Abstract
Purpose
Radiation proctitis (RP) is inflammation and damage to the rectum, manifested secondary to ionizing radiation utilized for treatment. In this study, we evaluated the anti-inflammatory therapeutical and protective effects of ruscogenin in a model of acute RP.
Methods
Thirty-two Sprague-Dawley rats were divided into 4 groups (n = 8) as sham, control, treatment, and prophylaxis groups. Prophylaxis group and treatment group were dosed ruscogenin by oral gavage for 14 days pre- and postradiation. At the end of the 28th day, all subjects were sacrificed.
Results
Histopathological analysis showed a significant increase in cryptitis abscess, cryptitis and reactive atypia, and depth of lymphocytic infiltration of the control group, compared to the other groups (P < 0.05), while treatment and prophylaxis groups showed significant decreases (P < 0.05). Immunohistochemical analysis indicated that immunoreactivity were significantly higher in control group (P < 0.05, P < 0.001, and P < 0.01, respectively), but vice versa for treatment and prophylaxis groups. There was not any significant difference for fibroblast growth factor 2 immunoreactivity. The epithelium of control rectums indicated an increase in TNF-α immunoreactivity while other groups had significant decrease (P < 0.01). Electron microscopical findings were parallel to light microscopy.
Conclusion
In this study, ruscogenin was observed to be effective on prophylaxis or treatment of acute RP. Although there are various reports on the treatment of the rectum damaged by acute RP in the literature, this could be the first study since there is no research indicating the ultrastructural effect of ruscogenin.
Radiation of the pelvic region has been generally applied for the treatment of rectal cancer, gynecological malignancies, prostate cancer, and lymphoma. Now, more than 200,000 patients in the United States have received radiation therapy for abdominal, pelvic, and retroperitoneal tumors. Radiation proctitis (RP) is inflammation and damage to the rectum, manifesting secondary to ionizing radiation utilized for treatment [1]. More than 75% of the patients receiving radiation therapy have symptoms of acute radiation proctitis (ARP) and only 20% have symptoms related to chronic radiation proctitis (CRP) [234].
The pathogenesis of RP has not been totally clarified as of yet; however, it is known that firstly, mucosal damage is observed after radiation, subsequently, connective tissue is expanded and remodeled, and lastly, fibrosis and ischemia are observed [5]. Types of pathological damage vary in acute and chronic proctitis. Cryptitis abscess and cryptitis are experienced in ARP. Also, T lymphocytes, macrophages, and neutrophils are found [56].
There are many methods of treatment and protection against RP. However, none of them are a gold standard. Thus, RP needs to be investigated in terms of protection and treatment [7].
Ruscogenin, first isolated from Ruscus aculeatus, also a major steroidal sapogenin of the traditional Chinese herb Radix Ophiopogon japonicus, has been found to exert significant anti-inflammatory and antithrombotic activities [89]. Due to antielastase activity and capillary permeability, it has been used for treatment of vasculitis and chronic venous insufficiency more than 40 years in Europe [1011].
In this study, our purpose was to investigate the prophylactic and therapeutic effects of ruscogenin, which proved to have antithrombotic and anti-inflammatory activites, in a model of ARP by histopathological, immunohistochemical, and transmission electron microscopical analysis.
Animal Care and Use Committee of The Bagcilar Training and Research Hospital of Health Science University approved all experimental procedures in this study (protocol number: 2016-10; approval date: 3th March 2016).
Thirty-two female Sprague-Dawley rats (250 ± 30 g, with ages 4–8 weeks), supplied by the Bagcilar Training and Research Hospital Animal Center, Istanbul, Turkey, were maintained under laboratory conditions, housed in a controlled room with 12-hour light/dark cycles at 22℃, and fed with standard pellet chow including 21% protein and daily fresh water. All subjects were kept in separate cages each including 8 rats. All rats were divided into 4 random groups (n = 8) as; group 1, sham group, received equal amount of isotonic solution; group 2, control (radiation) group, received radiation (RT) + equal amounts of isotonic solution; group 3, prophylaxis group, received 3 mg/kg/day ruscogenin for 14 days and then RT; group 4. treatment group, received first RT, then 3 mg/kg/day ruscogenin for 14 days [9].
Under ketamine anesthesia by 5-mg/kg xylazine (Rompun, Bayer, Leverkusen, Germany) and 30-mg/kg ketamine (Ketalar, Pfizer, Istanbul, Turkey), rats of groups 2–4 were fixed on an acrylic plate in the supine position by strapping the tails and legs (Fig. 1). All parts of the body and extremities were excluded from the radiation area except the lower pelvic region in 3- × 4-cm dimensions including the 2-cm-long rectum. The dimensions of radiation area (Elekta, Model Precise Treatment System, 6-mev electron energy, 0.5-bolus material) were 20 × 20 cm2 and a single fraction of 2,500 cGy was given [12].
A single 3-mg/kg/day dose of ruscogenin (Chengdu Biopurify Phytochemicals Ltd., Chengdu, Sichuan, China) was injected daily by oral gavage to group 3 for 2 weeks before radiation; and to group 4 for 14 days after radiation. Group 1 was injected daily with a single dose of isotonic solution at the same volume by oral gavage for 14 days without performing radiation. Group 2 was injected daily with a single dose of isotonic solution at the same volume by oral gavage for 2 weeks after radiation. None of the rats died during the experiment.
Laparotomy was performed on every subject at the second week after radiotherapy under ketamine anesthesia (10-mg/kg xyilazine and 60-mg/kg ketamine) (Fig. 1); the rats were sacrificied by intracardiact puncture.
Rectums were dissected and washed with isotonic solution for histopathological and immunohistochemical evaluations. Under observation of a pathologist, the tissues were cut into 3 equal horizontal pieces and put into relevant fixatives [12].
All relevant pieces of rectal segments at 1 cm3 were fixed by 4% neutral formalin and embedded into the paraffin blocks. Five-micrometer sections from every paraffin block were stained by hematoxylin and eosin. Slides were evaluated by the same blinded pathologist twice under a light microscope (Nikon Eclipse E600, Melville, NY, USA).
Lamina propria (inflammation), invasion of leucocytes through the cryptic epithelium (cryptitis), deterioration of crypts (cryptic distortion), regenerative/restorative atypia of epithelial cells (reactive atypia), and inflammatory infiltration were investigated. Lesion severity was evaluated as 1, mild lesion; 2, moderate lesion; and 3, severe lesion; and 0 was accepted as none [12].
Cryptitis abscess was noted as positive or negative for each slide. Infiltration depth was measured by an image analysis system of a video attached microscope (Zeiss Axioplan 2, Scientific Imaging Corp., Campbell, CA, USA).
All relevant pieces of rectal segments were fixed by 4% neutral formalin, routine procedures were applied for tissue preparation and embedded into the paraffin blocks. Sections were stained immunohistochemically by using Streptavidin-Biotin-Peroxidase method with monoclonal and polyclonal antibodies tagged to indicate cell and tissue antigens, according to a previously described method [13]. Primary antibodies against inflammatory markers for TNF-α (Clontech Laboratories Inc., Mountain View, CA, USA), hypoxia-inducible factor 1α (HIF-1α: Novus Bio Inc., Littleton, CO, USA), IL-1β (Santa Cruz Biotechnology Inc., Santa Cruz, CA, USA), FGF-2 (Santa Cruz Biotechnology Inc.), and angiogenesis marker of vascular endothelial growth factor (VEGF: RayBiotech Inc., Norcross, GA, USA) were used. All antibodies were diluted as 1:100 with fresh phosphate buffer saline. Positively stained regions with relevant antigens were analyzed semiquantitatively in terms of staining intensity (modified H-SCORE analysis) given values between 0–300 and percentages for 5 different regions of each slide [13]. Thereby, rectal localization of these proteins and variance of the staining intensity and regional differences were determined.
Rectal segments at 1 mm3 were fixed by 2.5% glutaraldehyde and prepared for ultrastructural analysis as described previously [13]. Sections were evaluated under a Jeol Jem 1011 transmission electron microscope. The images were transferred to the Soft Imaging System Analysis programme by Megaview III digital camera, and pictures were taken. Each group was analyzed by 2 researchers and observational positive results were given as histopathogical changes in ultrastructures of the glands, glandular epithelium, surface epithelium and its thickness and nuclei, intercellular spaces, Goblet cells, mucus secretion, microvilli, tight junctions, and the basal membrane.
Semiquantitative analysis of cytokines immunohistochemically marked in tissue of all groups was performed by modified H-SCORE, given values between 0–300 for five different regions of each slide [13]. Statistical analysis was performed by GraphPadInstat ver. 3.06 (Graphpad Software Inc., La Jolla, CA, USA). Mean values of continuous variables are compared by one-way analysis of variance and variations between groups were compared by Tukey-Kramer Multiple Comparison Test. Categorical variables were evaluated by chi-square test. P < 0.05, P < 0.01, and P < 0.001 were accepted as statistically significant for different values of the groups.
Light micrographs with H&E staining are given in Fig. 2. As a result of light microscopical evaluations, it was observed that normal structure of the mucosa and submucosa, cryptic glands and other epithelial components of the sham group were not preserved in the control group, as expected. The control group drew attention by damaged mucosal structure, a decrease in the amount of cryptic glands, high levels of epithelial degenerations. Moreover, the thickness of mucosa increased locally due to cryptitis and cryptic apsis, compared to the sham group; leucocyte infiltration and fibrosis were also observed in the submucosa of the control group. On the other hand, the prophylaxis group had similar images of normal mucosal and submucosal components as in the sham group, except for the lesion severity and cryptic distortion. The treatment group showed a decrease in cryptic distortion, as well as in other histopathological parameters except for lesion severity (Fig. 2).
Inflammation, cryptitis, cryptic distortion in lamina propria, reactive atypia of epithelial cells, and inflammatory infiltration were evaluated in H&E stained tissues and lesion severity were graded as 0–3 points. Lesion severity of sham group was found as 0 (no lesion). In control group, its mean was estimated as 2.375 (moderate-severe lesion); 2 (moderate) for treatment group and 1.75 (mild-moderate) for prophylaxis group; it was observed to decrease compared to the control group. However, the difference between the control group and prophylaxis and treatment groups was not statistically significant (Table 1).
There were statistically significant increases in cryptic apsis, cryptitis, and reactive atypia in the control group, compared to the sham group (P < 0.05) while there wree significant decreases in the treatment and prophylaxis groups (P < 0.05). Cryptic distortion showed a significant decrease in the treatment group, compared to the control group (P < 0.05) while there was no significant change in the prophylaxis group (Table 1).
A significant increase was detected for infiltration depth in mucosa of the control group measured by light microscopy, compared to the other groups. It was due to massive lymphocyte infiltration (P < 0.001). There was no significant difference between the sham, treatment, and prophylaxis groups (Table 1), thus, ruscogenin treatment ameliorated the effect of acute proctitis.
H-SCORE analysis of immunoreactivities for inflammation markers of TNF-α, HIF-1α, IL-1β, FGF-2 and angiogenesis marker of VEGF are given in Table 2. Light micrographs of the tissues stained with relevant markers are shown in Fig. 3. Immunoreactivities on rectal tissues were evaluated for three regions: epithelium, crypts, and stroma.
Immunoreactivity of the first cytokine, IL-1β, showed a significant increase in epithelium and stroma of the control group, with regard to other groups (P < 0.05) and treatment and prophylaxis groups indicated significant decreases (Table 2), compared to the control group. But, these differences were not detected for FGF-2 cytokine in the crypts or stroma while the epithelium showed increased FGF-2 immunoreactivity in the control group (Table 2). The prohylaxis and treatment groups indicated significant decreased stainings for FGF-2 cytokine in the epithelium, compared to the control group, but not in other parts of the rectum. Light micrographs also proved these results in Fig. 3.
Immunoreactivity of the other cytokine TNF-α increased significantly only in the epithelium of the control group, compared to the other groups (P < 0.01), and decreased significantly in the treatment and prophylaxis groups (Table 2). Stroma and crypts had no such significant differences of staining (Table 2).
There was a significant increase in immunoreactivity of HIF-1α in epithelium, crypts, and stromal regions of the control group, comparing the other groups (P < 0.001 for epithelium, P < 0.01 for crypts and P < 0.05 for stroma), and significant decreases in the treatment and prophylaxis groups (Table 2). These decreases were also observed in the light micrographs (Fig. 3).
Comparing the number of vessels stained for angiogenesis marker, VEGF in the stroma of the mucosa and submucosa, the control group showed significant increase (P < 0.01) and other groups had significant decreases (Table 2). In other words, the sham, treatment, and prophylaxis groups had no significant differences for VEGF immunoreactivity (Table 2). These findings were also observed in light micrographs (Fig. 3).
Electron microscopical results were parallel to the light microscopy as there were histopathogical changes in the control group related to the acute radiation (Fig. 4). With atrophy and distortion on the glands in the control group, the glandular epithelium was diminished and corroded (Fig. 4B), compared to the sham group (Fig. 4A); migrated nuclei of the epithelium to the lumen side and dilatation of intercellular spaces were observed. The number of goblet cells decreased, mucus secretion diminished, epithelial cells shrank, and there were a few damaged cryptic stem cells. Decreased numbers of microvilli on the epithelium and diminished length, as well as apical vacuolization, were remarkable on the epithelium. On some regions, microvilli were totally deleted, nuclei of the epithelium were enlarged, chromatin modelling differed, and even some gained apoptotic appearance (Fig. 4B).
In the prophylaxis and treatment groups, the epithelium of the rectum was at normal thickness, number and length, as in the sham group; regular crypts were also observed at normal depth (Fig. 4C, D). However, the treatment group had some apoptotic epithelial cells locally. Intercellular spaces were observed at normal distance but with local mild dilatations in both groups. The treatment group had more of these dilatations than the other groups (Fig. 4D2). Number and height of microvilli projecting to the lumen was preserved with their normal structure in these groups (Fig. 4C1, D1), as in the sham group (Fig. 4A1). Many tight junctions protected the integrity of the epithelium. Unlike the control group, prophylaxis group preserved the continuity and homogenicity of the basal membrane and had normal digitations in the intercellular spaces; however, the treatment group lost continuity and homogenicity and had abnormal digitations (Fig. 4D2).
It is crucial to prevent RP, having many difficulties in the therapy thereof. Decreasing the radiation dose or excluding the rectum from the radiation area is advised. Some agents can be used such as rectal or oral misoprostol, rectal sucralfate, and intravenous amifostine [14]. However, there have been no proven methods in the literature as of yet showing preventative strategies to be effective.
A part of the ARP can be ameliorated by supportive therapies [14]. The group with difficulties in treatment was with CRP, so it would be an effective approach to prevent this pathological situation as it has considerable drawbacks.
ARP is an inducible inflammation. Macroscopical, histopathological, and immunohistochemical analyses have been performed to determine this inflammation [1516171819202122]. In this study, to show the inflammation, markers of IL-1β, TNF-α, and HIF1α cytokines were investigated; to show fibrosis in the rectal tissue, FGF2 was used as a marker and VEGF marker was used to show angiogenesis.
The cytokine IL-1, initiator of prostaglandin synthesis in polymorphonuclear leucocytes and connective tissue cells, has coeffects with TNF. In addition, it activates the vascular endothelial cells [19]. TNF has an effect of oxidative damage on endothelial cells and increases the number of adhesion molecules; it also induces angiogenesis and arranges hematopoiesis [20]. Moreover, angiogenesis and endothelial cell proliferation can also be induced by FGF or VEGF [2123].
HIF-1 detects changes in oxygen concentrations at the cell level and as a response, induces formation of new vessels and organizes many metabolisms such as anaerobic glycolysis [22].
In our study, immunoreactivities of IL-1β, TNF-α, HIF-1α, and VEGF increased in the control group of ARP, without the treatment, compared to the other groups; on the contrary, ruscogenin application caused significant decreases; thus showing that ruscogenin has anti-inflammatory effects on ARP.
Our histopathological evaluations indicated significant increases in cryptic apsis, cryptitis, and reactive atypia of the control (proctitis) group. Cryptic distortion of the control group had a significant difference from the treatment group, but not from the prophylaxis group. Infiltration depth of the same group significantly increased, compared to the other groups. Generally considering these light microscopic evaluations, histopathological findings of the prophylaxis group was more significant than the treatment group. In other words, the protective effect of ruscogenin surpasses its therapeutical effect histopathologically.
Many agents like ruscogenin that can be effective on the damage of acute radiation have been investigated in several studies. In a study by Ito et al. [24] investigating the effect of C vitamin against lethal intestinal damage induced by radiation, pre- and postradiation application of vitamin C caused a decrease in cytokine levels (TNF and IL-6) in the intestine of rats. Our immunohistochemical analysis demonstrated that ruscogenin application gave rise to a decrease in elevated IL-1 and TNF levels, compared to increased levels in the proctitis group.
A flow cytometry study by Huang et al. [9] has revealed that ruscogenin decreases intercellular adhesion molecule 1 expression in endothelial cells by inhibiting nuclear factor-kappa B pathway (NF-κB), and has an anti-inflammatory effect parallel to the dexamethazole. Also, in a report by Lu et al. [25] using diabetic rats, anti-inflammatory effects of ruscogenin have been presented through NF-κB pathway of the renal activities.
In a report by Haboubi et al. [26], rectal biopsies of 6 patients with acute proctitis depending on the symptomatic radiation were evaluated by electron microscopy; epithelial changes, fibrosis, variations in the lamina propria, and cryptic distortion were detected ultrastructurally. These findings are parallel to our electron microscopic results in the experimental rat model of ARP.
Hovdenak et al. [27] evaluated rectal biopsies before pelvic radiation and at the 14th day and the 6th week after radiation histopathologically and endoscopically revealing the time interval could be original in terms of therapeutical and prophylactic effects. Moreover, their biopsy findings at the second week were similar to our data of cryptic distortion, cryptic apsis, epithelial changes, stromal infiltration, and the time intervals.
In an experimental ARP model by Sezer et al. [12], histopathological data was evaluated and hesperidine and diosmin were proven to be effective. Also, Doi et al. [16] investigated the effects of polaprezinc in an experimental report and it was found histopathologically and endoscopically effective.
In parallel to the aforementioned light microscopic findings, our electron microscopic analysis showed histopathological changes in the control group due to the acute radiation. Ultrastructurally, both treatment and prophylaxis groups demonstrated improvements in the mucosa and submucosa and most of the damage by ARP was cured. To sum up, electron microscopic analysis of the prophylaxis and treatment groups indicated the therapeutical and protective effects of ruscogenin on ARP. In the literature, there have been reports on the ultrastructure of the rectum damaged by ARP but the ultrastructural effect of ruscogenin on proctitis has not been reported yet.
As a result, this study is the first in terms of histopathological, anti-inflammatory, and ultrastructural effects of ruscogenin on the rectum of ARP models. Yet, many clinical trials and especially biochemical studies are needed to support our data. Our study may be a pilot research in this respect.
Figures and Tables
Fig. 1
Application of radiation (A, B) and dissection of rectums (C, D) after the sacrification of rats.
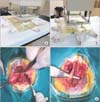
Fig. 2
Rectum samples from four experimental groups (sham, control, prophylaxis, and treatment) (H&E, ×100). (A) Sham group, (B) control (radiation) group, (C) prophylaxis group, and (D) treatment group. E, surface epithelium; C, cryptic glands; S, stroma with lamina propria; Su, submucosa; L, lumen of rectum.
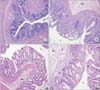
Fig. 3
Immunoreactivities for FGF-2 (A–D), HIF-1α (E–H) cytokines and vascular endothelial growth factor marker (I-L) in 4 experimental groups: (A–H, ×200; I–L, ×100) (A, E, I) sham group, (B, F, J) control (radiation) group, (C, G, K) treatment group, and (D, H, L) prophylaxis group. E, surface epithelium; C, cryptic glands; S, stroma with lamina propria; Su, submucosa; L, lumen of rectum; V, vessels.
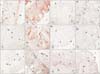
Fig. 4
Electron microscopical analysis of rectum sections from experimental rat model of radiation proctitis. (A1) On rectum surface epithelium of the sham group, microvilli (white arrow) at regular number and typical ultrastructure and tight junctions with regular ultrastructure (red arrow) were observed (×12,000). (A2) All parts of cryptic epithelium (N) of the sham group was shown to protect of their basement membrane (blue arrow) with homogenous and continuous ultrastructure, while intercellular area of the epithelium had epithelial digitations (asterisk) in accordance with normal rectum physiology (×10,000). (B1) Of the cryptic epithelial cells (E) in the control group, chromatin models of the nuclei (N) were noticed to alter, some of them showed diffuse heterochromatic appearance. Moreover, apical migration was remarkable in nuclei. Reduced number of shortened microvilli (white arrow) and intercellular digitations (asterisk) were observed both in cryptic and surface epithelia (×7,500). (B2) At basal level of surface epithelium (E) of the control group, the number of vacuoles (V) increased; number of microvilli (white arrow) decreased with highly shortened forms; the continuity and homogeneity of basement membrane (blue arrow) was lost and locally nude (green arrow). Fibrin augmentation (Fi) in the lamina propria (Lp) due to fibrosis was remarkable (×7,500). (C1) On the rectum surface epithelium of the prophylaxis group, microvilli (white arrow) projecting through lumen (L) at typical ultrastructure acquired their normal number and height. A large number of tight junctions (red arrows) also protected integrity of the epithelium (E) (×10,000). (C2) In prophylaxis group, the basement membrane (blue arrows) protected its continuity and homogeneity and the intercellular area (asterisk) had normal digitations. The Lp was remarkable to have a little increase in the extracellular matrix (×10,000). (D1) On the rectum surface epithelium of the treatment group, microvilli (white arrow) projecting through L at typical ultrastructure acquired their normal number and height. Intercellular borders (asterisk) protected their distance and a large number of tight junctions (red arrows) protected the integrity of the epithelium (E) (×10,000). (D2) The basement membrane (blue arrows) of the cryptic epithelium lost its continuity and homogeneity; abnormal digitations were observed in the intercellular spaces (red asterisk). Ultrastructure of mithochondrial (M) crista at the suprabasal locations was observed to be impaired. Normal number but irregular distribution of the fibers was remarkable at subjacent Lp (×15,000).
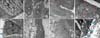
Table 1
Histopathological findings of rectum sections for 4 experimental groups in the model of acute radiation proctitis

Table 2
Immunohistochemical findings for epithelium, crypts and stromal regions of the rectum sections of 4 experimental groups
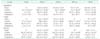
FGF-2, fibroblast growth factor 2; HIF-1α, hypoxia-inducible factor 1α; VEGF, vascular endothelial growth factor.
a)P < 0.05 vs. other groups. b)P < 0.01 vs. other groups. c)P < 0.01 control group vs. treatment and prophylaxis groups. d)P < 0.001 vs. other groups. e)P < 0.05 treatment group vs. prophylaxis group.
References
1. Hauer-Jensen M, Wang J, Boerma M, Fu Q, Denham JW. Radiation damage to the gastrointestinal tract: mechanisms, diagnosis, and management. Curr Opin Support Palliat Care. 2007; 1:23–29.


2. Swaroop VS, Gostout CJ. Endoscopic treatment of chronic radiation proctopathy. J Clin Gastroenterol. 1998; 27:36–40.


3. Novak JM, Collins JT, Donowitz M, Farman J, Sheahan DG, Spiro HM. Effects of radiation on the human gastrointestinal tract. J Clin Gastroenterol. 1979; 1:9–39.


4. Hayne D, Vaizey CJ, Boulos PB. Anorectal injury following pelvic radiotherapy. Br J Surg. 2001; 88:1037–1048.


5. Okunieff P, Cornelison T, Mester M, Liu W, Ding I, Chen Y, et al. Mechanism and modification of gastrointestinal soft tissue response to radiation: role of growth factors. Int J Radiat Oncol Biol Phys. 2005; 62:273–278.


6. Shadad AK, Sullivan FJ, Martin JD, Egan LJ. Gastrointestinal radiation injury: symptoms, risk factors and mechanisms. World J Gastroenterol. 2013; 19:185–198.


7. Anseline PF, Lavery IC, Fazio VW, Jagelman DG, Weakley FL. Radiation injury of the rectum: evaluation of surgical treatment. Ann Surg. 1981; 194:716–724.
8. Frishman WH, Sinatra ST, Moizuddin M. The use of herbs for treating cardiovascular disease. In : Frishman WH, Sonnenblick EH, Sica DA, editors. Cardiovascular pharmacotherapeutics. 2nd ed. New York: McGraw Hill;2004. p. 23–35.
9. Huang YL, Kou JP, Ma L, Song JX, Yu BY. Possible mechanism of the anti-inflammatory activity of ruscogenin: role of intercellular adhesion molecule-1 and nuclear factor-kappaB. J Pharmacol Sci. 2008; 108:198–205.
10. Facino RM, Carini M, Stefani R, Aldini G, Saibene L. Anti-elastase and anti-hyaluronidase activities of saponins and sapogenins from Hedera helix, Aesculus hippocastanum, and Ruscus aculeatus: factors contributing to their efficacy in the treatment of venous insufficiency. Arch Pharm (Weinheim). 1995; 328:720–724.
11. Capra C. Pharmacology and toxicology of some components of Ruscus aculeatus. Fitoterapia. 1972; 43:99–113.
12. Sezer A, Usta U, Kocak Z, Yagci MA. The effect of a flavonoid fractions diosmin + hesperidin on radiation-induced acute proctitis in a rat model. J Cancer Res Ther. 2011; 7:152–156.


13. Erturkuner SP, Yaprak Sarac E, Gocmez SS, Ekmekci H, Ozturk ZB, Seckin İ, et al. Anti-inflammatory and ultrastructural effects of Turkish propolis in a rat model of endotoxin-induced uveitis. Folia Histochem Cytobiol. 2016; 54:49–57.
14. Do NL, Nagle D, Poylin VY. Radiation proctitis: current strategies in management. Gastroenterol Res Pract. 2011; 2011:917941.


15. Gultekin FA, Bakkal BH, Sumer D, Kokturk F, Bektas S. Effects of ozonated olive oil on acute radiation proctitis in rats. Balkan Med J. 2013; 30:369–374.


16. Doi H, Kamikonya N, Takada Y, Fujiwara M, Tsuboi K, Inoue H, et al. Efficacy of polaprezinc for acute radiation proctitis in a rat model. Int J Radiat Oncol Biol Phys. 2011; 80:877–884.


17. Kronfol Z, Remick DG. Cytokines and the brain: implications for clinical psychiatry. Am J Psychiatry. 2000; 157:683–694.


18. Drenth JP, Van Uum SH, Van Deuren M, Pesman GJ, Van der Ven-Jongekrijg J, Van der Meer JW. Endurance run increases circulating IL-6 and IL-1ra but downregulates ex vivo TNF-alpha and IL-1 beta production. J Appl Physiol (1985). 1995; 79:1497–1503.


19. Duff GW. Cytokines and anti-cytokines. Br J Rheumatol. 1993; 32:Suppl 1. 15–20.
20. Karaca G, Aydin O, Pehlivanli F, Altunkaya C, Uzun H, Guler O. Effectiveness of thymoquinone, zeolite, and platelet-rich plasma in model of corrosive oesophagitis induced in rats. Ann Surg Treat Res. 2017; 92:396–401.


21. Cetin M, Capan Y. Basic fibroblast growth factor and a novel approach to its formulations. HacettepeUniversitesi Eczacılık Fakultesi Dergisi. 2004; 24:2.
22. Demirel SH, Cetinkaya S. Hipoksiyl eİnduklenen Faktor-1. Sakaryamj. 2014; 4:171–177.
23. Yazır Y, Gonca S, Filiz S, Dalcık H. An Important protein family for endothelial cells; vascular endothelial growth factor (VEGF) members of the family, structure and synthesis. Cumhur Univ Tıp FakultesiDergisi. 2004; 26:181–184.
24. Ito Y, Kinoshita M, Yamamoto T, Sato T, Obara T, Saitoh D, et al. A combination of pre- and post-exposure ascorbic acid rescues mice from radiation-induced lethal gastrointestinal damage. Int J Mol Sci. 2013; 14:19618–19635.
25. Lu HJ, Tzeng TF, Liou SS, Da Lin S, Wu MC, Liu IM. Ruscogenin ameliorates diabetic nephropathy by its anti-inflammatory and anti-fibrotic effects in streptozotocin-induced diabetic rat. BMC Complement Altern Med. 2014; 14:110.


26. Haboubi NY, Schofield PF, Rowland PL. The light and electron microscopic features of early and late phase radiation-induced proctitis. Am J Gastroenterol. 1988; 83:1140–1144.