenesis, whereas motor activity causes angiogenesis, in cerebellar cortex of adult rats. Proc Natl Acad Sci U S A. 1990; 87:5568–5572. PMID: 1695380.


97. Anderson BJ, Alcantara AA, Greenough WT. Motor-skill learning: changes in synaptic organization of the rat cerebellar cortex. Neurobiol Learn Mem. 1996; 66:221–229. PMID: 8946414.


98. Kleim JA, Swain RA, Armstrong KA, Napper RM, Jones TA, Greenough WT. Selective synaptic plasticity within the cerebellar cortex following complex motor skill learning. Neurobiol Learn Mem. 1998; 69:274–289. PMID: 9707490.


99. Federmeier KD, Kleim JA, Greenough WT. Learning-induced multiple synapse formation in rat cerebellar cortex. Neurosci Lett. 2002; 332:180–184. PMID: 12399010.


100. Kim HT, Kim IH, Lee KJ, Lee JR, Park SK, Chun YH, et al. Specific plasticity of parallel fiber/Purkinje cell spine synapses by motor skill learning. Neuroreport. 2002; 13:1607–1610. PMID: 12352611.


101. Anderson BJ, Li X, Alcantara AA, Isaacs KR, Black JE, Greenough WT. Glial hypertrophy is associated with synaptogenesis following motor-skill learning, but not with angiogenesis following exercise. Glia. 1994; 11:73–80. PMID: 7520887.


102. Kleim JA, Markham JA, Vij K, Freese JL, Ballard DH, Greenough WT. Motor learning induces astrocytic hypertrophy in the cerebellar cortex. Behav Brain Res. 2007; 178:244–249. PMID: 17257689.


103. Lee KJ, Jung JG, Arii T, Imoto K, Rhyu IJ. Morphological changes in dendritic spines of Purkinje cells associated with motor learning. Neurobiol Learn Mem. 2007; 88:445–450. PMID: 17720555.


104. Kleim JA, Swain RA, Czerlanis CM, Kelly JL, Pipitone MA, Greenough WT. Learning-dependent dendritic hypertrophy of cerebellar stellate cells: plasticity of local circuit neurons. Neurobiol Learn Mem. 1997; 67:29–33. PMID: 9013498.


105. Zatorre RJ, Fields RD, Johansen-Berg H. Plasticity in gray and white: neuroimaging changes in brain structure during learning. Nat Neurosci. 2012; 15:528–536. PMID: 22426254.


Fig. 2
Demonstrative image of a three-dimensional model of the cerebellum. The structural plasticity of vermian lobules VI and VII in sportspeople might be reflected by morphological changes detectable at microscopic level. Yellow: cerebellar hemisphere, orange: vermian lobules I-V (lingual, centralis, and culmen), red: vermian lobules VI and VII (declive, folium, and tuber), violet: vermian lobules VIII-X (pyramis, uvula, and nodulus). Park et al. Cerebellum,2009;8:334-339, with permission of Springer.31
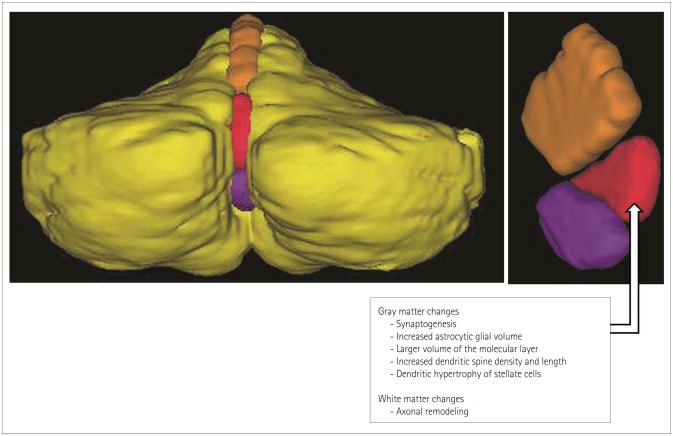
Table 1
Characteristics of the cerebellum resulting from structural plasticity in sportspeople

Study (year) | Sport | Characteristics of the cerebellum |
---|---|---|
Park et al. (2009)31 | Basketball players | ↑ Vermian lobules VI and VII |
Park et al. (2015)32 | Basketball players | ↑ WM of vermian lobules VI and VII |
Park et al. (2012)33 | Short-track speed skaters | ↑ Vermian lobules VI and VII and right hemisphere |
Park et al. (2013)34 | Short-track speed skaters | ↑ Vermian lobules VI and VII |
Wei et al. (2009)35 | Divers | ↓ GM of right hemisphere |
Hüfner et al. (2011)36 | Dancers and slackliners | ↑ GM of right hemisphere |
Di et al. (2012)37 | Badminton players | ↑ GM of right hemisphere |
Di Paola et al. (2013)38 | Rock climbers | ↑ Vermian lobules I–V |
Hänggi et al. (2015)39 | Handball players | ↓ WM of left and right hemisphere |