Abstract
Adipose tissue-derived stem cell (ASCs) are an attractive source of stem cells with therapeutic applicability in various fields for regenerating damaged tissues because of their stemness characteristics. However, little has reported on evaluating adverse responses caused by human ASC therapy. Therefore, in the present study, a clinical assessment after human ASC transplantation into dogs was undertaken. A total of 12 healthy male dogs were selected and divided into four groups: saline infusion, saline bolus, ASC infusion, and ASC bolus groups. Physical assessment and blood analysis were performed following ASC transplantation, and the concentrations of angiogenic factors, and pro- and anti-inflammatory cytokines were measured by enzyme-linked immunosorbent assay (ELISA). There were no adverse vital sign responses among the dogs. Blood analyses revealed no remarkable complete blood count or serum chemistry results. ELISA results for angiogenic and anti-inflammatory factors including matrix metalloproteinase 9 (MMP9), vascular endothelial growth factor (VEGF), basic fibroblast growth factor (bFGF), hepatocyte growth factor (HGF), and interleukin-10 (IL-10) were significantly higher in the two ASCs groups than in the controls. In conclusion, this study demonstrated that transplantation of human ASCs produced no adverse effects and could be used safely in dogs. In addition, human ASCs could be involved in modulating secretions of angiogenic factors including MMP9, VEGF, bFGF, and HGF and anti-inflammatory factor IL-10.
Mesenchymal stem cells (MSCs) obtained from bone marrow stroma possess the capacity for self-renewal and the potential to differentiate into other cell types of embryonic lineage, including adipocytes [28], muscle cells [12], neurons [36], and placenta [39], depending on the surrounding microenvironment. These characteristics make MSCs potentially useful in various kinds of cell therapy and regenerative medicine [427]. In particular, some studies have demonstrated that MSCs can be isolated from adipose tissue, and this has rapidly increased interest in the developmental and therapeutic potential of MSCs isolated from this tissue [1840]. Because adipose tissue is a readily accessible and abundant source of adult stem cells, MSCs isolated from this source could be ideal candidates for cell therapy and tissue engineering. Several studies have provided evidence that adipose tissue-derived stem cells (ASCs) are multipotent, differentiating in vitro along the adipocyte, chondrocyte, myocyte, and osteoblast lineages, thus representing a promising source of multipotent stem cell for regenerative medicine [51140].
Recently, many studies have demonstrated potential therapeutic effects of ASCs. It was reported that ASCs attenuated pulmonary hypertension by improving pulmonary blood flow and also elevated expression of an anti-inflammatory marker, interleukin-10 (IL-10), in a mouse pulmonary fibrosis model [31]. Another study suggested that systemic infusion of human ASCs alleviated the severe symptom of colitis by regulating inflammatory and autoimmune responses in mice [15]. In addition, it was demonstrated that human ASCs secrete multiple proangiogenic growth factors including vascular endothelial growth factor (VEGF) and hepatocyte growth factor (HGF), which have improved blood perfusion in nude mice [32], while administration of porcine ASCs moderately ameliorated acute myocardial infarction in a porcine model by promoting angiogenic processes in ischemic tissue[14].
However, each of these interventions has revealed some issues because the effects and adverse reactions arising from such stem cell therapies have not been fully characterized. It has been reported that adverse responses were detected following allogenic or xenogenic stem cell transplantations: pulmonary parenchymal hemorrhage in dogs [20], pulmonary sequestration and embolism in mice [13], and collapse and sepsis in humans [17]. Accordingly, as stem cell therapies are being used more frequently in regenerative medicine, the need to control the processing of stem cells continues to grow in order to prevent a high risk of complications and adverse effects in the recipient. Therefore, the aim of study was to assess the safety, based on physical and blood examinations, of intravenous transplantation of human ASCs into dogs, which share similar physiological characteristics with humans. In addition, further investigation of ASCs and their angiogenic, pro- and anti-inflammatory effects after transplantation was performed to elucidate the beneficial effects of human ASCs on dogs.
To the best of our knowledge, few investigations have reported physiological assessment of human ASCs posttransplantation into intact animals, particularly whether ASCs exhibit angiogenic and inflammatory characteristics, which are some of the main functions of ASCs. In one previous report, three different concentrations (5.0 × 106, 3.5 × 107, or 2.5 × 108 cells/kg) of human MSCs were injected into mice using different injection rates, and no adverse events were observed except at the highest dose with rapid injection [30]. Therefore, based on that previous research outcome, in the present study, we transplanted human ASCs intravenously at a specific dose (7.5 × 106 cells/mL, total 10 mL), infusion rate (3 mL/min), and bolus rate (60 mL/min) into intact dogs. Following transplantation of ASCs, we performed clinical evaluations based on physical assessment and blood analysis. In addition, we measured the concentration of matrix metalloproteinase 9 (MMP9), VEGF, basic fibroblast growth factor (bFGF), HGF, tumor necrosis factor-α(TNF-α), and IL-10 in the treated dogs to investigate the effect of ASCs on secretions of various angiogenic and pro-inflammatory/anti-inflammatory factors.
Twelve healthy, 4-year-old, intact male beagle dogs without clinical signs of disease were recruited for this research. All dogs were continuously monitored throughout the research period and fed a consistent amount of commercial adult dry food (Natural Balance; Natural Balance Pet Food, USA) and water daily. Also, all dogs were housed in an identical manner since their birth in individual cages. Protocols for the ethical use of animals were followed during the course of this research. All experiments and studies were conducted in accordance with recommendations described in “The Guide for the Care and Use of Laboratory Animals” published by the Institutional Animal Care and Use Committee of Seoul National University (approval No. SNU-150331-4). In this regard, dog care facilities and the procedures performed met or exceeded the standards established by the Committee for Accreditation of Laboratory Animal Care at Seoul National University.
The procedure for preparation of human ASCs was performed under Good Manufacturing Practices conditions at the R Bio Stem Cell Research Center (Korea) with approval from the Life Ethics Committee of the Biostar Stem Cell Institute (RBIO 2015-12-001; Korea). The ASCs were isolated from disposed human adipose tissues obtained from the lower abdomen of patients, with their agreement, and were primary cultured as previously described [30]. In detail, human subcutaneous adipose tissues were obtained by simple liposuction from abdominal subcutaneous fat with informed consent and digested with collagenase I (1 mg/mL) under gentle agitation for 60 min at 37℃. The digested tissues were then filtered through a 100-µm nylon sieve. The tissues were centrifuged at 470 × g for 5 min and resuspended in a Dulbecco's modified Eagle's medium (DMEM; Invitrogen, USA)-based medium containing 0.2 mM ascorbic acid and 10% fetal bovine serum (FBS). After re-centrifuging at 470 × g for 5 min, the supernatant was discarded and the cell pellet collected. The obtained cells were cultured overnight at 37℃ under 5% CO2 in DMEM-based medium containing 0.2 mM ascorbic acid and 10% FBS. The cell medium was changed to Keratinocyte-SFM (Invitrogen)-based medium containing 0.2 mM ascorbic acid, 0.09 mM calcium, 5 ng/mL recombinant epidermal growth factor (rEGF), and 5% FBS. The cells were maintained for 4 to 5 days until confluent (passage 0). When the cells reached 90% confluency, they were subculture-expanded in Keratinocyte-SFM-based medium containing 0.2 mM ascorbic acid, 0.09 mM calcium, 5 ng/mL rEGF, and 5% FBS and cryopreserved in 10% dimethyl sulfoxide containing a freezing solution. Cryopreserved ASC were thawed and cultured until 90% confluency, and then trypsinized for use in ASC transplantation.
The 12 healthy male dogs were divided into four groups: (i) 10 mL saline infusion (n = 3, injection rate 3 mL/min); (ii) 10 mL saline bolus (n = 3, 60 mL/min); (iii) 10 mL ASC infusion (n = 3, 7.5 × 106 cells/mL, injection rate 3 mL/min); and (iv) 10 mL ASC bolus (n = 3, 7.5 × 106 cells/mL, injection rate 60 mL/min). The saline and prepared ASCs were injected into the cephalic vein through a catheter.
Physical examinations such as determining body temperature, respiratory rate, and pulse rate were performed before and every 5 min after transplantation for up to 30 min. In addition, for monitoring the occurrence of adverse responses, electrocardiography (ECG) was performed and neurological symptoms, swelling of the four limbs, and heart murmur were assessed before injection and at 30 min after injection. Lead II ECG tracings were recorded from each dog. For evaluation of neurological symptoms, cranial nerve reflexes (olfaction, menace, pupillary light reflex, cotton ball, strabismus, nystagmus, palpebral reflex, hearing, and gag reflex) and postural reflexes (proprioception, hemi-standing/walking, wheelbarrowing, placing reaction-tactile/visual, and withdrawal reflex) were examined.
Complete blood counts (CBC) and serum chemistry panel tests were performed (IDEXX Laboratories, Korea) on days 1, 7, 14, 21, and 28 post-transplantation of saline or ASCs for assessment of adverse responses to transplantation. A total of 8 parameters for CBC tests were measured: red blood cell (RBC), white blood cell (WBC), packed cell volume (PCV), hemoglobin (HB), and platelet (PLT), neutrophil (NEUT), basophil (BASO), and eosinophil (EOS) counts. In addition, a serum chemistry panel test was performed including measurement of sodium (Na), potassium (K), chlorine (Cl), alkaline phosphatase (ALP), alanine aminotransferase (ALT), aspartate aminotransferase (AST), blood urea nitrogen/urea (BUN/UREA), creatinine (CREA), phosphorus (P) and calcium (Ca) levels.
For ELISA analysis, blood samples were collected, taking aseptic precautions, and allowed to clot to obtain serum, then centrifuged at 800 g for 10 min to separate serum. Sera were stored at −80℃ until use. The concentrations of TNF-α (R&D Systems, USA), IL-10 (R&D Systems), VEGF (R&D Systems), HGF (Biorbyt, UK), MMP9 (USCN Life Science, China) and bFGF (MyBioSource, USA) in serum from each dog were analyzed by ELISA. The assays were performed following the manufacturer's instructions. Briefly, standard solutions and samples were added to each well of the ELISA plates and incubated for 1 to 2 h at room temperature. To remove unbound antigen, wash buffer using a squirt bottle was applied five times to each well. After the last wash, conjugates were added to each well and samples were again incubated for 1 to 2 h at room temperature and washed five times with wash buffer. Substrate solutions were then added to each well followed by incubation for 30 min at room temperature with protection from light. Lastly, stop solutions were added to each well, and gentle tapping was performed to ensure thorough mixing. The spectroscopic absorbance of each well was determined immediately by using a microplate reader (Tecan Sunrise, USA) at 450 nm excitation/590 nm emission.
Statistical analysis was performed by using GraphPad Prism 5.0 (GraphPad, USA). Differences among the groups were examined with two-way ANOVA followed by the Bonferroni post-test. Differences associated with p < 0.05 were considered significant, and all data are presented as mean ± SEM values.
There were no remarkable clinical results for any of the dogs. Also, there were no significant differences in vital signs such as heart rate, respiratory rate, and body temperature (p > 0.05), and all vital signs were within normal ranges for all of the dogs after transplantation (Fig. 1). In addition, an ECG test was performed before and after transplantation in order to evaluate adverse circulatory responses such as arrhythmia and coronary artery anomalies. All groups showed normal patterns of wave interval and height (P width, P height, PR interval, QRS width, R height and QT interval) before and after saline or ASC transplantation (panel A in Fig. 2). Auscultation of heart murmur, neurological symptoms, and signs of swelling in the limbs were recorded for 30 min (panels B and C in Fig. 2); no group showed adverse responses such as heart murmur or limb swelling, and all dogs showed normal neurological responses for cranial nerve reflexes and postural reflexes.
We analyzed CBC and serum chemistry results on days 1, 7, 14, 21, and 28 post-transplantation of saline or ASCs to survey for possible adverse effects in all dogs (Figs. 3 and 4). All serial test results for CBC after transplantation were within normal ranges and showed no significant differences among the four groups (reference intervals: WBC, 5.2–17.0 K/µL; RBC, 5.7–8.8 M/µL; PLT, 143–400 K/µL; PCV, 37.1%–57.0%; HB, 12.9–18.4 g/dL; BASO, 0.0–0.5 K/µL; EOS, 0.0–1.82 K/µL; NEUT, 3.2–10.9 K/µL). Moreover, the serum chemistry results post-transplantation were within normal ranges for all groups (reference intervals: Na, 145–155 mmol/L; K, 2.7–5.5 mmol/L; Cl, 96–122 mmol/L; ALP, 0.0–97.9 U/L; ALT, 5.8–83.3 U/L; AST, 11.7–42.5 U/L; CREA, 0.4–1.3 mg/dL; albumin, 2.6–4.4 g/dL; BUN/UREA, 6.0–24.0 mg/dL; total protein, 5.4–7.5 g/dL; Ca, 8.0–11.9 mg/dL; P, 1.3–6.3 mg/dL). However, there were significant differences in serial test results for BUN/UREA and P. The BUN/UREA results revealed significant differences among groups on days 7 and 21, whereas the P results showed a significant difference only on day 21 following transplantation of ASCs. There was significantly increased BUN/UREA in the ASC bolus group (17.7 ± 0.3 mg/dL) compared with levels in other groups on day 7 post-transplantation (p < 0.05). Also, on day 21 post-transplantation, the saline infusion and saline bolus groups (19.0 ± 2.0 and 22.0 ± 2.1 mg/dL, respectively) showed significantly higher concentrations of BUN/UREA than those of the ASC infusion and ASC bolus groups (10.0 ± 0.6 and 10.7 ± 0.9 mg/dL, respectively). In addition, there was significantly increased P in the saline infusion group (5.5 ± 0.0 mg/dL) compared with the levels in the other three groups on day 21 following transplantation (p < 0.05).
The concentrations of MMP9, VEGF, bFGF, HGF, TNF-α, and IL-10 in serum of each group on days 1, 7, 14, 21, and 28 post-transplantation of saline or ASCs were analyzed (Fig. 5). There was no significant difference in serum TNF-αconcentrations among the four groups, whereas the concentration of MMP9 was significantly high in both ASC groups on days 7 and 14 following transplantation (p < 0.05): ASCs infusion group 76.9 ± 5.8 and 89.1 ± 7.6 ng/mL, respectively; ASC bolus group 78.7 ± 5.3 and 74.0 ± 4.0 mg/mL; saline infusion group 48.5 ± 3.6 and 57.0 ± 2.2 ng/mL; saline bolus group 51.1 ± 1.6 and 53.2 ± 1.7 ng/mL.
The serum concentrations of VEGF were significantly increased in the saline bolus and ASC bolus groups on day 1 post-transplantation: 114.1 ± 7.3 and 114.1 ± 2.7 pg/mL, respectively, compared with those of the infusion groups. On day 21 post-transplantation, VEGF was significantly upregulated in the ASC infusion group (150.4 ± 18.1 pg/mL) compared with the other groups, and, on day 28 post-transplantation, VEGF was significantly increased in the ASCs infusion group (124.4 ± 9.3 pg/mL) compared to that in the saline bolus group.
The bFGF concentrations in both ASC groups were significantly increased on days 14 and 21 (ASC infusion group 13.9 ± 2.9 and 10.9 ± 3.0 pg/mL, respectively; ASC bolus group 9.8 ± 1.2 and 10.1 ± 2.0 pg/mL) compared with those in the saline groups. On day 28 post-transplantation, bFGF was significantly increased in the ASC infusion group (9.2 ± 3.5 pg/mL) compared to those in the saline groups.
The concentration of HGF was significantly upregulated in both ASC groups on days 7, 14, 21, and 28 following transplantation (ASC infusion group 1.4 ± 0.1, 2.9 ± 0.4, 3.0 ± 0.2, and 2.8 ± 0.2 pg/mL, respectively; ASC bolus group 1.7 ± 0.1, 2.3 ± 0.2, 2.5 ± 0.2, and 2.3 ± 0.2 pg/mL) compared with the saline groups.
The serum concentration of IL-10 in the ASC infusion group was significantly upregulated on days 1, 7, 14, 21, and 28 post-transplantation (36.8 ± 4.7, 48.7 ± 7.1, 33.7 ± 4.4, 38.1 ± 6.1, and 35.6 ± 4.8, respectively) compared to those in the other groups.
Use of MSCs from tissues, bone marrow, or cord blood is a promising technology that can overcome the limitations of current medical technologies. These cells can be applied to various incurable diseases, including critical limb ischemia and arthritis, which cannot be treated with current medical technology [1622]. In clinical applications, MSCs have favorable properties, such as having immunomodulatory characteristics and protective effects on immunological disorders [212938] as well as angiogenic effects [37]. However, those properties may also have adverse effects. Some studies have demonstrated that transplanting MSCs into mouse brain elicits an inflammatory response and rapid rejection [89]. Moreover, it was reported that the immunosuppressive capacity of MSCs may, in some cases, favor the growth of tumor cells [10], and ASCs can promote growth of breast tumors and metastasis [24]. These contradictory results indicate that investigations into the characteristics of ASCs are far from complete, and further studies and consensual research to clinically assess transplantation of ASCs are necessary to fully elucidate their effects because there are no reports assessing the deleterious aspects of ASC transplantation into intact animals. To address these issues, we investigated clinical alterations after human ASC transplantation into healthy dogs to investigate possible applications in human clinical therapies. Furthermore, to determine whether transplanted human ASCs can maintain their positive aspects, including pro-inflammatory/anti-inflammatory and angiogenic properties as mentioned above, ELISA analyses were carried out to evaluate these characteristics.
In the present study, our results showed that after intravenous injection of human ASCs with two different injection rates (infusion and bolus), there was no incidence of adverse reactions in laboratory studies including physical examination (heart rate, respiratory rate, body temperature, heart murmur, ECG, neurological symptoms, swelling of four limbs). A previous study reported that MSCs transplanted into mice (15.7–24.3 g body weight) via a high dose (2.0 × 108 cells/kg) bolus injection died soon afterward, while no adverse events were observed when the high dose was injected slowly [30]. In the present study, we obtained no abnormal clinical assessment results related to injection rates. In addition, blood analysis results showed that all parameters were within normal ranges post-transplantation. Also, there were no significant differences among groups, except for P and BUN/UREA levels which did show significant differences among groups after transplantation. A previous study reported that the level of BUN could vary depending on the measurement methods [7]. In support of our results, there was no adverse response based on the BUN results, and we suggest that significant differences in these factors (P and BUN/UREA) might be caused by variations in measurement methods.
In the present study, we measured the concentration of angiogenic and pro-inflammatory/anti-inflammatory factors in serum following ASC transplantation. We found that various angiogenic factors including MMP9, VEGF, bFGF, and HGF were increased, which could lead to angiogenesis.
MMPs produced by stromal cells and adipocytes are capable of degrading the extracellular matrix (ECM) to facilitate invading endothelial cells [3]. Also, they are prerequisite factors for releasing ECM-sequestered proangiogenic factors including VEGFs and FGFs that have been clearly implicated in angiogenesis [2]. In support of those results, our study showed that the concentration of MMP9 was significantly increased in the ASC groups on days 7 and 14 following transplantation along with increased levels of VEGF and bFGF. Therefore, the results indicate that MMP9 might have facilitated the release and increase in the levels of VEGF and bFGF on days 21 and 14, respectively, post-transplantation.
ASCs make a considerable contribution to angiogenesis by producing VEGF, HGF, and bFGF [2635]. In particular, it has been determined that VEGF and HGF act in a synergistic manner, which makes them valuable in angiogenesis therapy [34]. Therefore, ASCs could contribute to angiogenesis by secreting angiogenic molecules and supplying vascular cells to blood vessels. For instance, increased expression of VEGF mRNA and protein has led to rapid and dramatic increases in myocardial capillary diameter, capillary density, and blood flow in anemic fetal sheep [23]. Another study found that when human ASCs were cultured in vitro, consistent augmentation of HGF secretion was observed by ELISA [25]. It was also reported that MSC implantation into ischemic limbs and myocardium markedly improved vessel formation, blood perfusion, and capillary density associated with release of large amounts of VEGF and bFGF [1933]. In our study, transplantation of ASCs into intact animals induced high levels of VEGF, bFGF, and HGF. The results indicate that transplantation of ASCs into intact animals might invoke angiogenesis by markedly increasing the concentrations of VEGF, bFGF, and HGF.
A previous study proposed that MSCs mediate their immunomodulatory effects by controlling various kinds of cytokines including TNF-α and IL-10, which are pro-inflammatory and anti-inflammatory cytokines, respectively [6]. Another report demonstrated the immunomodulatory functions of human MSCs by co-culturing them with immune cells and suggested that human MSCs changed the secretion of cytokines in immune cells by inducing secretion of IL-10 and decreasing that of TNF-α [1]. That study proposed that when human MSCs are present in an inflammatory environment they could change the on-going immune response, thereby causing a shift from a pro-inflammatory situation to an anti-inflammatory one. Thus, they demonstrated a close interaction between human MSCs and immunomodulatory cytokines. Our results showed that the level of IL-10 was significantly increased in the ASC infusion group compared with that in other groups after transplantation, while there were no significant differences in TNF-α concentrations. Therefore, we assume that ASCs might mediate the high expression of IL-10 in intact animals.
In conclusion, transplantation of human ASCs intravenously into intact dogs elicited no adverse responses based on our physical assessment and blood analysis results. Thus, for the first time, we have demonstrated that human ASCs can be safely transplanted into dogs for clinical use. Furthermore, transplantation of human ASCs could induce a markedly high concentration of angiogenic factors (MMP9, VEGF, bFGF, HGF) and the anti-inflammatory factor IL-10, which suggests that human ASCs might represent a novel therapeutic tool for stimulating anti-inflammatory and angiogenic factors in animals with ischemic vascular diseases.
Figures and Tables
Fig. 1
Assessment of (A) heart rate (normal range, 60–180 beats/min), (B) body temperature (normal range, 37.7℃–39.1℃), and (C) respiratory rate (normal range, 10–35 breaths/min) after the saline or adipose tissue-derived stem cell (ASC) transplantation. No significant differences in vital signs among the four groups (p > 0.05) and all groups were within normal ranges. 0, 5, 10, 15, 20, 25, 30 min: time after saline or ASC transplantation (infusion rate, 3 mL/min; bolus rate, 60 mL/min).
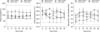
Fig. 2
Physical examination outcomes. (A) Representative lead II electrocardiography (ECG) tracing recorded from a member of each group (saline infusion, saline bolus, adipose tissue-derived stem cells [ASCs] infusion, and ASCs bolus) after transplantation of saline of ASCs. (B) Observation of limb swelling in representatives of each group after transplantation of saline or ASCs. (C) Physical examination outcome for heart murmur and neurological symptom. For evaluation of neurological symptom, cranial nerve reflexes and postural reflexes were examined. NE, none exist.
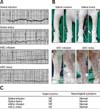
Fig. 3
Evaluation of complete blood cell count after saline or adipose tissue-derived stem cell (ASC) transplantation. All groups were within normal ranges. 1, 7, 14, 21, 28 days: time after the saline or ASC transplantation (infusion rate, 3 mL/min; bolus rate, 60 mL/min). Each group had 3 dogs and an analysis was performed on days 1, 7, 14, 21, and 28 post-transplantation in each dog. WBC, white blood cell; RBC, red blood cell; PLT, platelet; PCV, packed cell volume; HB, hemoglobin; BASO, basophil; EOS, eosinophil; NEUT, neutrophil.
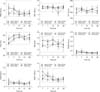
Fig. 4
Evaluation of serum chemistry analysis after the saline or adipose tissue-derived stem cell (ASC) transplantation. a,bWithin groups, values with different superscript letters are significantly different (p < 0.05) and all groups were within normal ranges. 1, 7, 14, 21, 28 days: time after the saline or ASC transplantation (infusion rate, 3 mL/min; bolus rate, 60 mL/min). Each group had 3 dogs and an analysis was performed on days 1, 7, 14, 21, and 28 post-transplantation in each dog. Na, sodium; K, potassium; Cl, chloride; ALP, alkaline phosphatase; ALT, alanine aminotransferase; AST, aspartate aminotransferase; CREA, creatinine; BUN/UREA, blood urea nitrogen/urea; Ca, calcium; P, phosphorus.
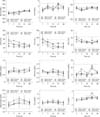
Fig. 5
Concentration of matrix metalloproteinase 9 (MMP9), vascular endothelial growth factor (VEGF), basic fibroblast growth factor (bFGF), hepatocyte growth factor (HGF), tumor necrosis factor (TNF)-α, and interleukin-10 (IL-10) from serum in each group after the saline or adipose tissue-derived stem cell (ASC) transplantation. a,b,cWithin groups, values with different superscript letters are significantly different (p < 0.05). 1, 7, 14, 21, 28 days: time after the saline or ASCs transplantation (infusion rate: 3 mL/min, bolus rate: 60 mL/min). Each group had 3 dogs and an analysis was performed on days 1, 7, 14, 21, 28 post-transplantation in each dog.
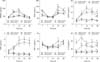
Acknowledgments
This research was supported by Nature Cell (No. 550-20150030), Global Ph.D. Fellowship Program through the NRF funded by the Ministry of Education (NRF-20142A1021187), RDA (No. PJ010928032016), IPET (No. 316002-05-1-SB010), and the Research Institute for Veterinary Science BK21 Plus program.
References
1. Aggarwal S, Pittenger MF. Human mesenchymal stem cells modulate allogeneic immune cell responses. Blood. 2005; 105:1815–1822.


2. Ardi VC, Van den Steen PE, Opdenakker G, Schweighofer B, Deryugina EI, Quigley JP. Neutrophil MMP-9 proenzyme, unencumbered by TIMP-1, undergoes efficient activation in vivo and catalytically induces angiogenesis via a basic fibroblast growth factor (FGF-2)/FGFR-2 pathway. J Biol Chem. 2009; 284:25854–25866.


3. Bouloumié A, Sengenès C, Portolan G, Galitzky J, Lafontan M. Adipocyte produces matrix metalloproteinases 2 and 9: involvement in adipose differentiation. Diabetes. 2001; 50:2080–2086.


4. Bruder SP, Jaiswal N, Ricalton NS, Mosca JD, Kraus KH, Kadiyala S. Mesenchymal stem cells in osteobiology and applied bone regeneration. Clin Orthop Relat Res. 1998; (355 Suppl):S247–S256.


5. Cao Y, Sun Z, Liao L, Meng Y, Han Q, Zhao RC. Human adipose tissue-derived stem cells differentiate into endothelial cells in vitro and improve postnatal neovascularization in vivo. Biochem Biophys Res Commun. 2005; 332:370–379.


6. Caplan AI. Adult mesenchymal stem cells for tissue engineering versus regenerative medicine. J Cell Physiol. 2007; 213:341–347.


7. Choi JH, Kim KL, Huh W, Kim B, Byun J, Suh W, Sung J, Jeon ES, Oh HY, Kim DK. Decreased number and impaired angiogenic function of endothelial progenitor cells in patients with chronic renal failure. Arterioscler Thromb Vasc Biol. 2004; 24:1246–1252.


8. Coyne TM, Marcus AJ, Reynolds K, Black IB, Woodbury D. Disparate host response and donor survival after the transplantation of mesenchymal or neuroectodermal cells to the intact rodent brain. Transplantation. 2007; 84:1507–1516.


9. Coyne TM, Marcus AJ, Woodbury D, Black IB. Marrow stromal cells transplanted to the adult brain are rejected by an inflammatory response and transfer donor labels to host neurons and glia. Stem Cells. 2006; 24:2483–2492.


10. Djouad F, Plence P, Bony C, Tropel P, Apparailly F, Sany J, Noël D, Jorgensen C. Immunosuppressive effect of mesenchymal stem cells favors tumor growth in allogeneic animals. Blood. 2003; 102:3837–3844.


11. El-Badawy A, Amer M, Abdelbaset R, Sherif SN, Abo-Elela M, Ghallab YH, Abdelhamid H, Ismail Y, El-Badri N. Adipose stem cells display higher regenerative capacities and more adaptable electro-kinetic properties compared to bone marrow-derived mesenchymal stromal cells. Sci Rep. 2016; 6:37801.


12. Ferrari G, Cusella-De Angelis G, Coletta M, Paolucci E, Stornaiuolo A, Cossu G, Mavilio F. Muscle regeneration by bone marrow-derived myogenic progenitors. Science. 1998; 279:1528–1530.


13. Furlani D, Ugurlucan M, Ong L, Bieback K, Pittermann E, Westien I, Wang W, Yerebakan C, Li W, Gaebel R, Li RK, Vollmar B, Steinhoff G, Ma N. Is the intravascular administration of mesenchymal stem cells safe? Mesenchymal stem cells and intravital microscopy. Microvasc Res. 2009; 77:370–376.
14. Gómez-Mauricio G, Moscoso I, Martín-Cancho MF, Crisóstomo V, Prat-Vidal C, Báez-Díaz C, Sánchez-Margallo FM, Bernad A. Combined administration of mesenchymal stem cells overexpressing IGF-1 and HGF enhances neovascularization but moderately improves cardiac regeneration in a porcine model. Stem Cell Res Ther. 2016; 7:94.


15. González MA, Gonzalez-Rey E, Rico L, Büscher D, Delgado M. Adipose-derived mesenchymal stem cells alleviate experimental colitis by inhibiting inflammatory and autoimmune responses. Gastroenterology. 2009; 136:978–989.


16. González MA, Gonzalez-Rey E, Rico L, Büscher D, Delgado M. Treatment of experimental arthritis by inducing immune tolerance with human adipose-derived mesenchymal stem cells. Arthritis Rheum. 2009; 60:1006–1019.


17. Granton J, Langleben D, Kutryk MB, Camack N, Galipeau J, Courtman DW, Stewart DJ. Endothelial NO-synthase gene-enhanced progenitor cell therapy for pulmonary arterial hypertension: the PHACeT trial. Circ Res. 2015; 117:645–654.


18. Halvorsen YD, Franklin D, Bond AL, Hitt DC, Auchter C, Boskey AL, Paschalis EP, Wilkison WO, Gimble JM. Extracellular matrix mineralization and osteoblast gene expression by human adipose tissue-derived stromal cells. Tissue Eng. 2001; 7:729–741.


19. Iwase T, Nagaya N, Fujii T, Itoh T, Murakami S, Matsumoto T, Kangawa K, Kitamura S. Comparison of angiogenic potency between mesenchymal stem cells and mononuclear cells in a rat model of hindlimb ischemia. Cardiovasc Res. 2005; 66:543–551.


20. Kang MH, Park HM. Evaluation of adverse reactions in dogs following intravenous mesenchymal stem cell transplantation. Acta Vet Scand. 2014; 56:16.


21. Krampera M, Cosmi L, Angeli R, Pasini A, Liotta F, Andreini A, Santarlasci V, Mazzinghi B, Pizzolo G, Vinante F, Romagnani P, Maggi E, Romagnani S, Annunziato F. Role for interferon-gamma in the immunomodulatory activity of human bone marrow mesenchymal stem cells. Stem Cells. 2006; 24:386–398.


22. Lu D, Chen B, Liang Z, Deng W, Jiang Y, Li S, Xu J, Wu Q, Zhang Z, Xie B, Chen S. Comparison of bone marrow mesenchymal stem cells with bone marrow-derived mononuclear cells for treatment of diabetic critical limb ischemia and foot ulcer: a double-blind, randomized, controlled trial. Diabetes Res Clin Pract. 2011; 92:26–36.


23. Martin C, Yu AY, Jiang BH, Davis L, Kimberly D, Hohimer AR, Semenza GL. Cardiac hypertrophy in chronically anemic fetal sheep: Increased vascularization is associated with increased myocardial expression of vascular endothelial growth factor and hypoxia-inducible factor 1. Am J Obstet Gynecol. 1998; 178:527–534.


24. Muehlberg FL, Song YH, Krohn A, Pinilla SP, Droll LH, Leng X, Seidensticker M, Ricke J, Altman AM, Devarajan E, Liu W, Arlinghaus RB, Alt EU. Tissue-resident stem cells promote breast cancer growth and metastasis. Carcinogenesis. 2009; 30:589–597.


25. Nakagami H, Maeda K, Morishita R, Iguchi S, Nishikawa T, Takami Y, Kikuchi Y, Saito Y, Tamai K, Ogihara T, Kaneda Y. Novel autologous cell therapy in ischemic limb disease through growth factor secretion by cultured adipose tissue-derived stromal cells. Arterioscler Thromb Vasc Biol. 2005; 25:2542–2547.


26. Nakagami H, Morishita R, Maeda K, Kikuchi Y, Ogihara T, Kaneda Y. Adipose tissue-derived stromal cells as a novel option for regenerative cell therapy. J Atheroscler Thromb. 2006; 13:77–81.


27. Pittenger M, Vanguri P, Simonetti D, Young R. Adult mesenchymal stem cells: potential for muscle and tendon regeneration and use in gene therapy. J Musculoskelet Neuronal Interact. 2002; 2:309–320.
28. Pittenger MF, Mackay AM, Beck SC, Jaiswal RK, Douglas R, Mosca JD, Moorman MA, Simonetti DW, Craig S, Marshak DR. Multilineage potential of adult human mesenchymal stem cells. Science. 1999; 284:143–147.


29. Puissant B, Barreau C, Bourin P, Clavel C, Corre J, Bousquet C, Taureau C, Cousin B, Abbal M, Laharrague P, Penicaud L, Casteilla L, Blancher A. Immunomodulatory effect of human adipose tissue-derived adult stem cells: comparison with bone marrow mesenchymal stem cells. Br J Haematol. 2005; 129:118–129.


30. Ra JC, Shin IS, Kim SH, Kang SK, Kang BC, Lee HY, Kim YJ, Jo JY, Yoon EJ, Choi HJ, Kwon E. Safety of intravenous infusion of human adipose tissue-derived mesenchymal stem cells in animals and humans. Stem Cells Dev. 2011; 20:1297–1308.


31. Rathinasabapathy A, Bruce E, Espejo A, Horowitz A, Sudhan DR, Nair A, Guzzo D, Francis J, Raizada MK, Shenoy V, Katovich MJ. Therapeutic potential of adipose stem cell-derived conditioned medium against pulmonary hypertension and lung fibrosis. Br J Pharmacol. 2016; 173:2859–2879.


32. Rehman J, Traktuev D, Li J, Merfeld-Clauss S, Temm-Grove CJ, Bovenkerk JE, Pell CL, Johnstone BH, Considine RV, March KL. Secretion of angiogenic and antiapoptotic factors by human adipose stromal cells. Circulation. 2004; 109:1292–1298.


33. Tateishi-Yuyama E, Matsubara H, Murohara T, Ikeda U, Shintani S, Masaki H, Amano K, Kishimoto Y, Yoshimoto K, Akashi H, Shimada K, Iwasaka T, Imaizumi T. Therapeutic angiogenesis for patients with limb ischaemia by autologous transplantation of bone-marrow cells: a pilot study and a randomised controlled trial. Lancet. 2002; 360:427–435.


34. Van Belle E, Witzenbichler B, Chen D, Silver M, Chang L, Schwall R, Isner JM. Potentiated angiogenic effect of scatter factor/hepatocyte growth factor via induction of vascular endothelial growth factor: the case for paracrine amplification of angiogenesis. Circulation. 1998; 97:381–390.


35. Weimar IS, Miranda N, Muller EJ, Hekman A, Kerst JM, de Gast GC, Gerritsen WR. Hepatocyte growth factor/scatter factor (HGF/SF) is produced by human bone marrow stromal cells and promotes proliferation, adhesion and survival of human hematopoietic progenitor cells (CD34+). Exp Hematol. 1998; 26:885–894.
36. Woodbury D, Schwarz EJ, Prockop DJ, Black IB. Adult rat and human bone marrow stromal cells differentiate into neurons. J Neurosci Res. 2000; 61:364–370.


37. Wu Y, Chen L, Scott PG, Tredget EE. Mesenchymal stem cells enhance wound healing through differentiation and angiogenesis. Stem Cells. 2007; 25:2648–2659.


38. Zappia E, Casazza S, Pedemonte E, Benvenuto F, Bonanni I, Gerdoni E, Giunti D, Ceravolo A, Cazzanti F, Frassoni F, Mancardi G, Uccelli A. Mesenchymal stem cells ameliorate experimental autoimmune encephalomyelitis inducing T-cell anergy. Blood. 2005; 106:1755–1761.


39. Zhang Y, Li CD, Jiang XX, Li HL, Tang PH, Mao N. Comparison of mesenchymal stem cells from human placenta and bone marrow. Chin Med J (Engl). 2004; 117:882–887.