Abstract
Ketamine is an anesthetic with hypertensive effects, which make it useful for patients at risk of shock. However, previous ex vivo studies reported vasodilatory actions of ketamine in isolated arteries. In this study, we reexamined the effects of ketamine on arterial tones in the presence and absence of physiological concentrations of 5-hydroxytryptamine (5-HT) and norepinephrine (NE) by measuring the isometric tension of endothelium-denuded rat mesenteric arterial rings. Ketamine little affected the resting tone of control mesenteric arterial rings, but, in the presence of 5-HT (100~200 nM), ketamine (10~100 µM) markedly contracted the arterial rings. Ketamine did not contract arterial rings in the presence of NE (10 nM), indicating that the vasoconstrictive action of ketamine is 5-HT-dependent. The concentration-response curves (CRCs) of 5-HT were clearly shifted to the left in the presence of ketamine (30 µM), whereas the CRCs of NE were little affected by ketamine. The left shift of the 5-HT CRCs caused by ketamine was reversed with ketanserin, a competitive 5-HT2A receptor inhibitor, indicating that ketamine facilitated the activation of 5-HT2A receptors. Anpirtoline and BW723C86, selective agonists of 5-HT1B and 5-HT2B receptors, respectively, did not contract arterial rings in the absence or presence of ketamine. These results indicate that ketamine specifically enhances 5-HT2A receptor-mediated vasoconstriction and that it is vasoconstrictive in a clinical setting. The facilitative action of ketamine on 5-HT2A receptors should be considered in ketamine-induced hypertension as well as in the pathogenesis of diseases such as schizophrenia, wherein experimental animal models are frequently generated using ketamine.
Ketamine is a non-competitive N-methyl-D-aspartate (NMDA) receptor antagonist that is clinically used for anesthesia. In contrast to other anesthetics, ketamine raises blood pressure at clinical concentrations [12345]. This unique characteristic of ketamine makes it useful for patients at risk for shock due to severe hemorrhage or hypotension. However, the use of ketamine in patients with hypertension or coronary disease is contraindicated [46]. Although ketamine-induced hypertension is largely known to occur through the inhibition of catecholamine reuptake in the sympathetic nervous system, its mechanism is not completely understood thus far [12].
To study ketamine-induced hypertension, several previous studies examined the hypothesis that ketamine contracts arteries to increase peripheral vascular resistance. However, these previous ex vivo and in vitro studies reported that ketamine has a vasodilatory effect on isolated arteries or smooth muscle cells [7891011]. An important point to be considered when interpreting these results is that most of these studies evaluated the direct ex vivo effect of ketamine on arteries after pre-contraction with high concentrations of vasoconstrictors such as KCl or norepinephrine (NE) [7].
It was also reported that the psychogenic effects of NMDA receptor antagonists such as ketamine, MK-801, and phencyclidine seem to go beyond the inhibition of the NMDA receptor; both dopamine D2 and serotonin 5-HT2 receptors are reportedly related to the pathogenesis of schizophrenia, and the agonistic effects of ketamine on D2 receptors and 5-HT2 receptors have been reported [121314]. Because the 5-HT2A receptor is a major subtype found in peripheral arteries, ketamine can act as a vasoconstrictor, provided that it can activate the 5-HT2A receptor in a clinical setting (i.e., in the presence of resting concentrations of 5-HT, which were reported to be ~100 nM [15]).
Here, we re-examined the direct effect of ketamine on the isolated arterial mechanics under physiological conditions, in the presence of physiological resting concentrations of vasoactive agonists such as 5-HT and NE. We found that ketamine is a vasoconstrictor in the presence of physiological concentrations of 5-HT, because of the facilitating effect of ketamine on the activation of serotonin 5-HT2A receptors. We expect that these results will contribute to interpreting the hypertensive effect of ketamine in a clinical setting. Furthermore, the results of this study could provide valuable insight into interpreting the pathogenesis of schizophrenia, since over-activity of 5-HT2A receptors may play a role and ketamine is used for animal models of the disorder [121314].
All experiments were conducted in accordance with the National Institutes of Health guidelines for the care and use of animals. The Institutional Animal Care and Use Committee of Konkuk University approved this study. Mesenteric arterial rings were prepared as previously described [16]. Male Sprague-Dawley (SD) rats (10- to 11-week-old) were exsanguinated by cutting the carotid arteries under deep ketamine-xylazine anesthesia or after exposure to rising concentrations of carbon dioxide. The branches of the superior mesenteric arteries were promptly isolated and placed in physiological saline solutions (PSS) containing 136.9 mM NaCl, 5.4 mM KCl, 1.5 mM CaCl2, 1.0 mM MgCl2, 23.8 mM NaHCO3, 1.2 mM NaH2PO4, 0.01 mM EDTA, and 5.5 mM glucose. The arteries were carefully cleaned of fat and connective tissue under a stereomicroscope and prepared as rings (3.5 mm in length) for tension measurements. The endothelium was mechanically removed with fine stainless-steel wire [17]. Endothelial removal was confirmed by the absence of relaxation induced by acetylcholine (10 µM) after contraction by NE (1~10 µM) or 5 HT (1~10 µM).
The isometric tension of arterial rings was measured as previously described [16]. The arterial rings were mounted vertically on two L-shaped stainless steel wires in a 3 mL tissue chamber. One wire was attached to a micromanipulator and the other to an isometric force transducer (FT03; Grass, West Warwick, RI, USA). Changes in isometric force were digitally acquired at 1 Hz with a PowerLab data acquisition system (ADInstruments, Colorado Springs, CO, USA). Resting tension was set to 1 g by the micromanipulator. After equilibration for 60 min under resting tension in a tissue chamber filled with PSS, the rings were sequentially exposed to 70 mM KCl PSS (10 min) and PSS (15 min) three times for stabilization. The high KCl (70 mM) PSS was prepared by replacing NaCl with equimolar KCl in PSS. Bathing solutions were thermostatically controlled at 37℃ and were continuously saturated with a mixture of 95% O2 and 5% CO2 to achieve pH 7.4.
Ketanserin, anpirtoline, and BW723C86 were purchased from Tocris (Bristol, UK). All other chemicals, including ketamine racemate, were purchased from Sigma-Aldrich (St. Louis, MO, USA). The ketamine, NE, 5-HT, and acetylcholine were prepared as stock solutions in distilled water. The drugs were diluted in the bathing solution on the day of the experiment.
Plasma concentrations of 5-HT were reported to be between 30 and 150 nM [15], which are the subthreshold concentrations of 5-HT required for contraction of rat mesenteric arteries [1819]. The threshold for 5-HT-induced mesenteric arterial contraction was ~300 nM [19]. To examine the effect of ketamine on vascular tone in the presence of physiological concentrations of 5-HT, ketamine was administered in the presence of 100~200 nM 5-HT. Fig. 1A (upper panel) shows a typical representative trace: in the presence of physiological low concentration (100~200 nM) of 5-HT, administration of ketamine (10~100 µM) contracted the arterial rings. The lower panel of Fig. 1A summarizes the effect of ketamine on the tone of arterial rings in the presence of 100~200 nM 5-HT. In the presence of a physiological low concentration (10 nM) of NE, ketamine did not contract the arterial rings (Fig. 1B). On the contrary, a high concentration (300 µM) of ketamine dilated the arterial rings that were slightly pre-contracted with NE (10 nM, Fig. 1B). Ketamine had no effect on the resting tone of the mesenteric arterial rings in the absence of vasoactive agonists such as 5-HT and NE (Fig. 1C).
As seen in Fig. 1A, 30 µM ketamine induced maximal vasoconstriction in the presence of physiological concentrations of 5-HT. To further examine the mechanism by which ketamine contracted the arterial rings in the presence of low concentrations of 5-HT, we compared the concentration-response (contraction) curve (CRC) of 5-HT in the absence and presence of ketamine (30 µM); note that the maximal constrictive effect of ketamine in the presence of 5-HT occurred at 30 µM ketamine. As shown in Fig. 2A, ketamine (30 µM) markedly shifted the CRC of 5-HT to the left, with little effect on the maximal contraction. Although pretreatment with a high concentration (300 µM) of ketamine decreased the maximal contraction induced by 5-HT , the shift of the CRC to the left by ketamine was still evident (Fig. 2B). Contrary to the ketamine-induced shift to the left of the 5-HT CRC, 30 µM ketamine had no effect on the CRC of NE (Fig. 2C). A high concentration of ketamine (300 µM) decreased the CRC in the presence of various concentrations of NE without shifting the NE CRC (Fig. 2D).
It was reported that the 5-HT2A receptor is responsible for 5-HT-induced vasoconstriction of control rat mesenteric arteries [1819]. In the case of hypertensive arteries, the unmasking or up-regulation of 5-HT1B or 5-HT2B receptors was reported to contribute to the enhancement in the 5-HT-inudced vasoconstriction [18]. Therefore, we examined whether ketamine facilitated the activation of the 5-HT2A receptor or whether ketamine unmasked the silent 5-HT1B or 5-HT2B receptor to selectively increase the 5-HT-induced vasoconstriction (left shift of 5-HT CRC) without having an effect on the NE-induced vasoconstriction (Figs. 2A and C). A previous study reported that the 5-HT2A receptor mediated the 5-HT-induced contraction of rat mesenteric artery [19]; in our study, the 5-HT CRC was markedly shifted to the right by ketanserine (10 nM, Fig. 3A, open symbols), which is a potent competitive inhibitor of the 5-HT2A receptor. Ketanserine also similarly shifted the 5-HT CRC in the presence of ketamine (30 µM, Fig. 3A, filled symbols). These results indicate that ketamine shifted the 5-HT CRC to the left (Fig. 2A) by acting on the 5-HT2A receptor.
Furthermore, we examined the hypothesis that ketamine unmasked 5-HT1B or 5-HT2B receptors to increase the 5-HT-induced vasoconstriction. However, anpirtoline and BW723C86, the agonists for 5-HT1B and 5-HT2B receptors, respectively, failed to contract rat mesenteric arterial rings both in the absence and in the presence of ketamine (Fig. 3C). This indicates that ketamine did not unmask 5-HT1B and 5-HT2B receptors in the rat mesenteric artery.
The major finding of this study is that clinical concentrations of ketamine (10~100 µM) contract rat mesenteric arteries in the presence of physiological plasma concentrations of 5-HT. These results may explain the previous paradoxical ex vivo or in vitro vasodilatory actions of ketamine in spite of its in vivo hypertensive effect. Although the effects of ketamine on the sympathetic nervous system (i.e., inhibition of catecholamine reuptake) may be a primary mechanism for the systemic hypertensive effect of ketamine, this study clearly demonstrated that the direct effect of ketamine on arteries is not vasodilatory but vasoconstrictive in a physiological clinical setting: ketamine was not vasoconstrictive by itself (Fig. 1C), however, 5-HT at its basal plasma concentration renders ketamine "practically vasoconstrictive".
As noted above, previous ex vivo or in vitro studies reported the vasodilatory actions of ketamine. The vasodilatory effect of ketamine was reported to involve inhibition of both influx of Ca2+ from extracellular space and release of Ca2+ from intracellular Ca2+ stores [7891011]. However, the vasodilatory actions of ketamine were usually observed at concentrations above 300 µM, which is higher than the clinical plasma concentrations of ketamine [20]. Moreover, this effect was observed after precontraction by high concentrations (1~10 µM) of NE or high concentrations (40~70 mM) of KCl; these high concentrations are not likely to be maintained for a significant duration in a clinical setting [716]. In this study, we aimed to examine the effect of ketamine in more clinically relevant, physiological conditions (in the presence of sub-micromolar concentrations of 5-HT or NE) than those employed in previous studies. We found that ketamine is vasoconstrictive under these conditions because of its facilitative effect on the activation of 5-HT2A receptors. Moreover, the vasoconstrictive action of ketamine through the 5-HT2A receptor was most dominant at the concentration of 30 µM; this concentration is in the clinical range [20].
In the normal rat aorta and mesenteric artery, the 5-HT2A receptor is a primary 5-HT receptor subtype [1921], and 5-HT1B or 5-HT2B receptors can be upregulated or unmasked under conditions such as hypertension [18] and increased pre-vasocontraction with depolarization [22]. Because ketamine reportedly blocked voltage-gated K+ channels and depolarized the membrane potential of vascular myocytes [23] (which is interestingly also the mechanism of 5-HT-induced vasoconstriction [2425]), depolarization by pre-treatment with ketamine could unmask the 5-HT1B or 5-HT2B receptors [22]. The results of the present study, however, clearly demonstrate that ketamine selectively facilitated the activation of 5-HT2A receptors without unmasking 5-HT1B or 5-HT2B receptors in rat mesenteric arteries. This is supported by the following evidence: First, ketanserin, a potent and selective inhibitor of 5-HT2A receptors, reversed the facilitative (left-shifting) effect of ketamine on the CRC of 5-HT (Fig. 3A); second, anpirtoline and BW723C86 failed to contract the mesenteric arterial rings both in the absence and presence of ketamine, which indicates that other 5-HT receptor subtypes such as 5-HT1B and 5-HT2B were not involved in the increased vasoconstriction of 5-HT by ketamine; third, vasoconstriction by NE through the adrenergic receptor was not affected by ketamine. The primary receptor subtype for NE-induced vasoconstriction of the rat mesenteric artery was reported to be the adrenergic α1D receptor [262728], and the results of the present study indicate that ketamine did not affect the sensitivity of the adrenergic α1D receptor. The NE CRC was not affected by 30 µM ketamine (Fig. 2C). Considering that the 5-HT2A and adrenergic α1D receptors are similar Gq11 protein coupled receptors (GqPCR) and they share similar signaling pathways (including activation of phospholipase Cβ), it is expected that ketamine selectively and directly interacts with the 5-HT2A receptor without interacting with the downstream signaling cascade. Interestingly, the direct interaction of ketamine with dopamine D2 and 5-HT2 receptors was previously reported [12]. Involvement of NMDA receptors in the vasoconstrictive actions of ketamine can also be excluded because the rat mesenteric artery lacks functional NMDA receptors [16].
In this study, data for elucidating the mechanism of the ketamine-induced facilitation of 5-HT2A receptors were not provided. In our previous study, however, we reported that activation of src tyrosine kinase and subsequent inhibition of Kv channels are the mechanisms of 5-HT-induced vasoconstriction in rat mesenteric arteries [19]. Therefore, ketamine might have amplified src activation after 5-HT2A receptor activation. Another possibility is that ketamine augmented the inhibition of Kv channels after 5-HT2A receptor and subsequent src activation because ketamine itself blocks Kv channels in mesenteric arteries [23]. Because this mechanism warrants further investigation, we are planning a study to elucidate the mechanism of the ketamine-induced facilitation of 5-HT2A receptors. Our preliminary observation is that ketamine increases the phosphorylation of src in the presence of low concentrations of 5-HT (~30 nM), which suggests that ketamine may facilitate the src activation process after 5-HT2A receptor activation. It is also interesting that some selective serotonin reuptake inhibitors (SSRIs), which are frequently used for treating mood disorders and work by increasing 5-HT concentrations in the synaptic spaces of the central nervous system, are reported to block Kv channels, such as Kv1.5 [2930], which are the downstream targets of 5-HT2A receptors [1925].
In this study, the vasoconstrictive action of ketamine in arteries through the enhancement of the activation of 5-HT2A receptors was reported for the first time. However, in the central nervous system, interaction of ketamine and other structurally-related non-competitive inhibitors of NMDA receptors (such as MK801 and phencyclidine) with 5-HT2A receptors was previously reported [121314]. Moreover, several studies suggested that the direct interaction of ketamine and phencyclidine with 5-HT2A receptors is involved in the pathogenesis of schizophrenia by enhancing the activity of 5-HT2A receptors. In this regard, the results of the present study again strengthen the hypothesis that ketamine facilitates 5-HT2A receptor activation. The mechanism and clinical significance of the interaction of phencyclidine analogs, ketamine, MK801, and phencyclidine with 5-HT2A receptors warrant further study.
ACKNOWLEDGEMENTS
This work was supported by Basic Science Research Program through the National Research Foundation of Korea (NRF) funded by the Ministry of Education (NRF-2009-0071242).
Notes
References
1. Goodman LS, Hardman JG, Limbird LE, Gilman AG. Goodman and Gilman's the pharmacological basis of therapeutics. 10th ed. New York: McGraw-Hill;2001. p. 342–347.
2. Katzung BG. Basic & clinical pharmacology. A Concise medical library for practitioner and student. 5th ed. Los Altos, Calif: Lange Medical Publications;1982. p. 350–362.
3. White PF, Way WL, Trevor AJ. Ketamine--its pharmacology and therapeutic uses. Anesthesiology. 1982; 56:119–136. PMID: 6892475.


4. Zielmann S, Kazmaier S, Schnüll S, Weyland A. S-(+)-Ketamine and circulation. Anaesthesist. 1997; 46(Suppl 1):S43–S46. PMID: 9163278.
5. Zhang G, Cui J, Chen Y, Ma J. The relaxant effect of propofol on isolated rat intrapulmonary arteries. Korean J Physiol Pharmacol. 2014; 18:377–381. PMID: 25352756.


7. Akata T, Izumi K, Nakashima M. Mechanisms of direct inhibitory action of ketamine on vascular smooth muscle in mesenteric resistance arteries. Anesthesiology. 2001; 95:452–462. PMID: 11506120.


8. Fukuda S, Murakawa T, Takeshita H, Toda N. Direct effects of ketamine on isolated canine cerebral and mesenteric arteries. Anesth Analg. 1983; 62:553–558. PMID: 6846876.


9. Hara Y, Chugun A, Nakaya H, Kondo H. Tonic block of the sodium and calcium currents by ketamine in isolated guinea pig ventricular myocytes. J Vet Med Sci. 1998; 60:479–483. PMID: 9592721.


10. Kanmura Y, Yoshitake J, Casteels R. Ketamine-induced relaxation in intact and skinned smooth muscles of the rabbit ear artery. Br J Pharmacol. 1989; 97:591–597. PMID: 2758233.


11. Yamazaki M, Ito Y, Kuze S, Shibuya N, Momose Y. Effects of ketamine on voltage-dependent Ca2+ currents in single smooth muscle cells from rabbit portal vein. Pharmacology. 1992; 45:162–169. PMID: 1332085.


12. Kapur S, Seeman P. NMDA receptor antagonists ketamine and PCP have direct effects on the dopamine D(2) and serotonin 5-HT(2) receptors-implications for models of schizophrenia. Mol Psychiatry. 2002; 7:837–844. PMID: 12232776.


13. Kim HS, Park IS, Park WK. NMDA receptor antagonists enhance 5-HT2 receptor-mediated behavior, head-twitch response, in mice. Life Sci. 1998; 63:2305–2311. PMID: 9877220.


14. Winter JC, Doat M, Rabin RA. Potentiation of DOM-induced stimulus control by non-competitive NMDA antagonists: a link between the glutamatergic and serotonergic hypotheses of schizophrenia. Life Sci. 2000; 68:337–344. PMID: 11191649.
15. Watts SW. 5-HT in systemic hypertension: foe, friend or fantasy? Clin Sci (Lond). 2005; 108:399–412. PMID: 15831089.


16. Noh HJ, Bae YM, Park SH, Kim JG, Kim B, Kim YS, Kim SH, Cho SI, Woo NS. The vasodilatory effect of ketamine is independent of the N-methyl-D-aspartate receptor: lack of functional N-methyl-Daspartate receptors in rat mesenteric artery smooth muscle. Eur J Anaesthesiol. 2009; 26:676–682. PMID: 19352184.


17. Kim YH, Ahn DS, Joeng JH, Chung S. Suppression of peripheral sympathetic activity underlies protease-activated receptor 2-mediated hypotension. Korean J Physiol Pharmacol. 2014; 18:489–495. PMID: 25598663.


18. Banes AK, Watts SW. Upregulation of arterial serotonin 1B and 2B receptors in deoxycorticosterone acetate-salt hypertension. Hypertension. 2002; 39:394–398. PMID: 11882579.


19. Sung DJ, Noh HJ, Kim JG, Park SW, Kim B, Cho H, Bae YM. Serotonin contracts the rat mesenteric artery by inhibiting 4-aminopyridine-sensitive Kv channels via the 5-HT2A receptor and Src tyrosine kinase. Exp Mol Med. 2013; 45:e67. PMID: 24336234.


20. Domino EF, Zsigmond EK, Domino LE, Domino KE, Kothary SP, Domino SE. Plasma levels of ketamine and two of its metabolites in surgical patients using a gas chromatographic mass fragmentographic assay. Anesth Analg. 1982; 61:87–92. PMID: 7198883.


21. Lu R, Alioua A, Kumar Y, Kundu P, Eghbali M, Weisstaub NV, Gingrich JA, Stefani E, Toro L. c-Src tyrosine kinase, a critical component for 5-HT2A receptor-mediated contraction in rat aorta. J Physiol. 2008; 586:3855–3869. PMID: 18599541.
22. Smith JR, Kim C, Kim H, Purdy RE. Precontraction with elevated concentrations of extracellular potassium enables both 5-HT1B and 5-HT2A "silent" receptors in rabbit ear artery. J Pharmacol Exp Ther. 1999; 289:354–360. PMID: 10087024.
23. Kim SH, Bae YM, Sung DJ, Park SW, Woo NS, Kim B, Cho SI. Ketamine blocks voltage-gated K+ channels and causes membrane depolarization in rat mesenteric artery myocytes. Pflugers Arch. 2007; 454:891–902. PMID: 17342532.
24. Bae YM, Kim A, Kim J, Park SW, Kim TK, Lee YR, Kim B, Cho SI. Serotonin depolarizes the membrane potential in rat mesenteric artery myocytes by decreasing voltage-gated K+ currents. Biochem Biophys Res Commun. 2006; 347:468–476. PMID: 16828462.
25. Cogolludo A, Moreno L, Lodi F, Frazziano G, Cobeño L, Tamargo J, Perez-Vizcaino F. Serotonin inhibits voltage-gated K+ currents in pulmonary artery smooth muscle cells: role of 5-HT2A receptors, caveolin-1, and KV1.5 channel internalization. Circ Res. 2006; 98:931–938. PMID: 16527989.
26. Arévalo-León LE, Gallardo-Ortíz IA, Urquiza-Marín H, Villalobos-Molina R. Evidence for the role of alpha1D- and alpha1A-adrenoceptors in contraction of the rat mesenteric artery. Vascul Pharmacol. 2003; 40:91–96. PMID: 12646397.
27. Hussain MB, Marshall I. Alpha(1)-adrenoceptor subtypes mediating contractions of the rat mesenteric artery. Eur J Pharmacol. 2000; 395:69–76. PMID: 10781676.
28. Yousif M, Kadavil EA, Oriowo MA. Heterogeneity of alpha 1-adrenoceptor subtypes mediating noradrenaline-induced contractions of the rat superior mesenteric artery. Pharmacology. 1998; 56:196–206. PMID: 9566021.
29. Lee HM, Hahn SJ, Choi BH. Blockade of Kv1.5 channels by the antidepressant drug sertraline. Korean J Physiol Pharmacol. 2016; 20:193–200. PMID: 26937216.


30. Lee HM, Hahn SJ, Choi BH. Blockade of Kv1.5 by paroxetine, an antidepressant drug. Korean J Physiol Pharmacol. 2016; 20:75–82. PMID: 26807026.


Fig. 1
Effects of ketamine on the tone of arterial rings in the absence and presence of 5-hydroxytryptamine (5-HT) or norepinephrine (NE).
(A) Upper panel represents a typical trace showing the effect of ketamine on the arterial tone in the presence of 5-HT (200 nM). Lower panel summarizes the effect of ketamine on the arterial tone in the presence of 5-HT (100~200 nM). (B) Upper panel represents a typical trace showing the effect of ketamine on the arterial tone in the presence of NE (10 nM). Lower panel summarizes the upper panel experiments. (C) Upper panel represents a typical trace showing the effect of ketamine on the arterial tone in the absence of 5-HT and NE. Lower panel summarizes the upper panel experiments.
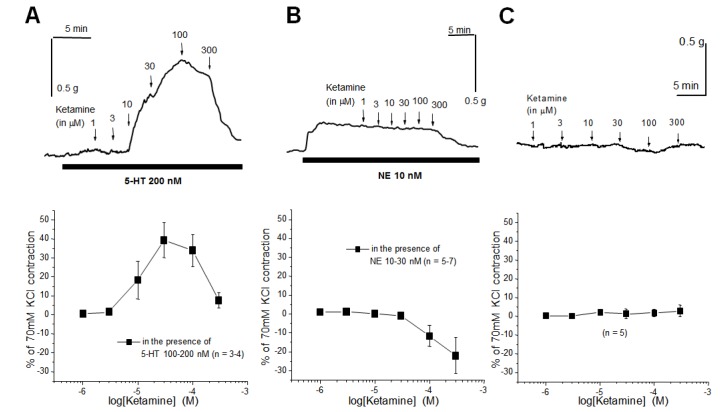
Fig. 2
Effect of ketamine on the concentration-response (contraction) curve (CRC) of 5-HT and NE.
(A, B) CRCs of 5-HT in the absence and presence of 30 and 300 µM ketamine, respectively. (C, D) CRCs of NE in the absence and presence of 30 and 300 µM ketamine, respectively.
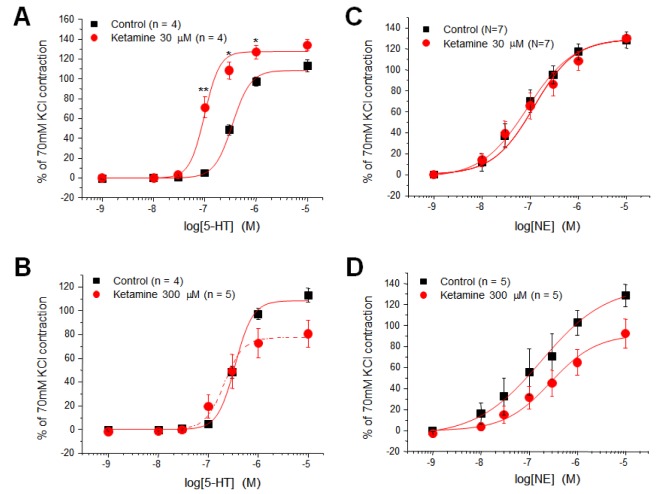
Fig. 3
Effects of ketanserin, anpirtoline, and BW723C86 on the ketamine-induced facilitation of vasoconstriction.
(A) Ketanserine, a potent competitive inhibitor of 5-HT2A receptors, similarly shifted the CRCs of 5-HT in the absence and presence of ketamine (30 µM). (B) Anpirtoline and BW723C86, which are agonists of 5-HT1B and 5-HT2B receptors, respectively, failed to contract the mesenteric arterial rings both in the presence and in the absence of ketamine (30 µM).
