INTRODUCTION
There are different clinical restorative techniques available that are used to replicate the natural tooth structure. The incremental layer build-up technique consists of utilizing layers of different resin composites to build-up the restoration in order to better mimic the original tooth appearance. The double-layer build-up technique is one of the simplest and most common clinical techniques, where the dentin shade composite is applied to approximate the area up until the dentin-enamel junction and then the enamel shade composite is applied with similar thickness to that of natural enamel, with the goal of returning the original contour of the lost tissue [
1]. However, this technique does not always guarantee an acceptable color match for all clinical situations [
1234]. For some types of dental composites, the thickness of the enamel layer needs to be reduced or increased in order to reproduce the optical properties of the natural tooth and create a similar color appearance [
5].
The multi-layer technique allows for the reproduction of personal characteristics and can mimic natural optical effects, as well as the proper color stratification of the polychromatic tooth [
4]. Both enamel and dentin can vary in thickness over the surface of the tooth. Generally, the thickness of enamel is greater at the occlusal/incisal edge of the tooth, which creates the translucent incisal edge in young incisors and, sometimes, the perception of the dentin mamelons underneath [
6]. The dentin thickness, on the other hand, is increased in the cervical portion of the tooth. This creates the polychromatic appearance of the crown, in which, the cervical portion looks darker due to the presence of thicker dentin and thinner enamel [
7] and the incisal portion looks lighter or more translucent due the presence of thicker enamel with little to no dentin [
6].
Not all the different types of dental composite are able to scatter light in the same way as the tooth itself [
3]. More important than choosing the right color for the dentin and enamel layers is layering the dental composites in a way that mimics the differences in characteristics between enamel and dentin, which can affect the way the color is perceived [
89].
As the differences in the layering thicknesses seem to clinically affect the way the light is transmitted and, as a consequence, also affect the way the color is perceived [
5], the purpose of this
in vitro observational study was to compare the difference in the light-transmittance among natural dental tissues and 4 different types of dental composites (nanofilled, supra-nanofilled, microfilled, and microhybrid). This was done by utilizing the incremental layering build-up technique and varying the enamel layer thicknesses to 0.3, 0.5, and 1.2 mm and varying the dentin layer to compensate for an overall thickness of 3.7 ± 0.1 mm. The tested null hypothesis in this study was that there is no difference in light-transmittance among natural dental tissues and different dental composites, regardless of the final enamel layer thickness.
MATERIALS AND METHODS
Sample preparation
One hundred fifty samples were equally distributed into 15 experimental groups (
n = 10): dental tissue (DT); nanofilled dental composite (DC1); microfilled dental composite (DC2); microhybrid dental composite (DC3); supra-nanofilled dental composite (DC4), for each distinct final enamel resin layer (FERL) thickness of 0.3, 0.5, and 1.2 mm. The types of dental composites tested are described in
Table 1 [
1011121314]. Layer thickness variations were selected according to the maximum and minimum reported thickness of natural enamel, which varies from 0.3 to 1.2 mm [
15].
Table 1
Dental composites tested and its composition according to manufacturers
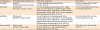
Composite type |
Commercial name (manufacturer) |
Resin matrix composition |
Filler content |
Microfilled |
Durafill VS (Heraeus Kulzer, Hanau, Germany) |
5–10 wt% triethylene glycol dimethacrylate (CAS No. 109-16-0) |
51 wt% pre-polymerized silica filler (10–20 µm; 0.02–2 µm) |
Microhybrid |
Herculite XRV (Kerr Dental, Orange, CA, USA) |
5–10 wt% diurethane dimethacrylate (CAS No. 72869-86-4); 5–10 wt% bisphenol A polyethylene glycol diether dimethacrylate (CAS No. 41637-38-1); 5–10 wt% hexanediol dimethacrylate (CAS No. 6606-59-3); 5–10 wt% triethylene glycol dimethacrylate (CAS No. 109-16-0) |
79 wt% of barium glass filler (0.4 µm) and silica filler (20–50 nm) |
Nanofilled |
Z350 Supreme (3M ESPE, St. Paul, MN, USA) |
5–15 wt% bisphenol A polyethylene glycol diether dimethacrylate (CAS No. 41637-38-1); 5–15 wt% diurethane dimethacrylate (CAS No. 72869-86-4); 1–10 wt% bisphenol A diglycidyl ether methacrylate (CAS No. 1565-94-2); < 5 wt% triethylene glycol dimethacrylate (CAS No. 109-16-0) |
65–75 wt% ceramic filler (4–11 nm); 5–10 wt% silica filler (20 nm) |
Supra-nanofilled |
Estelite Omega (Tokuyama, Tokyo, Japan) |
10–30 wt% bisphenol A diglycidyl ether methacrylate (CAS No. 1565-94-2); 5–10 wt% triethylene glycol dimethacrylate (CAS No. 109-16-0) |
78–82 wt% ceramic and silica filler (200 nm) |
For the control group (DT), 10 unerupted, unidentified, extracted human third molars were randomly selected from the Iowa Institute of Research at the University of Iowa College of Dentistry and Dental Clinics. The human subjects research application of this study was submitted to the Institutional Review Board from the Human Subjects Office at the University of Iowa College of Dentistry and Dental Clinics under protocol ID 201510773. Unerupted molars were used as control to exclude carious lesion, presence of tertiary dentin, extrinsic discoloration, and wear. After cleaning, color measurements were taken using a pre-calibrated spectrophotometer (Easyshade V, Vita Zahnfabrik, Bad Sackingen, Germany). All natural samples selected for this experiment were shade B1. As illustrated in
Figure 1, all teeth were sectioned cross-sectionally (bucco-lingually) into 1.5 mm thick segments (Accutom-5, Struers, Ballerup, Denmark). From the occlusal portion, the average thickeness of each sample (enamel/dentin) was 3.7 ± 0.1 mm. Only the central portions of each tooth were used as the control group. The samples with enamel thickness of 0.5 mm and 0.3 mm were produced by grinding the enamel on wet 400 grit silicon carbide paper (Buehler-Met II, Buehler, Coventry, UK) to reduce them from 1.2 mm to their respective thicknesses and varying the dentin layer to compensate for an overall thickness of 3.7 ± 0.1 mm. The samples were then polished on wet 600 grit silicon carbide paper. A light microscope Stemi 2000 (Carl Zeiss, Göttingen, Germany) and an automatic caliper (Mitutoyo Corp., Kawasaki, Japan) were used to verify all measurements of each sample after each procedure.
Figure 1
Displaying materials and methods. (A, B) Teeth samples preparation. (A) Natural teeth being cut; (B) Measurements for the thickness of natural enamel and dentin; (C, D) RBCs samples. (C) Estelite Omega Custom Shade Guide (Tokuyama America Inc.); (D) Measurements for the thickness of enamel and dentin composite layers; (E, F) Light-transmittance analysis. (E) Sample placed at the center of the goniometric rotary stage; (F) Blue laser striking the sample. (G) Experimental set-up used in the light-transmittance analysis.
RBC, resin-based composite.
Samples of the different types of dental composites were created by using the Estelite Omega Custom Shade Guide molds (Tokuyama America Inc., Chicago, IL, USA) to match the same dimensions of the natural teeth slabs. The composite samples were prepared with a distinct thickness of the layer corresponding to the enamel (FERL) of 0.3, 0.5, and 1.2 mm and a varied dentin layer to achieve an overall sample thickness of 3.7 ± 0.1 mm. Dentin and enamel were restored with A2 and B1 shades, respectively. These shades were selected to match the color measurements that were taken of the natural tooth by the pre-calibrated spectrophotometer (Easyshade V, Vita Zahnfabrik). The composites were light cured with the Valo LED Curing Light AF907 (Ultradent Products Inc., South Jordan, UT, USA) to 16 J/cm
2 of energy (1,067 mW/cm
2 for 15 seconds). Polishing of the composites was performed on wet 400 and 600 grit silicon carbide paper. All samples were kept in artificial saliva before readings [
16].
Light-transmittance analysis
RGB lasers were used to detect translucency in different dental composites, human enamel and dentin, based on the fact that translucent objects absorb and reflect light in certain wavelengths. Three wavelengths were used (red, green, and blue), to cover the portion of the electromagnetic spectrum that is visible to the human eye (390–700 nm). The spectrophotometer set-up used for light-transmittance measurements consisted of a laser source, a goniometric rotary stage and a photodiode detector connected to an amplifier-multimeter (34401A, Agilent Technologies, Santa Clara, CA, USA) measuring system. The detector was mounted on a collimator that had an aperture of 5 cm in diameter. The samples were placed at the center of the rotary stage of the spectrophotometer at 0° angle and were oriented in an occlusal-cervical direction. They were irradiated with a RGB laser beam that was placed behind a second collimator. The laser light was aimed at the respective enamel surface and the light that propagated through the sample was detected by the power meter. Five readings were performed for each sample using the 3 specific wavelengths (red laser, 650 ± 10 nm; green laser, 532 ± 10 nm; blue laser, 405 ± 10 nm), with a beam noise level of 1 mW.
After the raw data collection, the mean was calculated and the data was merged to increase the robustness of translucence detection. The light absorbed by each sample was determined by comparing the intensities of transmitted light (
I). The ratio of intensity of the transmitted light (
I) to the intensity of the incident light (
I0) was called light-transmittance (
T) and it was calculated as follows:
Statistical analysis
Descriptive statistics were computed. A 2-way analysis of variance (ANOVA) was performed to examine the interaction between the FERL thickness level and the experimental group on light-transmittance. One-way ANOVA, followed by the post hoc Tukey's Honestly Significant Difference (HSD) test, was conducted to detect the difference in light-transmittance among the dental tissues and the different types of dental composites tested within each FERL thickness and among the different FERL thickness levels within the dental tissues and each type of dental composites. All tests utilized a significance level of 0.05.
RESULTS
Table 2 provides the results of the comparison of light-transmittance among dental tissues and the 4 different dental composites within the 3 different FERL thicknesses levels and among the FERL levels within the dental tissues and each type of dental composites. Light-transmittance by the different experimental groups (dental tissue [DT]; nanofilled [DC1]; microfilled [DC2]; microhybrid [DC3]; supra-nanofilled [DC4]) were measured by RGB lasers.
Table 2
Comparisons of light-transmittance among experimental groups within each level of final enamel resin layer (FERL) thickness

Experimental group |
0.3 mm FERL thickness |
0.5 mm FERL thickness |
1.2 mm FERL thickness |
Natural dental tissues |
1.898 ± 0.054E,a
|
1.552 ± 0.027C,b
|
0.746 ± 0.052B,C,c
|
Nanofilled |
2.963 ± 0.075B,a
|
1.689 ± 0.048B,b
|
0.885 ± 0.053A,c
|
Microfilled |
3.301 ± 0.092A,a
|
1.840 ± 0.111A,b
|
0.819 ± 0.123A,B,c
|
Microhybrid |
2.875 ± 0.058C,a
|
1.472 ± 0.054D,b
|
0.655 ± 0.074C,c
|
Supra-nanofilled |
2.140 ± 0.055D,a
|
1.561 ± 0.087C,b
|
0.680 ± 0.079C,c
|
Results of 2-way ANOVA revealed that there was a significant interaction between the FERL thickness level and the experimental group on light-transmittance (
p < 0.0001). Since the significant interaction existed, subsequent analyses for simple effects were assessed using 1-way ANOVA. Within each level of FERL thickness, the results from 1-way ANOVA revealed that there was a significant effect of technique type on light-transmittance (
p < 0.0001 in each instance). For the 0.3 mm FERL thickness samples, the
post hoc Tukey's HSD test indicated that the mean light-transmittance was significantly different among the dental tissues and 4 different dental composite types tested. The least to greatest mean light-transmittance were recorded as follows: dental tissues, supra-nanofilled, microhybrid, nanofilled, and then microfilled composites. For the 0.5 mm FERL thickness samples, the mean light-transmittance observed in the microfilled composite was significantly greater than that observed in the other 4 groups, while mean light-transmittance observed in the microhybrid composite was significantly lower than that observed in the other 4 groups. Moreover, no significant difference was found between the dental tissues and the supra-nanofilled composite. For the samples with 1.2 mm FERL thickness, the mean light-transmittance observed in the nanofilled composite was significantly greater than that observed in the dental tissues, the microhybrid, and the supra-nanofilled composites, while no significant difference was found between nanofilled and microfilled composites, or between dental tissues and microfilled composite, or among dental tissues, microhybrid, and supra-nanofilled composites. Detailed results for the comparisons of the 5 experimental groups are presented in
Table 2.
Within each experimental group, results from the 1-way ANOVA indicated that a significant effect of FERL thickness levels on light-transmittance was observed (
p < 0.0001 in each instance). For all 5 experimental groups, the
post hoc Tukey's HSD tests indicated that the mean light-transmittance observed in the samples with the 1.2 mm FERL thickness was significantly lower than those observed in the samples with the 0.3 and 0.5 mm FERL thicknesses, while mean light-transmittance observed at the 0.5 mm FERL thickness samples was significantly lower than that observed at the 0.3 mm FERL thickness samples (
Table 2).
In summary, tests for simple effects displayed that no significant difference in light-transmittance existed between the dental tissues and the supra-nanofilled composite at 0.5 mm or at 1.2 mm FERL thickness, while a significant difference was found between the dental tissues and the supra-nanofilled composite at 0.3 mm FERL thickness. Moreover, all experimental groups did show significant increases in light-transmittance when the FERL thickness level decreased.
DISCUSSION
This in vitro observational study assessed the difference in light-transmittance among natural dental tissues and dental composite restorations using the incremental double-layer technique with varying enamel and dentin layer thicknesses. As observed in the results, there were differences in light-transmittance among natural tissues and the different composite types tested according to the thickness of the FERL used with the incremental double-layer technique. It was observed that the light-transmittance through the different types of dental composites was not always equal to the light-transmittance through dental tissues with regard to the thickness of the FERL used in the incremental build-up technique. Thus, the null hypothesis of this study, that there would be no difference in light-transmittance among natural dental tissues and the different dental composites regardless of the FERL thickness, was rejected.
The samples with the thickest enamel resin layer (1.2 mm) had similar light-transmittance through the dental tissues and all dental composites tested, with the exception of the nanofilled composite. On the contrary, as the FERL got thinner, the dental composites that originally had a light-transmittance similar to the dental tissues decreased. Within the 0.5 mm FERL thickness samples, only the supra-nanofilled composite showed similar light-transmittance to the dental tissues, while within the 0.3 mm FERL thickness samples, none of the composites had similar light-transmittance to that of the dental tissues.
In this study, commercial composites were used from multiple manufacturers. As the exact composition of these products is not possible to confirm, this can be described as a limitation of the study. However, it is known that the factor which most influences the optical properties of composites is the concentration and particle size of the filler [
17]. The different types of resin composites have different types and amounts of filler in their composition [
18]. As described in
Table 1, the microfilled composites contain filler particles with sizes in the micron scale, compared to microhybrids which have particles with sizes in both the micron and the macro scales. The combination of filler particles of different sizes allows for a higher concentration of filler in the microhybrid composite, in comparison to the microfilled composite. The nanofilled composite, on the other hand, integrates a high concentration of filler with particle sizes in the nanometric scale. These silica fillers tend to agglomerate, thereby producing larger effective particles [
1920]. Due to advances in technology, the supra-nanofilled composites have non-agglomerated silica particles with standardized sizes in the nanometric scale [
1017]. The supra-nanofilled composites allow for an even higher concentration of filler particles as compared to the nanofilled composite.
The characteristics of filler particles from commercial dental composites vary widely in size, shape and content [
18]. But, although the most common current classification of dental composites is exclusively based on particle size, physical properties are not necessarily exclusively correlated to particle size [
916]. Instead, physical properties can be correlated to filler content and distribution [
89181921].
Light-transmittance is expected to be reduced as the filler content increases [
822]. This reduction occurs due to light scattering differences between the refractive index of the resin matrix and the refractive index of the filler particles [
923]. The effect of organic and inorganic matrix on optical properties of dental composites is associated with the refractive index of each specific material and particular formulation, that commonly ranges from 1.45 to 1.55 ± 0.20. It is expected that the greater mismatching between the refractive index of the resin matrix and filler particles, the lower translucency of the dental composite. Also, dental composites with higher filler content have higher scattering of light through the material [
921]. However, an important exception is the nanofilled composites. Light scattering occurs when the size of the filler particle is greater than or equal to the wavelength of the incoming visible light, which will be between 400 and 750 nm. The wavelength, however, is about ten times larger than the nanoparticles present in nanofilled composites [
19]. This would suggest minimal light scattering and higher light-transmittance for these kind of composites, such as the nanofilled and supra-nanofilled composites tested in this study. However, as silica particles tend to agglomerate, creating larger particles, it is expected that the light shall be scattered reducing light-transmittance through the nanofilled composites [
1920] in comparison to the supra-nanofilled composites, in which silica particles do not tend to agglomerate [
1017]. The non-agglomeration phenomenon of the latter seems to be related to its development process, which is based on the sol-gel method that both controls the diameter and the refractive index of the fillers [
1017] and increases light-transmittance [
17].
It is important to notice that in general, the mean light-transmittance observed at thicker FERLs was significantly lower than that observed at thinner FERLs, regardless of the type of dental composite tested (
Table 2). It can be explained due the increase of light scattering through the superficial and sub-superficial composite. Also, a greater light absorption and reflection when used a thicker dentin layer, which would reduce the light-transmittance through the samples, regardless of the material's composition and category used in this study. Numerous factors such as organic and inorganic matrix, respective refractive index before and after polymerization, pigments, and filler content, size and arrangement influence directly the light transmittance and opacity of dental composites. These factors must be thoroughly investigated in order to understand the light propagation in dental composites.
The clinical significance of this study is that differences in light-transmittance through dental composites and dental tissues affect the color matching capabilities of restorations [
5]. Despite the fact that incremental layering seems to be effective in reproducing light-transmittance similar to dental tissues, the thickness of the FERL, as well as the type of composite, are important factors that can influence the light-transmittance pattern. Thus, clinicians should be aware that the final thickness of enamel resin layers may need to be varied with different types of dental composites in order to reproduce the same shade and provide esthetic restorations.