Abstract
Transgenic (TG) pigs are important in biomedical research and are used in disease modeling, pharmaceutical toxicity testing, and regenerative medicine. In this study, we constructed two vector systems by using the promoter of the pig glial fibrillary acidic protein (pGFAP) gene, which is an astrocyte cell marker. We established donor TG fibroblasts with pGFAP-CreERT2/LCMV-EGFPLoxP and evaluated the effect of the transgenes on TG-somatic cell nuclear transfer (SCNT) embryo development. Cleavage rates were not significantly different between control and transgene-donor groups. Embryo transfer was performed thrice just before ovulation of the surrogate sows. One sow delivered 5 TG piglets at 115 days after pregnancy. Polymerase chain reaction (PCR) analysis with genomic DNA isolated from skin tissues of TG pigs revealed that all 5 TG pigs had the transgenes. EGFP expression in all organs tested was confirmed by immunofluorescence staining and PCR. Real-time PCR analysis showed that pGFAP promoter-driven Cre fused to the mutated human ligand-binding domain of the estrogen receptor (CreERT2) mRNA was highly expressed in the cerebrum. Semi-nested PCR analysis revealed that CreERT2-mediated recombination was induced in cerebrum and cerebellum but not in skin. Thus, we successfully generated a TG pig with a 4-hydroxytamoxifen (TM)-inducible pGFAP-CreERT2/EGFPLoxP recombination system via SCNT.
Among alternative laboratory models, pigs are most suitable because they have cardiovascular, renal, and gastrointestinal organs similar to those of humans [2136]. However, transgenic (TG) pig production efficiency is very low compared to that of other mammals such as cattle, sheep, and mice due to incomplete reprogramming of somatic cell nuclear transfer (SCNT) embryos [32]. Therefore, unstable development and unsuccessful implantation of SCNT embryos limit the production of TG pigs [2338]. In genetically engineered animals, early development of embryos has been reported to be affected by abnormal expression of foreign genes, causing miscarriage or early mortality of TG animals [35]. To overcome this limitation, many researchers have recently applied conditional transgene expression systems such as the ecdysone-inducible gene switch, tetracycline-inducible system, or Gal4/UAS system to prevent inhibition of embryonic development and to induce gene expression as desired by the researcher [2033]. These systems have begun to be used in large animals, such as pigs, in addition to their original use in rodents. In 2006, the first TG pigs with a tetracycline-inducible system were produced by a pronuclear DNA microinjection technique [25].
The CreER-LoxP system is a frequently used site-specific recombination system for averting undesirable development outcomes such as embryonic or postnatal lethality [2631]. Cre recombinase recognizes two consensus sequences, called locus of X(cross)-over in P1(LoxP), and catalyzes excision, insertion, inversion, or even translocation of target DNA sequences in accordance with their location and orientation [12]. In addition, CreERT2, a fusion protein of Cre recombinase and the modified estrogen receptor (ER) with a higher sensitivity to its optimal ligand 4-hydroxytamoxifen (TM) than to endogenous estradiol, was developed to control the timing of a recombination event by treatment with the ligand [1030]. This system has been widely applied to TG mouse models that require conditional activation or knock-out of genes of interest [39].
Glial fibrillary acidic protein (GFAP), a class III intermediate filament protein, is an astrocytic cell lineage marker [7]. A 2.2 kb 5′-flanking sequence of the human GFAP (hGFAP) gene, called Gfa2, has been identified and used to induce the expression of oncogenes specifically in astrocytes in numerous brain tumor mouse models [217]. However, the mouse brain is 750 times smaller than the human brain, and it includes a much lower amount of white matter than that in the human brain. Furthermore, the mouse brain is lissencephalic (i.e., it has a smooth outer cerebral cortex). In contrast, the pig brain is 7.5 times smaller than the human brain, has a white-to-gray matter ratio (60:40) similar to that of humans, and is gyrencephalic (i.e., it has a highly convoluted outer cerebral cortex), similar to the human brain. Thus, the pig is a better animal model than the mouse for research in human brain diseases [1416].
In the present study, we constructed pig GFAP promoter-CreERT2 [8] and enhanced green fluorescent protein (EGFP)-LoxP transgenes for use in TM-inducible CreERT2-mediated recombination. We then established donor TG pig fibroblasts with pGFAP-CreERT2/LCMV-EGFPLoxP transgenes for use in SCNT. After SCNT, we evaluated the effect of the transgenes on TG-SCNT embryo development. After embryo transfer, we generated 5 TG pigs and confirmed the presence of the transgenes and TM-inducible CreERT2-mediated recombination in a living TG pig.
The experimental protocol was approved by the Committee on Ethics of Animal Experiments of the Chungbuk National University (permission No. CBNUR-871-15). All surgeries were performed under anesthesia, and we tried to minimize the suffering of the animals.
The equipment and reagents used in this study are detailed in the following sections. All other chemicals were purchased from Sigma-Aldrich (USA).
To construct pCDH-pGFAP-MCS-EF1-puro, the CMV promoter sequence of the pCDH-CMV-MCS-EF1-puro lentiviral vector (System Biosciences, USA) was removed by using two restriction enzymes, SnaBI and NheI. The pGFAP promoter sequence (−1,817 to −17 upstream from the pGFAP transcription start site +1) in the pGL3-basic-pGFAP promoter plasmid [8] was digested with EcoRI (blunted by the Klenow fragment) and NheI. Subsequently, the pGFAP promoter sequence was ligated into the aforementioned CMV promoter-deleted lentiviral vector. Finally, to construct a lentiviral vector containing the pGFAP-CreERT2 gene construct, the CreERT2 gene digested by EcoRI from the pCre-ERT2 plasmid [19] was inserted into the identical restriction enzyme sites of pCDH-pGFAP-MCS-EF1-puro.
To generate the CMV-LoxP-EGFP-pA-LoxP construct, LCMV:EGFP(LoxP)MCS (No. 31377; Addgene, USA) was modified by removing the EGFP sequence by using NcoI and BsrGI. Then, the EGFP sequence from EGFP-C2 (No. 6083-1; Clontech Laboratories, USA) that had been digested with the same restriction enzymes was inserted into the aforementioned EGFP-deleted vector.
To produce the lentivirus, 293FT cells were plated on 100-mm plates in complete Dulbecco's modified Eagle medium (DMEM) with 10% fetal bovine serum (FBS; Gibco, Australia) and 2 mM L-glutamine (Lonza, Switzerland) and allowed to adhere for 12 h. Transfections were performed as per the manufacturer's instructions with 4 µg of transfer vector plasmid, 6 µg of third generation packaging plasmids, and 24 µL Polyexpress agent (Excellgene, USA) in serum-free DMEM (Invitrogen, USA). Six hours after transfection, the cells were washed with phosphate-buffered saline, after which, fresh complete DMEM with L-glutamine and 10% FBS was added, and the cells were incubated for an additional 42 h. Supernatant medium containing lentivirus was collected 48 h after transfection and filtered through a 0.45-µm syringe. Each lentivirus was concentrated with the Lenti-X Concentrator (Clontech Laboratories) according to the manufacturer's instructions.
The primary cell line was derived from male Yucatan miniature pig fetal fibroblast cells. The cell line was named Cloud male. It was transfected with LCMV-EGFPLoxP using an electroporation tool (Neon Transfection System; Invitrogen) according to the manufacturer's instructions. After 3 to 4 weeks, EGFP-positive cells were sorted by flow cytometry (fluorescence-activated cell sorter [FACS], Aria II; BD Biosciences, USA). The EGFP-positive cells were then infected with the pCDH-pGFAP-CreERT2-EF1-puro lentivirus vector by using polybrene (6 µg/mL). Three days after infection, cells were selected by puromycin treatment (2 µg/mL; Clontech Laboratories) for 5 days.
Oocyte collection and in vitro maturation was performed according to Hwang et al. [18]. Briefly, porcine ovaries were collected from a slaughterhouse. Porcine follicular fluid (pFF) and cumulus-oocyte complexes (COCs) were recovered from 3 to 6 mm ovarian follicles by aspiration. The composition of the medium used during in vitro maturation (IVM) was as follows: TCM199 (Gibco), 0.6 mM cysteine, 0.91 mM sodium pyruvate, 10 ng/mL EGF, 75 µg/mL kanamycin, 1 µg/mL insulin, and 10% (v/v) pFF. Porcine COCs were co-cultured at 50 to 60 cells per well in a 4-well dish (Nunc, Denmark) with 500 µL IVM media. The conditions for IVM were 39℃ in a 5% CO2 atmosphere in a humid incubator (Astec, Japan). Maturation was performed in IVM medium with 10 IU/mL equine chorionic gonadotropin and 10 IU/mL human CG for 22 h. The cells were then moved into hormone-free IVM medium and cultured for 18 h. Matured COCs were denuded by using gentle pipetting with 0.1% hyaluronidase and HEPES-buffered Tyrode's medium containing 0.05% (w/v) polyvinyl alcohol (TLH-PVA) medium. Denuded oocytes obtained through this process were used in subsequent experiments.
After 40 h of IVM, denuded oocytes at metaphase II (MII) stage were chosen for enucleation. MII oocytes were washed thrice in calcium-free TLH containing 0.2% bovine serum albumin (TLH-BSA) and 5 µg/mL cytochalasin B (CB). Enucleation was performed by using a micro-manipulator with a 16-mm glass pipette (Humagen, USA). After enucleation, trypsinized TG donor cells were transferred into the perivitelline space of enucleated oocytes. Next, they were fused by two pulses of 180 V/mm direct current for 60 µsec in a 260 mM mannitol solution containing 0.1 mM CaCl2 and 0.05 mM MgCl2 by using a cell fusion generator (LF201; Nepa Gene, Japan). After electrical fusion, the SCNT embryos were incubated in 6-dimethyl aminopurine with 5 µg/mL CB in 30-µL droplets of porcine zygote medium (PZM) for 4 h (post-activation). Embryos were transferred to PZM droplets for in vitro culture. On the second day after fusion, embryo cleavage was evaluated (1 cell, 2-3 cell, 4-5 cell, 6-8 cell stages and fragmented embryos), and embryos were transferred to new PZM droplets. On the 4th day, we transferred the embryos to PZM droplets containing 10% FBS. On the 7th day after fusion, blastocyst (BL) formation was evaluated quantitatively (early, expanded, hatched BL).
Non-superovulated, naturally cycling Landrace × Duroc crossbreed gilts were used as surrogate mothers. The ear veins were injected with 1 mL ketamine (50 mg/mL; Yuhan, Korea) and 3 mL xylazine (100 mg/mL; SF, Korea) for preanesthesia. Thereafter, respiratory anesthesia using isoflurane liquid (Hana Pharm, Korea) was maintained. All surgical instruments were sterilized before surgery. A midventral laparotomy was performed for exposure of the reproductive organs. At 4 h post-activation, SCNT embryos (100–150 embryos per recipient) were transferred into surrogate oviducts (at the ampullary-isthmic junction). After 30 days, ultrasonography was used to diagnose pregnancy.
In previous studies, CreERT2-mediated recombination in mice was induced by oral or intraperitoneal injection. However, injections by oral or intraperitoneal injection. Compared with oral administration, intraperitoneal injections require a 5 to 10 fold increase in concentration [11]. Therefore, we decided to induce CreERT2-mediated recombination in piglets by oral administration. Previously, the tamoxifen concentration in blood after oral administration of tamoxifen was reported in rats [22]. We established the concentration on the basis of this result. At 3 months of age, the piglet was orally administered 15 mg/kg TM for 5 days and then euthanized 7 days later.
Genomic DNA was isolated from frozen tissues and cells by using the Wizard genomic DNA purification kit (Promega, USA) according to the manufacturer's instructions. Large and tough tissues were homogenized by using a Bullet Blender (Next Advance, USA) according to the manufacturer's instructions. Transgenes in genomic DNA were amplified with the appropriate primer set (EGFP, CreERT2, or 18S rRNA) and NeoTherm DNA polymerase (Genecraft, Germany) under the following thermal cycling conditions: 60 sec at 95℃; 30 cycles of 30 sec at 95℃, 40 sec at 58℃, and 60 sec at 72℃; followed by 10 min at 72℃.
For semi-nested polymerase chain reaction (PCR) analysis to detect CreERT2-mediated recombination, first-round PCR was performed by using the first pair of primers (forward and reverse) and NeoTherm DNA polymerase under the following thermal cycling conditions: 60 sec at 95℃; 35 cycles of 30 sec at 95℃, 30 sec at 56℃, and 120 sec at 72℃; followed by 10 min at 72℃. All PCR products were separated by DNA electrophoresis in a 1% agarose gel. Gel blocks at the expected product size after recombination were removed without UV exposure. Then, in-gel DNA extraction in the isolated gel slices was performed by using a gel extraction kit (Elpis Biotech, Korea) according to the manufacturer's instructions. Finally, second-round PCR was performed by using forward and reverse primers under PCR conditions identical to those of the first-round PCR. The primer sequences are presented in Table 1.
Quantitative reverse-transcription PCR (qRT-PCR) was performed by using Takara SYBR Premix Ex Taq (Takara Bio, Japan) and CFX096 (Bio-Rad, USA) to determine mRNA levels. Total RNA was isolated from cells by using TRIzol reagent (Invitrogen) according to the manufacturer's instructions. RNase-free DNase-treated RNA (1 µg) was used as the template to synthesize cDNA by using the RevertAid first-strand cDNA synthesis kit (Thermo Fisher Scientific, USA) according to the manufacturer's instructions. The expression levels of genes of interest were normalized to that of 18S rRNA. All primer sequences are presented in Table 1.
Pig brain tissues embedded in paraffin were sliced into 4-µm-thick sections, deparaffinized, and rehydrated as follows: 3 changes of xylene (5 min each), 2 changes of 100% ethanol (3 min each), 95% ethanol (3 min), 80% ethanol (3 min), and 70% ethanol (3 min). Then, antigen retrieval was performed by using sodium citrate buffer (10 mM sodium citrate, 0.05% Tween-20, pH 6.0) followed by microwaving for 20 min. Pig brain tissues were stained with primary antibodies against pGFAP (691102, 1:500; MP Biomedical, USA), Cre recombinase (12830S, 1:200; Cell Signaling, USA), and EGFP (AB6673, 1:200 and AB290, 1:200; Abcam, UK) for 12 h at 4℃. This was followed by incubation with secondary antibodies conjugated with Alexa Fluor 488 or 594 (A11001, A11005, A11012, or A11055, all 1:500; Thermo Fisher Scientific) for 1 h at room temperature. Nuclei were then stained with 4′,6-diamidino-2-phenylindole (1 µg/mL) for 5 min. Fluorescence images were obtained by using a confocal laser scanning microscope (LSM 5 Pascal; Carl Zeiss Vision, Germany).
Experiments for statistical analysis were replicated more than three times. All data were analyzed by one-way ANOVA followed by Duncan's test as provided in IBM SPSS Statistics software (ver. 21; IBM, USA). Mean ± SEM values were obtained. Differences were considered significant at p < 0.05.
Before constructing a pig astrocyte-specific recombination system, we tested whether the CreERT2/loxP system worked in pig fibroblasts by detecting EGFP-floxed populations after TM-mediated CreERT2 activation (Fig. 1). Subsequently, we constructed an astrocyte-specific pGFAP-CreERT2/EGFPLoxP recombination vector for developing TG pigs. Using these vectors, we attempted to construct nuclear donor fibroblast cells with pGFAP-CreERT2/EGFPLoxP transgene constructs. Cells expressing the EGFP transgene were sorted by FACS, and EGFP expression was validated by fluorescence microscopy (Fig. 2). Next, we infected the pGFAP-CreERT2-puro lentiviral vector into cells expressing the EGFP transgene, and three cell colonies were established as final donor cells for SCNT by using antibiotic selection. The presence of each transgene into the pig fibroblast genome was identified by performing genomic DNA PCR analysis (panel D in Fig. 2). In the same manner, we constructed nuclear donor fibroblasts with an astrocyte-specific CreERT2/LoxP recombination system by using two vectors and confirmed the presence of each transgene of the system in the genomic DNA.
The development of SCNT embryos was evaluated in order to assess the normality of the TG donor cell line (Fig. 3). Cloud male No. 5 (primary cell line from a normal fetal Yucatan minipig), and Cloud male No. 5 pGFAP-CreERT2 + LCMV-EGFPLoxP (vector-inserted cell line) cells were used as the donor cells. The fusion rates (71.8 ± 6.1 and 74.2 ± 2.6, respectively) and cleavage rates (73.8 ± 4.0 and 63.6 ± 2.2, respectively) did not show significant differences. The BL formation rate was not significantly different between the Cloud male No. 5 and Cloud male No. 5 pGFAP-CreERT2 + LCMV-EGFPLoxP cells (19.5 ± 1.2 and 21.0 ± 1.6, respectively). These findings indicate that the foreign genes in the donor cells did not affect the development of SCNT embryos.
Embryo transfer was performed thrice just before the onset of ovulation of the surrogate mothers (panel A in Fig. 4). One of the surrogates became pregnant and delivered 5 TG piglets at 115 days after pregnancy. Three piglets (No. 1, 2, and 3) were stillborn, No. 5 died after 22 days, and only No. 4 survived. Genomic DNA analysis in skin cells confirmed that all 5 piglets had the pGFAP-CreERT2/EGFPLoxP transgene constructs (panels B and C in Fig. 4). Further diagnosis of stillbirths (No. 1, 2, and 3) was not performed. After 3 months, the surviving piglet was orally administered 15 mg/kg TM for 5 days and then euthanized 7 days later.
To determine whether the transgene controlled by the pGFAP promoter sequence was expressed specifically in astrocytic lineages, tissues from 4 TG piglets, including two spontaneously aborted TG piglets (No. 2 and 3), were analyzed by qRT-PCR. The carcass of TG pig No. 1 was unsuitable for the experiments because of inappropriate storage; therefore, it was excluded.
Endogenous GFAP expression was primarily restricted to brain tissues, and the CreERT2 gene was also highly expressed in brain tissues compared to that in other tissues (panel A in Fig. 5). Immunofluorescence (IF) staining was performed to determine whether CreERT2 controlled by the pGFAP promoter was expressed in GFAP-positive glial cells. CreERT2 expression was detected not only in star-shaped GFAP-positive cells but also in some other cell types (panel B in Fig. 5). To determine whether the pGFAP promoter transcribed the CreERT2 transgene in non-astrocytic cells, fibroblasts obtained from the ear tissues of aborted TG pigs No. 2 and 3 were assessed by primary culture. The cell lines were treated with TM. We observed that CreERT2 controlled by the pGFAP promoter was not expressed in these cells (Fig. 6). Furthermore, EGFP transgene expression in brain tissues was detected by IF staining (panel C in Fig. 5). Although GFAP-positive cells exhibited a slightly weaker fluorescence intensity than other cell types in brain tissue, most of the cells expressed EGFP. These data indicate that the pGFAP-CreERT2/EGFPLoxP developed in this study can be used as an astrocyte-specific recombination system.
We next administered TM to TG pig No. 4 for 5 days and sacrificed it after one week to determine whether the pig astrocyte-specific pGFAP-CreERT2/EGFPLoxP recombination system was present (panel A in Fig. 7). We first used IF staining to compare the EGFP signal intensity in brain tissues of TM-treated TG pig No. 4 with that of untreated TG pig No. 5, which had died 22 days after birth and was used as a negative control for CreERT2-mediated recombination. Astrocytic cells without EGFP expression were mainly detected in the brain of pig No. 4 (panel B in Fig. 7).
We also conducted genomic DNA PCR analysis by amplifying the recombination site using forward and reverse primer pairs flanking the two LoxP sites. PCR products amplifying pre-recombinant DNA were only detected in brain and skin tissues (panel C in Fig. 7). We attributed the invisible post-recombinant PCR products in the PCR analysis to the presence of a very small population of recombined cells. Therefore, we used a semi-nested PCR analysis to increase PCR sensitivity. After first-round amplification, second-round PCR was performed with the first-round PCR product and a primer set that enabled amplification of an internal sequence of the first-round PCR product (panel D in Fig. 7). The presence of a 450-base pair PCR product indicated that post-recombination occurred only in the brain of the TM-treated pig (panel E in Fig. 7). These data indicate that pGFAP-CreERT2-driven recombination occurs in a very small population of cerebral astrocytic cells in response to TM treatment.
The production and characterization process for pig models of human neurological disease is more expensive and time-consuming than that for mouse models [15]. The purpose of this study was to generate TG piglets using an astrocyte-specific pGFAP-CreERT2-inducible system and to confirm the effectiveness of the system in vivo.
TM-inducible recombination systems using the pGFAP promoter sequence have been widely used in astroglia-related studies requiring conditional turning on or off of transgenes. This is an important tool for the generation of various TG models, such as brain tumor models. In a previous study, we isolated a 5′-flanking sequence of pGFAP from the Yucatan miniature pig genome and verified its function as an astrocyte-specific promoter in vitro [8]. In the present study, we introduced an astrocyte-specific pGFAP-CreERT2 inducible system to prevent stillbirth and premature death caused by the expression of transgenes and the neural-specific pGFAP promoter. The hGFAP promoter has been widely used to produce mouse models for central nervous system (CNS) diseases [14].
Techniques for the production of TG pigs include SCNT by micromanipulation, handmade cloning (a simple variant of SCNT technology without micromanipulation), and microinjection, among others [63437]. Herein, we constructed an astrocyte-specific conditional pGFAP-CreERT2/EGFPLoxP recombination system and evaluated its validity in TG pig embryos and assessed the pig model generated by SCNT technology.
In this study, the production rate of TG pigs was approximately 1.9% (5 piglets/269 SCNT embryos). At present, the production efficiency of TG pigs is very low worldwide. When calculated based on birth weight, the efficiency is 1% to 2%; however, a production rate of 5.5% (28 piglets/511 SCNT embryos) was reported by Walker et al. [38]. There are several reasons for the low pregnancy and production rates. First, the current TG techniques used in the TG embryo creation process, such as IVM of oocytes and somatic cell treatment processing, are incomplete. Second, the low developmental potential of TG embryos and the physiological characteristics of pigs necessitate the implantation of at least 3 to 4 embryos [38]. Finally, transgenes have been reported to negatively affect embryonic and fetal development [3539]. Our present astrocyte-specific pGFAP-CreERT2 inducible system demonstrated no deleterious effect on the rate of embryo development in vitro. In this study, we produced SCNT embryos using a Cloud male No. 5 pGFAP-CreERT2 + LCMV-EGFPLoxP donor cell line that was verified in vitro. The embryos were transferred into a surrogate pig that gave birth to five pGFAP-CreERT2/EGFPLoxP TG piglets. In qRT-PCR results, we detected relatively high levels of GFAP and CreERT2 expression in the cerebellum relative to that in the cerebrum. This result might be attributed to the GFAP-expressing Bergmann glial cells, which are a major cell type in the cerebellum [5]. IF staining and semi-nested PCR analysis showed that the CreER-mediated astrocyte-specific recombination system was functional in some cerebral astrocytes after TM administration to TG pig No. 4. However, in this process, some technical limitations related to low recombination efficiency occurred. First, despite its cerebral-specific expression, CreERT2 regulated by the pGFAP promoter showed low expression compared to that of endogenous GFAP expression in the brain. Previously, in a TG mouse model, an intron sequence of mouse protamine-1 was added into the 3′ region of the Gfa2 transgene [2]. Therefore, in the TG pig model, it might also be necessary to introduce a transcription-enhancing element, such as an intron(s) or enhancer(s), to increase transgene expression. Second, EGFP transgene silencing was detected in TG pigs. The transcriptional activity of the CMV promoter becomes progressively weaker during long-term cell culture or the reprogramming process through promoter methylation. Kong et al. [24] reported that methylation of the CMV promoter caused a gradual decrease of GFP expression in TG pigs and pig fibroblasts. Therefore, the addition of stably expressing promoters such as elongation factor 1α or CMV early enhancer/chicken β-actin promoter should be considered [13]. Third, unstable expression and transgene silencing might result from positional effects of random integration [27]. The use of a homologous recombination-based locus-targeting knock-in system to integrate the transgene into a safe harbor locus, such as Rosa26, could be an effective alternative strategy [2829]. Finally, given that the turning off of the reporter expression in target cells was almost impossible to clearly distinguish and quantify, an effective dual fluorescence reporter system should be applied [28].
In summary, we showed the successful generation of a pGFAP promoter-driven, TM-inducible CreERT2-LoxP recombination system in a TG pig generated by SCNT. To our knowledge, this is the first report of an astrocyte-specific pGFAP-CreERT2 inducible system in TG pigs. Therefore, we suggest that this technology might be a useful tool for studying the physiology of astrocytes and generating TG pig models of CNS diseases such as Huntington's disease, Alzheimer's disease, and brain tumors.
Figures and Tables
Fig. 1
Validation of CreERT2-mediated recombination in pig fibroblasts after 4-hydroxytamoxifen (TM) administration. (A) To test the recombination system, a fibroblast cell line with the CMV-EGFPLoxP construct was transiently transfected with the CreERT2 expression vector by using electroporation and, 24 h after transfection, treated with TM at each indicated concentration for 48 h. EGFP expression of each sample was determined by fluorescence-activated cell sorter analysis. The numbers indicate the percentage of the EGFP-negative population. Puro; puromycin. (B) To examine transient transfection efficiency, a red fluorescent protein (RFP) expression vector was transfected by same method, and its signal was detected by using fluorescence-activated cell sorter (FACS). Approximately 25% of the cells were RFP-positive. (C) When the floxed-population of each sample shown in (A) was normalized to the transfection efficiency shown in (B), approximately 21% of cells were recombined by CreERT2 when activated by TM treatment. Data are presented as mean ± SEM. *p<0.05 between Puro and CreERT2.
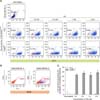
Fig. 2
Establishment of a somatic cell nuclear transfer (SCNT) donor cell line with the CreER-mediated astrocyte-specific recombination system. (A) Strategy for production of transgenic (TG) pig CreERT2-mediated astrocyte-specific recombination system by SCNT and its validation. TM, 4-hydroxytamoxifen. (B) Donor cell lines transfected with LCMV-EGFPLoxP plasmid vector were sorted by using a fluorescence-activated cell sorter (FACS). (C) Morphology of Cloud male No. 5 pGFAP-CreERT2+LCMV-EGFPLoxP donor cells; their EGFP expression was detected by using microscopy (200×). (D) The presence of system vectors in the genome was identified by polymerase chain reaction amplification of two transgenes in genomic DNA samples of constructed donor cell lines. 18s was used as a control.
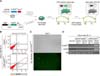
Fig. 3
Effect of different donor cell types during preimplantation development (A) on the cleavage pattern and (B) the blastocyst formation pattern of somatic cell nuclear transfer (SCNT) embryos. BL, blastocyst. (C) Summary of embryonic development after SCNT. The cleavage rate was measured on day 2, and blastocyst formation rate was evaluated on day 7 of culture. Values with different superscript letters within a column differ significantly (p < 0.05). Experiment was repeated more than three times. The data presented are means ± SEM. *Percentage of total oocytes. †Percentage of total fused oocytes.
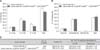
Fig. 4
Production of transgenic (TG) piglets with pGFAP-CreERT2/EGFPLoxP transgene constructs. (A) Summary of embryo transfer result. J.B.O., just before ovulation. (B) Picture of pGFAP-CreERT2/EGFPLoxP TG piglets. (C) Polymerase chain reaction was performed using genomic DNA isolated from skin tissue of somatic cell nuclear transfer piglets. 18s was amplified as a control. Normal, newborn normal Yucatan piglet tissue.
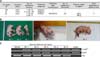
Fig. 5
Confirmation of cerebral expression of EGFP and CreERT2 transgenes in the astrocyte-specific CreERT2-LoxP recombination system. (A) Expression of endogenous GFAP and CreERT2 regulated by the exogenous GFAP promoter was confirmed by quantitative polymerase chain reaction analysis. Asterisks indicate significant difference (*p < 0.05) from non-brain tissues. (B) CreERT2 expression in GFAP-positive astrocytes and (C) cerebral expression of the EGFP gene in brain tissues was detected by immunofluorescence staining. Normal, newborn normal Yucatan piglet tissue; GFAP, glial fibrillary acidic protein; EGFP, enhanced green fluorescent protein; DAPI, 4′,6-diamidino-2-phenylindol.
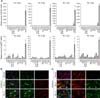
Fig. 6
Confirmation of pGFAP promoter activity in fibroblasts. (A) Experimental scheme showing expected pre-recombinant and post-recombinant sizes of genomic DNA polymerase chain reaction (PCR) products as amplified using the F and R primer set. (B) Genomic DNA PCR analysis revealed that 4-hydroxytamoxifen (4OH-TM) treatment in fibroblast cells (derived from the No. 2 and No. 3 transgenic pigs) for 48 h did not induce pGFAP-CreERT2-mediated recombination of LoxP sites. Con, control.
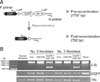
Fig. 7
Induction of CreER-mediated astrocyte-specific recombination system. (A) Scheme of 4-hydroxytamoxifen (4OH-TM) administration. (B) Result of No. 4 pig and control No. 5 pig cerebellum tissue Immunofluorescent staining. No. 4-cerebellum : TM-administrated pig, No. 5-cerebellum : TM nontreatment control. (C) Genomic DNA polymerase chain reaction (PCR) analysis showed that pre-recombinant PCR products were amplified in the cerebral cortex (upper) and cerebellum tissues (bottom) of pGFAP-CreERT2/EGFPLoxP transgenic pigs. Skin-derived genomic DNA was used as a control, representing non-cerebral tissue. (D) Scheme of Semi-nested PCR. After first-round PCR using the F and R primer set, an invisible target PCR product of around 702 bp size was extracted from agarose gel after electrophoresis. Then, a second PCR was performed using F and R′ primers. (E) Detection of recombinant genomic DNA by Semi-nested PCR. ‘C’ means a control and indicates a semi-nested PCR product from the plasmid vector, while ‘V’ represents a single PCR amplification product from thevector.
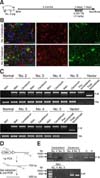
Acknowledgments
This work was supported, in part, by a grant from the “the Next-Generation BioGreen 21 Program (project Nos. PJ011288 and PJ011077)” Rural Development Administration, the “National Research Foundation of Korea Grant funded by the Korean Government (NRF-2016R1D1A1B03933191 and NRF-2017R1A2B4002546)”, the “Korea Institute of Planning and Evaluation for Technology in Food, Agriculture, Forestry and Fisheries (IPET) through Advanced Production Technology Development Program, funded by Ministry of Agriculture, Food and Rural Affairs (MAFRA) (grant No. 115103-02)”, the Global Research and Development Center (GRDC) Program through the National Research Foundation of Korea (NRF) funded by the Ministry of Education, Science and Technology (2017K1A4A3014959), and the Business for Cooperative R&D between Industry, Academy, and Research Institute funded by Korea Small and Medium Business Administration in 2017 (grant No. 2017020681010101), Republic of Korea.
References
1. Bradford J, Shin JY, Roberts M, Wang CE, Li XJ, Li S. Expression of mutant huntingtin in mouse brain astrocytes causes age-dependent neurological symptoms. Proc Natl Acad Sci U S A. 2009; 106:22480–22485.


2. Brenner M, Kisseberth WC, Su Y, Besnard F, Messing A. GFAP promoter directs astrocyte-specific expression in transgenic mice. J Neurosci. 1994; 14:1030–1037.


3. Danielian PS, Muccino D, Rowitch DH, Michael SK, McMahon AP. Modification of gene activity in mouse embryos in utero by a tamoxifen-inducible form of Cre recombinase. Curr Biol. 1998; 8:1323–1326.


4. Daschil N, Humpel C. Green-fluorescent protein+ astrocytes attach to beta-amyloid plaques in an Alzheimer mouse model and are sensitive for clasmatodendrosis. Front Aging Neurosci. 2016; 8:75.


5. de Blas AL. Monoclonal antibodies to specific astroglial and neuronal antigens reveal the cytoarchitecture of the Bergmann glia fibers in the cerebellum. J Neurosci. 1984; 4:265–273.


6. Du Y, Kragh PM, Zhang Y, Li J, Schmidt M, Bøgh IB, Zhang X, Purup S, Jørgensen AL, Pedersen AM, Villemoes K, Yang H, Bolund L, Vajta G. Piglets born from handmade cloning, an innovative cloning method without micromanipulation. Theriogenology. 2007; 68:1104–1110.


7. Eng LF. Glial fibrillary acidic protein (GFAP): the major protein of glial intermediate filaments in differentiated astrocytes. J Neuroimmunol. 1985; 8:203–214.


8. Eun K, Hwang SU, Jeon HM, Hyun SH, Kim H. Comparative analysis of human, mouse, and pig glial fibrillary acidic protein gene structures. Anim Biotechnol. 2016; 27:126–132.


9. Feil R, Brocard J, Mascrez B, LeMeur M, Metzger D, Chambon P. Ligand-activated site-specific recombination in mice. Proc Natl Acad Sci U S A. 1996; 93:10887–10890.


10. Feil R, Wagner J, Metzger D, Chambon P. Regulation of Cre recombinase activity by mutated estrogen receptor ligand-binding domains. Biochem Biophys Res Commun. 1997; 237:752–757.


12. Grindley ND, Whiteson KL, Rice PA. Mechanisms of site-specific recombination. Annu Rev Biochem. 2006; 75:567–605.


13. Herbst F, Ball CR, Tuorto F, Nowrouzi A, Wang W, Zavidij O, Dieter SM, Fessler S, van der Hoeven F, Kloz U, Lyko F, Schmidt M, von Kalle C, Glimm H. Extensive methylation of promoter sequences silences lentiviral transgene expression during stem cell differentiation in vivo. Mol Ther. 2012; 20:1014–1021.


14. Herculano-Houzel S. The human brain in numbers: a linearly scaled-up primate brain. Front Hum Neurosci. 2009; 3:31.


15. Holm IE, Alstrup AK, Luo Y. Genetically modified pig models for neurodegenerative disorders. J Pathol. 2016; 238:267–287.


16. Howells DW, Porritt MJ, Rewell SS, O'Collins V, Sena ES, van der Worp HB, Traystman RJ, Macleod MR. Different strokes for different folks: the rich diversity of animal models of focal cerebral ischemia. J Cereb Blood Flow Metab. 2010; 30:1412–1431.


17. Huszthy PC, Daphu I, Niclou SP, Stieber D, Nigro JM, Sakariassen PØ, Miletic H, Thorsen F, Bjerkvig R. In vivo models of primary brain tumors: pitfalls and perspectives. Neuro Oncol. 2012; 14:979–993.


18. Hwang SU, Jeon Y, Yoon JD, Cai L, Kim E, Yoo H, Kim KJ, Park KM, Jin M, Kim H, Hyun SH. Effect of ganglioside GT1b on the in vitro maturation of porcine oocytes and embryonic development. J Reprod Dev. 2015; 61:549–557.


19. Indra AK, Warot X, Brocard J, Bornert JM, Xiao JH, Chambon P, Metzger D. Temporally-controlled site-specific mutagenesis in the basal layer of the epidermis: comparison of the recombinase activity of the tamoxifen-inducible Cre-ERT and Cre-ERT2 recombinases. Nucleic Acids Res. 1999; 27:4324–4327.


20. Jin YX, Jeon Y, Lee SH, Kwon MS, Kim T, Cui XS, Hyun SH, Kim NH. Production of pigs expressing a transgene under the control of a tetracycline-inducible system. PLoS One. 2014; 9:e86146.


21. Kararli TT. Comparison of the gastrointestinal anatomy, physiology, and biochemistry of humans and commonly used laboratory animals. Biopharm Drug Dispos. 1995; 16:351–380.


22. Kim CS, Choi SJ, Park CY, Li C, Choi JS. Effects of silybinin on the pharmacokinetics of tamoxifen and its active metabolite, 4-hydroxytamoxifen in rats. Anticancer Res. 2010; 30:79–85.
23. King TJ, Dobrinsky JR, Zhu J, Finlayson HA, Bosma W, Harkness L, Ritchie WA, Travers A, McCorquodale C, Day BN, Dinnyés A, De Sousa PA, Wilmut I. Embryo development and establishment of pregnancy after embryo transfer in pigs: coping with limitations in the availability of viable embryos. Reproduction. 2002; 123:507–515.


24. Kong Q, Wu M, Huan Y, Zhang L, Liu H, Bou G, Luo Y, Mu Y, Liu Z. Transgene expression is associated with copy number and cytomegalovirus promoter methylation in transgenic pigs. PLoS One. 2009; 4:e6679.


25. Kues WA, Schwinzer R, Wirth D, Verhoeyen E, Lemme E, Herrmann D, Barg-Kues B, Hauser H, Wonigeit K, Niemann H. Epigenetic silencing and tissue independent expression of a novel tetracycline inducible system in double-transgenic pigs. FASEB J. 2006; 20:1200–1202.


26. Le Y, Sauer B. Conditional gene knockout using Cre recombinase. Mol Biotechnol. 2001; 17:269–275.


27. Levis R, Hazelrigg T, Rubin GM. Effects of genomic position on the expression of transduced copies of the white gene of Drosophila. Science. 1985; 229:558–561.


28. Li S, Flisikowska T, Kurome M, Zakhartchenko V, Kessler B, Saur D, Kind A, Wolf E, Flisikowski K, Schnieke A. Dual fluorescent reporter pig for Cre recombination: transgene placement at the ROSA26 locus. PLoS One. 2014; 9:e102455.
29. Li X, Yang Y, Bu L, Guo X, Tang C, Song J, Fan N, Zhao B, Ouyang Z, Liu Z, Zhao Y, Yi X, Quan L, Liu S, Yang Z, Ouyang H, Chen YE, Wang Z, Lai L. Rosa26-targeted swine models for stable gene over-expression and Cre-mediated lineage tracing. Cell Res. 2014; 24:501–504.


30. Metzger D, Clifford J, Chiba H, Chambon P. Conditional site-specific recombination in mammalian cells using a ligand-dependent chimeric Cre recombinase. Proc Natl Acad Sci U S A. 1995; 92:6991–6995.


32. Niemann H, Tian XC, King WA, Lee RS. Epigenetic reprogramming in embryonic and foetal development upon somatic cell nuclear transfer cloning. Reproduction. 2008; 135:151–163.


33. No D, Yao TP, Evans RM. Ecdysone-inducible gene expression in mammalian cells and transgenic mice. Proc Natl Acad Sci U S A. 1996; 93:3346–3351.


34. Ogura A, Inoue K, Wakayama T. Recent advancements in cloning by somatic cell nuclear transfer. Philos Trans R Soc Lond B Biol Sci. 2013; 368:20110329.


35. Orian JM, Lee CS, Weiss LM, Brandon MR. The expression of a metallothionein-ovine growth hormone fusion gene in transgenic mice does not impair fertility but results in pathological lesions in the liver. Endocrinology. 1989; 124:455–463.


36. Schuurman HJ, Pierson RN 3rd. Progress towards clinical xenotransplantation. Front Biosci. 2008; 13:204–220.


37. Uchida M, Shimatsu Y, Onoe K, Matsuyama N, Niki R, Ikeda JE, Imai H. Production of transgenic miniature pigs by pronuclear microinjection. Transgenic Res. 2001; 10:577–582.