Abstract
Bovine abortion, diarrhea, and respiratory disease complexes, caused by infectious agents, result in high and significant economic losses for the cattle industry. These pathogens are likely transmitted by various vectors and reservoirs including insects, birds, and rodents. However, experimental data supporting this possibility are scarce. We collected 117 samples and screened them for 44 bovine abortive, diarrheal, and respiratory disease complex pathogens by using Dembo polymerase chain reaction (PCR), which is based on TaqMan real-time PCR. Fifty-seven samples were positive for at least one pathogen, including bovine viral diarrhea virus, bovine enterovirus, Salmonella enterica ser. Dublin, Salmonella enterica ser. Typhimurium, and Neospora caninum; some samples were positive for multiple pathogens. Bovine viral diarrhea virus and bovine enterovirus were the most frequently detected pathogens, especially in flies, suggesting an important role of flies in the transmission of these viruses. Additionally, we detected the N. caninum genome from a cockroach sample for the first time. Our data suggest that insects (particularly flies), birds, and rodents are potential vectors and reservoirs of abortion, diarrhea, and respiratory infectious agents, and that they may transmit more than one pathogen at the same time.
Abortion, diarrhea, and respiratory infectious agents cause a broad spectrum of diseases, resulting in significant economic losses in the cattle industry [6919]. In the USA, it has been estimated that late-term cattle abortions cost between US dollar (USD) 500 and USD 900 per case and that the cattle respiratory disease complex causes 70% to 80% of all feedlot cattle morbidity and 40% to 50% of all cattle mortality, resulting in major economic losses amounting to more than USD 500 million per year [1019]. Diarrhea in cattle decreases fertility and productivity, including reductions in milk production and weight gain [911]. According to the United States Department of Agriculture's reports, 57% of deaths of weaning calves in the USA were due to diarrhea [31].
Various vectors and reservoirs have important roles in the transmission of pathogens [42227]. Vector-borne diseases are transmitted by insects, such as mosquitoes, flies, ticks, fleas, and lice [2227]. Vectors may be divided into two types: biological and mechanical. Biological vectors carry infectious agents or pathogens within their bodies, where the infectious agents undergo multiplication and/or development, consequently transmitting the infectious agents to the host through bites. Mosquitoes are a biological vector of many pathogens. Mechanical vectors transfer pathogens from an infected host or a contaminated substrate to a susceptible host without multiplying and/or developing of the pathogens within the vector. Many insects can serve as mechanical vectors [27]. Reservoirs are one or more epidemiologically connected populations or environments, in which the infectious agent can be permanently maintained, and from which infection is transmitted to the defined target population [8]. Mammals, such as rodents and carnivores, are examples of most commonly known disease reservoirs [8].
Several studies have shown that vectors and reservoirs play a critical role in the transmission of a broad spectrum of pathogens, including bovine viral diarrhea virus (BVDV), bovine enterovirus (BEV), Salmonella enterica ser. Typhimurium, Escherichia coli, and Campylobacter spp. [372227].
Previously, we developed detection systems for 19 bovine diarrheal agents and 16 bovine respiratory disease complexes by using a detection system of microbes for bovine (Dembo) diarrheal diseases via real-time polymerase chain reaction (Dembo diarrhea-PCR) and a similar system for bovine respiratory disease complex via real-time PCR (Dembo respiratory-PCR), respectively; both detection systems are based on TaqMan real-time PCR [1331]. The Dembo-PCR method exhibits high sensitivity, high specificity, rapidity, and a capacity to simultaneously detect all targeted infectious agents.
In the present study, we developed a real-time PCR-based system for detection of 24 bovine abortive agents (Dembo abortion-PCR). Subsequently, by using Dembo-PCR, we evaluated whether infectious agents causing diseases in cattle could be transferred by vectors and reservoirs such as insects, rodents, and birds.
We selected 24 pathogens as bovine abortive agents. To detect 15 of those pathogens, we used previously reported primers and probes [1820232425282931323336]. We used newly designed primers and probes for the remaining 9 pathogens: Schmallenberg virus, Chuzan virus, Sathuperi virus, Shamonda virus, Douglas virus, Ibaraki virus, Aino virus, Toxoplasma gondii, and Neospora caninum. The multiple nucleotide sequences for each of these 9 pathogens were obtained from the National Center for Biotechnology Information database (Supplementary Table 1), and new sets of primers and probes were designed by using PrimerQuest tool (Integrated DNA Technologies, USA) based upon a consensus sequence acquired by multiple alignments of the obtained sequences obtained via the BioEdit software 7.0.5 (Ibis Therapeutics, USA). Bovine β-actin was used as the internal control for the extraction of nucleic acids [133134]. All probes at the 5′ end were indicated by the dye FAM (6-carboxyfluorescein) and by the fluorescent dye TAMRA (6-carboxytetramethylrhodamine) at the 3′ end. All primers and probes were purchased from Sigma-Aldrich (USA) or Integrated DNA Technologies. Table 1 lists the primers and probes used in this study.
Viral nucleic acids were extracted by using High Pure Viral Nucleic Acid Kit (Roche Diagnostics, Germany). The QIAamp Fast DNA Stool Mini Kit (Qiagen, Germany) was used according to the manufacturer's instructions to obtain bacterial, protozoal, and fungal DNA. The extracted DNA and RNA were stored at −80℃ until use.
All TaqMan real-time PCR assays were performed under the same reaction conditions used for Dembo diarrhea-PCR and Dembo respiratory-PCR [1331]. A One Step PrimeScript RT-PCR Kit (Perfect Real Time; TaKaRa Bio, Japan) was used to detect viral RNA, and Premix Ex Taq (Perfect Real Time) was used to detect the viral, protozoal, fungal, and bacterial DNAs. The real-time PCR assay was performed with the Applied Biosystems 7300 Real-Time PCR System (ABI 7300; Applied Biosystems, USA) for screening and with the LightCycler Nano (Roche Diagnostics) for validation of positive samples detected during screening. To analyze the fluorescence data, the automatic quantification algorithm was used in LightCycler Nano Software 1.1 and Applied Biosystems 7300 Real-Time PCR software. The parameters of analysis were as follows: Exclude early cycle = 7, minimum relative amplifications = 0, and minimum amplification quality = 5.
To evaluate the sensitivity of the Dembo abortion-PCR, the synthetic DNA (including target genome regions) of all target pathogens was used to determine the limit of detection (LOD), correlation coefficient (R2), and PCR efficiency (E). The synthetic DNA was fabricated at Integrated DNA Technologies. After creation of standard curves, LOD, R2, and E were calculated as described previously [1331]. To validate the specificity of the Dembo abortion-PCR, 22 cattle blood samples and 14 cattle aborted fetus spinal cord samples were collected and subjected to Dembo abortion-PCR. All samples were negative for the presence of target pathogens; no positive or false positive results were obtained.
A total of 117 vector and reservoir samples, including 63 flies, 18 gadflies, 7 insects, 14 fecal and intestinal contents from rodents, and 14 fecal samples from birds were collected from inside and outside of 4 dairy cattle farms and 17 beef cattle farms between 2014 to 2016 in Japan (Supplementary Table 2). Nucleic acids were extracted from each sample. To identify bovine diarrheal pathogens, all 117 samples were screened individually. To detect bovine abortive and respiratory disease complex pathogens, extracted nucleic acid samples were pooled as shown in Table 2. After sample pooling, RNAs in each pooled sample were reverse transcribed into complementary DNA (cDNA) by using SuperScript III Reverse Transcriptase (Invitrogen, USA), and then, the cDNA and genomic DNA were amplified by using GenomiPhi V2 DNA Amplification Kit (GE Healthcare, UK). The extracted nucleic acids were evaluated in triplicate by targeting abortive, diarrheal, and respiratory disease complex pathogens in a single run of Dembo-PCR [31]. When the Cq values were calculated by algorithm described above in more than two out of three runs, the samples were considered positive.
Table 3 shows the LOD, R2, and E value of the Dembo abortion-PCR results from the LightCycler Nano instrument. The LOD, according to the DNA copy number of all pathogens, was between 1 and 100 copies per reaction. The coverage of the calibration curves for each assay was within a linear dynamic range of more than five orders of magnitude, and R2 values were at least 0.9582. The E values were in the range of 92.1% to 106%. When using the ABI 7300 instrument, the LODs of the Dembo respiratory- and abortion-PCR assays were evaluated with 100 copies per reaction. When the sensitivity was lower than 100 copies per reaction, lower diluents were used to evaluate the LOD. All sets of primers and probes, except for 5, showed sensitivities of 100 copies per reaction (Table 4).
Field samples were analyzed via two different approaches. To detect diarrheal pathogens, all 117 samples were individually screened via Dembo-PCR using the LightCycler Nano instrument. Fifty-seven of the 117 samples were positive for at least one diarrheal pathogen; 34 of the 63 flies (53.97%), 8 of the 14 fecal and intestinal contents from rodents (57.14%), 8 of the 18 gadflies (44.44%), 5 of the 14 fecal samples from birds (35.71%), and 2 of the 7 insects (28.57%) were positive for at least one pathogen including BVDV, BEV, S. enterica ser. Dublin, and S. enterica ser. Typhimurium. To detect abortive and respiratory disease complex pathogens, 15 pooled samples were screened via Dembo-PCR using the ABI 7300 instrument. N. caninum was detected only in an insect pooled sample, which consisted of 7 different insect samples including 2 cockroaches, 2 spiders, and 3 unidentified insects. All other pathogen results were negative in all of the pooled samples. To determine which insects were positive for N. caninum, each of the 7 insect samples was analyzed using the LightCycler Nano instrument, resulting in the cockroach sample testing positive exclusively. Table 5 summarizes the numbers of positive samples from each vector and reservoir.
This is the first study that simultaneously evaluated the presence of a wide range of bovine abortion pathogens in potential vectors and reservoirs by using the Dembo abortion-PCR, a highly sensitive and rapid pathogen detection system. The Dembo abortion-PCR was performed by using the same reaction conditions as those reported for Dembo diarrhea-PCR and Dembo respiratory-PCR [1331]. We first used the Dembo abortion-PCR to detect 24 cattle abortive agents including 11 viruses, 8 bacteria, 4 protozoa, and 1 fungus. Subsequently, 44 bovine abortive, diarrheal, and respiratory disease complex pathogens, including 23 viruses, 12 bacteria, 6 protozoa, 2 mycoplasmas, and 1 fungus, were targeted in a single run by Dembo-PCR. For the Dembo abortion-PCR, additional primers and probes were designed to detect the Schmallenberg virus, Sathuperi virus, Douglas virus, and Shamonda virus [35]. These viruses belong to the Simbu group, which includes important viruses causing abortion in cattle [35].
BVDV was one of the most frequently detected pathogens from the wide spectrum of examined vectors and reservoirs, including flies, gadflies, and rodent and bird fecal matter. It should be noted that in addition to diarrhea, BVDV causes abortion and respiratory diseases [131731]. Out of the 117 vector and reservoir samples, 20 tested positive for BVDV, and 8 flies that were positive for BVDV formed the largest group among the examined vectors and reservoirs. Our results are consistent with those in a previous study that reported flies as a potential source of BVDV transmission in cattle [3]. We also detected BVDV in rodent and avian fecal matter; in contrast, no previous study has reported the presence of BVDV in rodents and birds. The results of these studies (both current and past [1331]) imply that BVDV is one of the most important infectious agents in cattle-related diseases and that BVDV could be transmitted by flies, rodents, and birds.
BEV was positive in 34 samples, including 28 flies, 2 gadflies, 1 rodent fecal matter sample, 2 avian fecal matter samples, and 1 cockroach. BEV, a common virus in the environment, is very stable under a broad range of environmental conditions such as pH, temperature, and salinity. These physiological properties of BEV facilitate easy transmission of BEV to cattle [15]. Although BEV was detected from diarrheal samples in this study, it also has the potential to cause abortion in cattle [31]. While previous studies have mentioned that BEV could be spread in the environment and contaminate water and food, our present study represents the first detection of BEV in vectors and reservoirs [12].
Both S. enterica ser. Dublin and S. enterica ser. Typhimurium were detected in flies, gadflies, and fecal samples of rodents and birds, suggesting that those animals serve as reservoirs [1212227]. In cattle, these two serovars of Salmonella cause diarrhea and may also cause abortion and respiratory disease [21331]. In this study, we did not demonstrate whether cattle inside the farms were infected with these pathogens. Further study is needed to isolate these bacteria from potential vectors and reservoirs because we detected only the bacterial genomes in this study; additionally, there is a need to investigate transmission from these pests to cattle.
N. caninum and BEV were simultaneously detected in one cockroach sample. N. caninum is an obligate intracellular coccidian parasite that is globally distributed and is one of the major pathogens causing abortion in cattle [1630]. A broad spectrum of wild and domestic animals can be infected by N. caninum with dogs, coyotes, and gray wolves (Canis lupus) considered to be final hosts of N. caninum. However, mammals and birds, including cattle, sheep, goat, water buffalo, horse, donkey, bison, white-tailed deer, red fox, chicken, pigeon, sparrow, feral swine, capybara, and rabbit can also serve as potential natural intermediate hosts for this pathogen. Although N. caninum has been detected in several mammals and birds, further investigation into the lifecycle and hosts of this pathogen is required [5162630]. Cockroaches are vectors or potential transmitters of protozoans such as T. gondii, Sarcocystis oocysts, and others [142737]. Our study detected the N. caninum genome in a cockroach sample for the first time, implying that cockroaches may play a role in its life cycle. Alternatively, cockroaches may serve as a potential vector of N. caninum.
Our results have shown that insects and rodents, while acting as potential vectors and reservoirs of cattle pathogens, can carry more than one pathogen at the same time. This is the first demonstration of vectors and reservoirs acting in tandem to transmit more than one infectious agent.
Figures and Tables
Table 3
Results of sensitivity tests for bovine abortive pathogens obtained by using the LightCycler Nano (Roche Diagnostics)

Table 4
Results for the LOD of bovine abortive and respiratory disease complex pathogens obtained by using the Applied Biosystems 7300 Real-Time PCR System (Applied Biosystems)
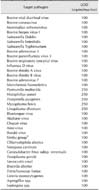
References
1. Alley MR, Connolly JH, Fenwick SG, Mackereth GF, Leyland MJ, Rogers LE, Haycock M, Nicol C, Reed CE. An epidemic of salmonellosis caused by Salmonella Typhimurium DT160 in wild birds and humans in New Zealand. N Z Vet J. 2002; 50:170–176.


2. Barkallah M, Gharbi Y, Hassena AB, Slima AB, Mallek Z, Gautier M, Greub G, Gdoura R, Fendri I. Survey of infectious etiologies of bovine abortion during mid- to late gestation in dairy herds. PLoS One. 2014; 9:e91549.


3. Chamorro MF, Passler T, Givens MD, Edmondson MA, Wolfe DF, Walz PH. Evaluation of transmission of bovine viral diarrhea virus (BVDV) between persistently infected and naive cattle by the horn fly (Haematobia irritans). Vet Res Commun. 2011; 35:123–129.


4. Desquesnes M, Biteau-Coroller F, Bouyer J, Dia ML, Foil L. Development of a mathematical model for mechanical transmission of trypanosomes and other pathogens of cattle transmitted by tabanids. Int J Parasitol. 2009; 39:333–346.


5. Feng Y, Lu Y, Wang Y, Liu J, Zhang L, Yang Y. Toxoplasma gondii and Neospora caninum in free-range chickens in Henan Province of China. Biomed Res Int. 2016; 2016:8290536.
6. Givens MD. A clinical, evidence-based approach to infectious causes of infertility in beef cattle. Theriogenology. 2006; 66:648–654.


7. Glawischnig W, Lazar J, Wallner A, Kornschober C. Cattle-derived Salmonella enterica serovar Dublin infections in red foxes (Vulpes vulpes) in Tyrol, Austria. J Wildl Dis. 2017; 53:361–363.


8. Haydon DT, Cleaveland S, Taylor LH, Laurenson MK. Identifying reservoirs of infection: a conceptual and practical challenge. Emerg Infect Dis. 2002; 8:1468–1473.


9. Heuer C, Healy A, Zerbini C. Economic effects of exposure to bovine viral diarrhea virus on dairy herds in New Zealand. J Dairy Sci. 2007; 90:5428–5438.


10. Hilton WM. BRD in 2014: where have we been, where are we now, and where do we want to go? Anim Health Res Rev. 2014; 15:120–122.


11. Hovingh E. Abortions in Dairy Cattle I: Common Causes of Abortions. Blacksburg: Virginia Polytechnic Institute and State University;2009. p. 288–404.
12. Jiménez-Clavero MA, Escribano-Romero E, Mansilla C, Gómez N, Córdoba L, Roblas N, Ponz F, Ley V, Sáiz JC. Survey of bovine enterovirus in biological and environmental samples by a highly sensitive real-time reverse transcription-PCR. Appl Environ Microbiol. 2005; 71:3536–3543.


13. Kishimoto M, Tsuchiaka S, Rahpaya SS, Hasebe A, Otsu K, Sugimura S, Kobayashi S, Komatsu N, Nagai M, Omatsu T, Naoi Y, Sano K, Okazaki-Terashima S, Oba M, Katayama Y, Sato R, Asai T, Mizutani T. Development of a one-run real-time PCR detection system for pathogens associated with bovine respiratory disease complex. J Vet Med Sci. 2017; 79:517–523.


14. Kopanic RJ Jr, Sheldon BW, Wright CG. Cockroaches as vectors of Salmonella: laboratory and field trials. J Food Prot. 1994; 57:125–135.
15. Ley V, Higgins J, Fayer R. Bovine enteroviruses as indicators of fecal contamination. Appl Environ Microbiol. 2002; 68:3455–3461.


16. Li J, He P, Yu Y, Du L, Gong P, Zhang G, Zhang X. Detection of Neospora caninum-DNA in feces collected from dogs in Shenyang (China) and ITS1 phylogenetic analysis. Vet Parasitol. 2014; 205:361–364.


17. Lucchese L, Benkirane A, Hakimi I, El Idrissi A, Natale A. Seroprevalence study of the main causes of abortion in dairy cattle in Morocco. Vet Ital. 2016; 52:13–19.
18. Luong ML, Clancy CJ, Vadnerkar A, Kwak EJ, Silveira FP, Wissel MC, Grantham KJ, Shields RK, Crespo M, Pilewski J, Toyoda Y, Kleiboeker SB, Pakstis D, Reddy SK, Walsh TJ, Nguyen MH. Comparison of an Aspergillus real-time polymerase chain reaction assay with galactomannan testing of bronchoalvelolar lavage fluid for the diagnosis of invasive pulmonary aspergillosis in lung transplant recipients. Clin Infect Dis. 2011; 52:1218–1226.


19. Miles DG. Overview of the North American beef cattle industry and the incidence of bovine respiratory disease (BRD). Anim Health Res Rev. 2009; 10:101–103.


20. Moré G, Schares S, Maksimov A, Conraths FJ, Venturini MC, Schares G. Development of a multiplex real time PCR to differentiate Sarcocystis spp. affecting cattle. Vet Parasitol. 2013; 197:85–94.


21. Olsen AR. Regulatory action criteria for filth and other extraneous materials. III. Review of flies and foodborne enteric disease. Regul Toxicol Pharmacol. 1998; 28:199–211.
22. Olsen AR, Hammack TS. Isolation of Salmonella spp. from the housefly, Musca domestica L., and the dump fly, Hydrotaea aenescens (Wiedemann) (Diptera: Muscidae), at caged-layer houses. J Food Prot. 2000; 63:958–960.


23. Pantchev A, Sting R, Bauerfeind R, Tyczka J, Sachse K. New real-time PCR tests for species-specific detection of Chlamydophila psittaci and Chlamydophila abortus from tissue samples. Vet J. 2009; 181:145–150.


24. Probert WS, Schrader KN, Khuong NY, Bystrom SL, Graves MH. Real-time multiplex PCR assay for detection of Brucella spp., B. abortus, and B. melitensis. J Clin Microbiol. 2004; 42:1290–1293.


25. Rodríguez-Lázaro D, Hernández M, Scortti M, Esteve T, Vázquez-Boland JA, Pla M. Quantitative detection of Listeria monocytogenes and Listeria innocua by real-time PCR: assessment of hly, iap, and lin02483 targets and AmpliFluor technology. Appl Environ Microbiol. 2004; 70:1366–1377.


26. Salman D, Oohashi E, Mohamed AE, Abd El-Mottelib Ael-R, Okada T, Igarashi M. Seroprevalences of Toxoplasma gondii and Neospora caninum in pet rabbits in Japan. J Vet Med Sci. 2014; 76:855–862.


27. Sarwar M. Insect vectors involving in mechanical transmission of human pathogens for serious diseases. Int J Bioinform Biomed Eng. 2015; 1:300–306.
28. Shirafuji H, Yazaki R, Shuto Y, Yanase T, Kato T, Ishikura Y, Sakaguchi Z, Suzuki M, Yamakawa M. Broad-range detection of arboviruses belonging to Simbu serogroup lineage 1 and specific detection of Akabane, Aino and Peaton viruses by newly developed multiple TaqMan assays. J Virol Methods. 2015; 225:9–15.


29. Stoddard RA, Gee JE, Wilkins PP, McCaustland K, Hoffmaster AR. Detection of pathogenic Leptospira spp. through TaqMan polymerase chain reaction targeting the LipL32 gene. Diagn Microbiol Infect Dis. 2009; 64:247–255.


30. Truppel JH, Montiani-Ferreira F, Lange RR, Vilani RG, Reifur L, Boerger W, da Costa-Ribeiro MC, Thomaz-Soccol V. Detection of Neospora caninum DNA in capybaras and phylogenetic analysis. Parasitol Int. 2010; 59:376–379.


31. Tsuchiaka S, Masuda T, Sugimura S, Kobayashi S, Komatsu N, Nagai M, Omatsu T, Furuya T, Oba M, Katayama Y, Kanda S, Yokoyama T, Mizutani T. Development of a novel detection system for microbes from bovine diarrhea by real-time PCR. J Vet Med Sci. 2016; 78:383–389.


32. van der Graaf-van Bloois L, van Bergen MA, van der Wal FJ, de Boer AG, Duim B, Schmidt T, Wagenaar JA. Evaluation of molecular assays for identification Campylobacter fetus species and subspecies and development of a C. fetus specific real-time PCR assay. J Microbiol Methods. 2013; 95:93–97.
33. Wernike K, Hoffmann B, Beer M. Simultaneous detection of five notifiable viral diseases of cattle by single-tube multiplex real-time RT-PCR. J Virol Methods. 2015; 217:28–35.


34. Wong K, Xagoraraki I. Quantitative PCR assays to survey the bovine adenovirus levels in environmental samples. J Appl Microbiol. 2010; 109:605–612.


35. Yanase T, Kato T, Aizawa M, Shuto Y, Shirafuji H, Yamakawa M, Tsuda T. Genetic reassortment between Sathuperi and Shamonda viruses of the genus Orthobunyavirus in nature: implications for their genetic relationship to Schmallenberg virus. Arch Virol. 2012; 157:1611–1616.


Supplementary Materials
Supplementary data is available at http://www.vetsci.org only.