Abstract
BACKGROUND/OBJECTIVES
Reactive oxygen species (ROS) formation is closely related to miconazole-induced heart dysfunction. Although rhamnetin has antioxidant effects, it remained unknown whether it can protect against miconazole-induced cardiomyocyte apoptosis. Thus, we investigated the effects of rhamnetin on miconazole-stimulated H9c2 cell apoptosis.
MATERIALS/METHODS
Cell morphology was observed by inverted microscope and cell viability was determined using a WelCount™ cell proliferation assay kit. Miconazole-induced ROS production was evaluated by fluorescence-activated cell sorting with 6-carboxy-2',7'-dichlorofluoroscein diacetate (H2DCF-DA) stain. Immunoblot analysis was used to determine apurinic/apyrimidinic endonuclease 1 (APE/Ref-1) and cleaved cysteine-aspartic protease (caspase) 3 expression. NADPH oxidase levels were measured using real-time polymerase chain reaction.
RESULTS
Miconazole (3 and 10 µM) induced abnormal morphological changes and cell death in H9c2 cells. Rhamnetin enhanced the viability of miconazole (3 µM)-treated cells in a dose-dependent manner. Rhamnetin (1 and 3 µM) treatment downregulated cleaved caspase 3 and upregulated APE/Ref-1 expression in miconazole-stimulated cells. Additionally, rhamnetin significantly reduced ROS generation.
Cardiovascular disease is a life-threatening and prevalent disorder in industrialized countries [12]. It is caused by the narrowing of blood vessels, which can reduce the blood supply to the heart, thereby damaging the heart muscle [3]. Furthermore, acute coronary syndrome requires sudden therapy, such as in-stent treatment within 90 min, because it can cause permanent heart failure [4]. However, ischemia/reperfusion injury after in-stent therapy can generate high quantities of reactive oxygen species (ROS), consequently inducing oxidative stress and cardiomyocyte apoptosis [5].
Miconazole, an azole antifungal agent, is used to treat cutaneous fungal diseases, including dermatophytosis, ringworm, and jock itch [6]. Miconazole exerts its antifungal activity by generating ROS, thereby inducing oxidative damage that kills fungi [7]. Superoxide (O2-) and hydrogen peroxide (H2O2) are intracellular signaling molecules associated with damage in numerous cell types [8]. Moreover, ROS are closely related to heart failure, which is attributable to cardiomyocyte apoptosis. Apoptosis, or programmed cell death, is a homeostatic process that removes abnormal, mutated, or damaged cells. The apoptotic signaling pathway involves cysteine-aspartic protease (caspase) 3 cleavage. In contrast, cell proliferation increases the population of healthy cells [9101112]. However, cellular replication is impossible in cardiomyocytes. Thus, identifying methods to prevent cardiomyocyte apoptosis is essential [13].
Antioxidants can prevent major disorders, including cancer and heart disease [1415]. Moreover, antioxidant proteins can prevent apoptotic signaling pathways in damaged cardiomyocytes [16]. For example, the multifunctional antioxidant enzyme apurinic/apyrimidinic endonuclease 1 (APE/Ref-1) may prevent cardiomyocyte damage [17]. Rhamnetin, a flavonoid, is a bioactive polyphenolic compound that is commonly found in fruits and vegetables [18]. It is a potent antioxidant that exerts beneficial effects against oxidative- and free radical-mediated cell injury [1920]. However, the effect of rhamnetin on miconazole-mediated cardiomyocyte apoptosis remains unclear. Therefore, we explored the effect of rhamnetin on miconazole-induced H9c2 cardiomyoblast apoptosis.
Miconazole (Fig. 1A) and rhamnetin (Fig. 2A) were purchased from Sigma-Aldrich (St. Louis, MO, USA). Cell culture materials were purchased from Gibco BRL (Gaithersburg, MD, USA). Anti-APE/Ref-1, anti-cleaved caspase3, and anti-GAPDH antibodies were obtained from Santa Cruz Biotechnology (Santa Cruz, CA, USA). All other chemicals were purchased from Sigma or Amersham Pharmacia (Piscataway, NJ, USA).
Rat embryonic cardiomyoblast-derived H9c2 cells were obtained from the American Type Culture Collection (Rockville, MD, USA). Cells were cultured in Dulbecco's modified Eagle's medium (DMEM) containing 10% fetal bovine serum (FBS), 100 U/ml penicillin, 100 µg/ml streptomycin, and 200 mM glutamine, in a 95% air/5% CO2 atmosphere at 37℃. For all experiments, H9c2 cells were grown to 70-80% confluence and trypsinized every 3-5 days.
To determine cell viability, a 2,3-bis [2-methyloxy-4-nitro-5-sulfophenyl]-2H-tetrazolium-5-carboxanilide (XTT) assay was employed using the WelCount™ cell proliferation assay kit (WELLGENE, Daegu, South Korea). H9c2 cells were harvested with trypsin-EDTA and resuspended in culture medium. H9c2 cells (5 × 103 cells/well) were then seeded in 96-well plates and cultivated in DMEM containing 10% FBS for 24 h. Cells were then cultivated in serum-free DMEM for 6 h, followed by incubation in serum-free medium containing rhamnetin or miconazole for 24 h. XTT dye was added to each well and incubated for 3 h. Formazan dye formation was quantitated with an enzyme-linked immunosorbent assay reader at 450 nm. Morphological changes in the cells were observed, and images were captured under an inverted microscope connected to a digital camera (IX71; Olympus, Tokyo, Japan).
Generation of intracellular ROS in H9c2 cells was measured using the green fluorescence probe 6-carboxy-2',7'-dichlorofluoroscein diacetate (H2DCF-DA; Invitrogen, Rockville, MD, USA) and fluorescence-activated cell sorting (FACS; BD FACSCalibur™; BD Bioscience, San Jose, CA, USA).
Total RNA was isolated from cells using the Maglisto™ cell total RNA extraction kit (Bionner, Daejeon, Korea) according to the manufacturer's instructions. And, first strand cDNA was synthesized from 0.5 µg of total RNA using the Superscript™ II reverse transcription system (Invitrogen, Carlsbad, CA, USA) following the manufacturer's instructions. Real-time polymerase chain reaction (qPCR) was performed using 20 µL of the Accupower® GreenStar™ prermix (Bioneer, Daejeon, Korea) under the following conditions: initial denaturation (10 min, 95℃) followed by 40 cycles of denaturation (10 s, 95℃), annealing, and extension (30 s, 60℃). The following primers were used for the qPCR: NOX1, sense 5'-TTCCTCACTGGCTGGGATA-3' and anti-sense, 5'-TGACAGCATTTGCGCAGGCT-3'; NOX2, sense 5'-CAGTGAAGATGTGTTCAGCT-3' and anti-sense, 5'-GCACAGCCAGTAGAAGTAGAT-3'; NOX4, sense, 5'-AGTCAAACAGATGGGATA-3' and anti-senser, 5'-TGTCCCATATGAGTTGTT-3'; and GAPDH, sense 5'-TGAACGGGAAGCTCACTGG-3' and antisense 5'-TCCACCACCCTGTTGCTGTA-3'. The relative gene expression levels were determined by calculating the value of the Δcycle threshold (ΔCt), normalizing the average Ct value to the control GAPDH, and then calculating 2-ΔΔCt.
Cells were lysed with cold extraction buffer (20 mM HEPES, 1% Nonidet P-40, 150 mM NaCl, 10% glycerol, 10 mM NaF, 1 mM Na3VO4, 2.5 mM 4-nitrophenylphosphate, 0.5 mM PMSF, and 1 tablet of complete proteinase inhibitor cocktail, pH 7.5). The lysates were centrifuged (13,000 × g, 15 min, 4℃), and the supernatants were collected. Protein concentrations were determined using the Bio-Rad DC protein assay reagent (Hercules, CA, USA). The protein homogenates were diluted 1:1 (v/v) with sodium dodecyl sulfate (SDS) sample buffer containing 40 mM Tris (pH 6.8), 8 mM EGTA, 4% 2-mercaptoethanol, 40% glycerol, 0.01% bromophenol blue, and 4% SDS and boiled for 5 min. Proteins (20 µg/lane) were separated using 12% polyacrylamide SDS gels and transferred electrophoretically to a polyvinylidene fluoride membrane (Millipore, Bedford, MA, USA). The membrane was then blocked for 1 h at room temperature with PBS containing 0.05% Tween 20 and 5% fat-free dried milk. The membranes were incubated with antibodies diluted 1:1,000 overnight at 4℃. Immune complexes were detected with horse-radish peroxidase-conjugated antibodies (Amersham Pharmacia) diluted 1:1,000 and incubated for 1 h at room temperature. After application of the secondary antibody, the blots were incubated in enhanced chemiluminescence detection reagent (Amersham Pharmacia). Band intensity was analyzed by Luminescent Image Analyzer LAS-4000 (Fujifilm, Tokyo, Japan).
Data were expressed as the mean ± SE of the mean. Statistical evaluation of the data was performed using GraphPad prism, version 5.0 (GraphPad Software), the Student's t-test, and one-way analysis of variance (ANOVA) with Tukey's post-hoc test to compare the data. P-values less than 0.05 were considered statistically significant.
We performed cell viability assays to test miconazole-induced cell damage. H9c2 cells were treated with miconazole (3 and 10 µM) for 24 h. As the concentration of miconazole increased from 3 to 10 µM, the cell viability dose-dependently decreased (Fig. 1B). Furthermore, concentration-dependent abnormal morphological changes were observed. Therefore, we used 3 µM miconazole in further experiments.
H9c2 cells were treated with rhamnetin (1, 3, or 5 µM) and 3 µM miconazole for 24 h to evaluate the effects of rhamnetin on miconazole-induced cell death. As the concentration of rhamnetin increased, the cell viability increased in a dose-dependent manner. In contrast, H9c2 cells treated with miconazole alone exhibited over 40% cell death. Treatment with rhamnetin (3 µM) for 24 h in the presence of miconazole (3 µM) did not alter cytotoxicity or morphology (Fig. 2). Therefore, we used up to 3 µM rhamnetin in all experiments.
Cardiomyocte apoptosis contributes to abnormal morphological changes and these events are induced by miconazole [10]. During apoptosis, signaling molecules induce the expression of apoptosis regulatory proteins that underlie the caspase-3-dependent pathway [12]. Thus, to confirm the anti-apoptotic effect of rhamnetin during miconazole-induced apoptosis, we explored the expression levels of caspase 3 by western blot. As shown in Fig. 3, miconazole treatment significantly increased cleaved-caspase 3, whereas rhamnetin treatment significantly decreased miconazole-induced caspase-3 cleavage in H9c2 cells.
Miconazole induces ROS generation, thereby elevating oxidative stress [7]. Therefore, we evaluated whether rhamnetin could reduce ROS generation and the expression of ROS-dependent proteins.
First, we evaluated the mRNA expression of the NOX family members, proteins upstream of H2O2, using real-time PCR with specific primers. Rhamnetin did not alter miconazole-induced expression of NOX family members in H9c2 cells. Next, we measured H2O2 by H2DCF-DA staining. Miconazole treatment increased H2O2 levels in H9c2 cells; however, this effect was attenuated by rhamnetin. In addition, we assessed APE/Ref-1 expression in the presence or absence of rhamnetin and/or miconazole. Rhamnetin significantly inhibited the miconazole-induced reduction of APE/Ref-1 expression in H9c2 cells (Fig. 4).
In the present study, we demonstrated that rhamnetin protects against miconazole-induced H9c2 cell death via the ROS pathway. Our results indicate that rhamnetin regulates APE/Ref-1 expression, thereby inhibiting miconazole-induced ROS generation and apoptosis.
ROS are extremely unstable and reactive. Although ROS generated during respiration are removed from the body, residual ROS can induce oxidative stress, causing numerous diseases, including cancer, metabolic disorders, and cardiovascular disease [212223]. In particular, heart muscle cells can be damaged by excessive oxidative stress due to hypoxia/reperfusion following stenting or cardiac bypass surgery [24]. Our results indicate that rhamnetin inhibits miconazole-induced ROS production.
We evaluated the effect of rhamnetin on ROS-induced damage in H9c2 cells. Interestingly, rhamnetin did not alter miconazole-induced NOX1 expression in H9c2 cells. NOX family is an important enzymatic sources of ROS production, and generates superoxide radicals associated with pathological reactions, including inflammation, cell migration endothelial dysfunctions, vascular remodeling and apoptotic cell death [2526]. Upregulated expression of NOX family enzymes can induce apoptosis in cells [27]. In particular, NOX2 and NOX4 are highly expressed in cardiomyocytes, whereas NOX1 expression is relatively low [2829]. NOX1 is tightly associated with endotoxin stress-induced cardiomyocyte apoptosis [30]. Our results confirmed that miconazole induced ROS generation and NOX1 expression, whereas rhamnetin did not alter miconazole-induced NOX1 expression in H9c2 cells. We hypothesized that rhamnetin may regulate ROS production downstream of superoxide, because rhamnetin limited H2O2 production. Park et al. demonstrated that rhamnetin upregulated the expression of manganese superoxide dismutase (MnSOD) and catalase following H2O2-induced cardiomyocyte damage [31]. However, these results raised other questions on the effect of rhamnetin, including how to regulate ROS-related signaling pathways.
To address this question, we evaluated the effect of rhamnetin on APE1/Ref-1 expression.
Especially, APE/Ref-1 protein, a ubiquitously expressed multifunctional protein, encodes the redox function and DNA repair activity as well as AP endonuclease, which induces redox homeostasis and repair of cellular DNA [32]. Won et al. demonstrated that overexpressed APE1 protects against miconazole-induced cardiomyocyte damage [17]. In the present study, rhamnetin enhanced APE/Ref-1 expression in miconazole-treated H9c2 cells. Therefore, we propose that rhamnetin in foods may protect against heart failure.
Although research is necessary to validate the efficacy and establish the appropriate dietary levels of rhamnetin, reports suggest it may improve memory, reduce arthritis, and reduce cardiovascular disease [18]. Moreover, because nutrient imbalance in western diets may lead to heart disease, it is may represent a nutritional or functional food. Therefore, we propose that foods containing rhamnetin may protect cardiomyocytes. The results indicate that rhamnetin may effectively prevent cardiomyocyte dysfunction.
In conclusion, rhamnetin is a candidate for the treatment of heart dysfunction because of its ability to protect against miconazole-induced cell death. Furthermore, rhamnetin functions as a ROS scavenger through modulation of APE1/Ref-1 signaling.
Figures and Tables
Fig. 1
Effect of miconazole on H9c2 cell viability.
(A) The chemical structure of miconazole 1-(2,4-dichloro-β-[(2,4-dichlorobenzyl)oxy] phenethyl) imidazole; molecular weight 479.14. (B) Cell morphology was observed after 24 h miconazole (Mico) treatment (3 and 10 µM). (C) H9c2 cells were treated with miconazole (3 and 10 µM) for 24 h and cytotoxicity in the quiescent state was analyzed by XTT assay. The results represent the mean ± SE of three independent experiments. Values with the same superscript letter are not significantly different based on Tukey's multiple range test (P < 0.05).
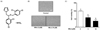
Fig. 2
Effect of rhamnetin on miconazole-stimulated H9c2 cell viability.
(A) The chemical structure of rhamnetin (Rham; molecular weight 316.26). (B) Cell morphology was observed after 24 h in the presence or absence of miconazole (Mico; 3 µM) and rhamnetin (1 and 3 µM). (C) H9c2 cells were co-treated with miconazole (3 µM) and rhamnetin (1, 3 and 5 µM) for 24 h, and cytotoxicity was evaluated in the quiescent state by XTT assay. The results represent the mean ± SE of three independent experiments. Values with the same superscript letter are not significantly different based on Tukey's multiple range test (P < 0.05).
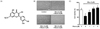
Fig. 3
Effect of rhamnetin on miconazole-induced caspase3 cleavage in H9c2 cells.
Cells were incubated in serum-free medium, followed by treatment with miconazole (Mico;3 µM) and rhamnetin (Rham;1 and 3 µM) for 24 h. (A) The cells were lysed, and proteins were resolved by SDS-PAGE, transferred to a PVDF membrane, and blotted with anti-cleaved capase3 antibodies to analyze protein expression. (B) Statistical results obtained from panel A. The basal levels of caspase3 were considered 100% (n = 3). The results represent the mean ± SE from three independent experiments. Values with the same superscript letter are not significantly different based on Tukey's multiple range test (P < 0.05).
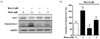
Fig. 4
Effect of rhamnetin on miconazole-induced ROS production and signaling in H9c2 cells.
Cells were cultured in serum-free medium, followed by miconazole (Mico;3 µM) and rhamnetin (Rham;1 and 3 µM) stimulation for 24 h. (A) Rhamnetin reduced miconazloe-induced ROS production in H9c2 cells as measured by FACS using H2DCF-DA stain (Left panel). Bar graph displays the the percentage of fluorescence cells on left panel. (B) mRNA expression levels of NOX family proteins (NOX1, NOX2, and NOX4) were measured by real-time polymerase chain reaction. The relative levels of mRNA were calculated by using 2-ΔΔCt values and normalizing GAPDH. (C) Proteins were separated and blotted with APE/Ref-1 antibodies to analyze protein expression. (D) Statistical results obtained from panel C. GAPDH was used for normalization. These results represent the mean ± SE from three independent experiments. Values with the same superscript letter are not significantly different based on Tukey's multiple range test (P < 0.05).
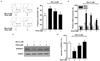
References
1. Mendis S, Lindholm LH, Anderson SG, Alwan A, Koju R, Onwubere BJ, Kayani AM, Abeysinghe N, Duneas A, Tabagari S, Fan W, Sarraf-Zadegan N, Nordet P, Whitworth J, Heagerty A. Total cardiovascular risk approach to improve efficiency of cardiovascular prevention in resource constrain settings. J Clin Epidemiol. 2011; 64:1451–1462.


2. Ministry of Health and Welfare. Korea Centers for Disease Control and Prevention. Korea Health Statistics 2012: Korea National Health and Nutrition Examination Survey (KNHANES V-3). Cheongwon: Korea Centers for Disease Control and Prevention;2013.
3. Ross R. The pathogenesis of atherosclerosis: a perspective for the 1990s. Nature. 1993; 362:801–809.


4. Müller C. New ESC guidelines for the management of acute coronary syndromes in patients presenting without persistent ST-segment elevation. Swiss Med Wkly. 2012; 142:w13514.


5. Zweier JL, Talukder MA. The role of oxidants and free radicals in reperfusion injury. Cardiovasc Res. 2006; 70:181–190.


6. Coley KC, Crain JL. Miconazole-induced fatal dysrhythmia. Pharmacotherapy. 1997; 17:379–382.
7. Kobayashi D, Kondo K, Uehara N, Otokozawa S, Tsuji N, Yagihashi A, Watanabe N. Endogenous reactive oxygen species is an important mediator of miconazole antifungal effect. Antimicrob Agents Chemother. 2002; 46:3113–3117.


8. Irani K, Xia Y, Zweier JL, Sollott SJ, Der CJ, Fearon ER, Sundaresan M, Finkel T, Goldschmidt-Clermont PJ. Mitogenic signaling mediated by oxidants in Ras-transformed fibroblasts. Science. 1997; 275:1649–1652.


9. Dumaine R, Roy ML, Brown AM. Blockade of HERG and Kv1.5 by ketoconazole. J Pharmacol Exp Ther. 1998; 286:727–735.
12. Shih PH, Yeh CT, Yen GC. Anthocyanins induce the activation of phase II enzymes through the antioxidant response element pathway against oxidative stress-induced apoptosis. J Agric Food Chem. 2007; 55:9427–9435.


13. Kim DE, Kim B, Shin HS, Kwon HJ, Park ES. The protective effect of hispidin against hydrogen peroxide-induced apoptosis in H9c2 cardiomyoblast cells through Akt/GSK-3β and ERK1/2 signaling pathway. Exp Cell Res. 2014; 327:264–275.


14. Baines CP, Molkentin JD. STRESS signaling pathways that modulate cardiac myocyte apoptosis. J Mol Cell Cardiol. 2005; 38:47–62.


15. Glasauer A, Chandel NS. Targeting antioxidants for cancer therapy. Biochem Pharmacol. 2014; 92:90–101.


16. Núñez-Córdoba JM, Martínez-González MA. Antioxidant vitamins and cardiovascular disease. Curr Top Med Chem. 2011; 11:1861–1869.


17. Won KJ, Lin HY, Jung S, Cho SM, Shin HC, Bae YM, Lee SH, Kim HJ, Jeon BH, Kim B. Antifungal miconazole induces cardiotoxicity via inhibition of APE/Ref-1-related pathway in rat neonatal cardiomyocytes. Toxicol Sci. 2012; 126:298–305.


18. Ozipek M, Caliş I, Ertan M, Rüedi P. Rhamnetin 3-p-coumaroylrhamninoside from Rhamnus petiolaris. Phytochemistry. 1994; 37:249–253.


19. Igarashi K, Ohmuma M. Effects of isorhamnetin, rhamnetin, and quercetin on the concentrations of cholesterol and lipoperoxide in the serum and liver and on the blood and liver antioxidative enzyme activities of rats. Biosci Biotechnol Biochem. 1995; 59:595–601.


20. Yamamoto N, Moon JH, Tsushida T, Nagao A, Terao J. Inhibitory effect of quercetin metabolites and their related derivatives on copper ion-induced lipid peroxidation in human low-density lipoprotein. Arch Biochem Biophys. 1999; 372:347–354.


21. Slimen IB, Najar T, Ghram A, Dabbebi H, Ben Mrad M, Abdrabbah M. Reactive oxygen species, heat stress and oxidative-induced mitochondrial damage. A review. Int J Hyperthermia. 2014; 30:513–523.


22. Sullivan LB, Chandel NS. Mitochondrial reactive oxygen species and cancer. Cancer Metab. 2014; 2:17.


23. Paneni F, Costantino S, Cosentino F. Role of oxidative stress in endothelial insulin resistance. World J Diabetes. 2015; 6:326–332.


24. Brown DI, Griendling KK. Regulation of signal transduction by reactive oxygen species in the cardiovascular system. Circ Res. 2015; 116:531–549.


25. Manea A. NADPH oxidase-derived reactive oxygen species: involvement in vascular physiology and pathology. Cell Tissue Res. 2010; 342:325–339.


26. Bedard K, Krause KH. The NOX family of ROS-generating NADPH oxidases: physiology and pathophysiology. Physiol Rev. 2007; 87:245–313.


27. Puca R, Nardinocchi L, Starace G, Rechavi G, Sacchi A, Givol D, D'Orazi G. Nox1 is involved in p53 deacetylation and suppression of its transcriptional activity and apoptosis. Free Radic Biol Med. 2010; 48:1338–1346.


28. Kuroda J, Ago T, Matsushima S, Zhai P, Schneider MD, Sadoshima J. NADPH oxidase 4 (Nox4) is a major source of oxidative stress in the failing heart. Proc Natl Acad Sci U S A. 2010; 107:15565–15570.


29. Kuroda J, Sadoshima J. NADPH oxidase and cardiac failure. J Cardiovasc Transl Res. 2010; 3:314–320.


30. Matsuno K, Iwata K, Matsumoto M, Katsuyama M, Cui W, Murata A, Nakamura H, Ibi M, Ikami K, Zhang J, Matoba S, Jin D, Takai S, Matsubara H, Matsuda N, Yabe-Nishimura C. NOX1/NADPH oxidase is involved in endotoxin-induced cardiomyocyte apoptosis. Free Radic Biol Med. 2012; 53:1718–1728.

