Abstract
PURPOSE
This study was to evaluate the effect of grinding of the inner metal surface during the porcelain try-in stage on metal-porcelain bonding considering the maximum temperature and the vibration of samples.
MATERIALS AND METHODS
Ninety-one square prism-shaped (1 × 1 × 1.5 mm) nickel-chrome cast frameworks 0.3 mm thick were prepared. Porcelain was applied on two opposite outer axial surfaces of the frameworks. The grinding was performed from the opposite axial sides of the inner metal surfaces with a low-speed handpiece with two types of burs (diamond, tungsten-carbide) under three grinding forces (3.5 N, 7 N, 14 N) and at two durations (5 seconds, 10 seconds). The shear bond strength (SBS) test was performed with universal testing machine. Statistical analyzes were performed at 5% significance level.
RESULTS
The samples subjected to grinding under 3.5 N showed higher SBS values than those exposed to grinding under 7 N and 14 N (P<.05). SBS values of none of the groups differed from those of the control group (P>.05). The types of bur (P=.965) and the duration (P=.679) did not affect the SBS values. On the other hand, type of bur, force applied, and duration of the grinding affected the maximum temperatures of the samples, whereas the maximum vibration was affected only by the type of bur (P<.05).
For decades, metal-porcelain restorations have represented the majority of tooth-colored laboratory-fabricated restorations due to their low cost when compared with metal-free restorations, easy cementation technique, and excellent clinical performance. As a result of the high cost of precious alloys and advances in porcelain technology, the use of nickel-chrome (Ni-Cr) and cobalt-chrome (Co-Cr) based alloys as framework materials for fixed dental prostheses (FDPs) has increased considerably.1 These alloys allow excellent quality treatments due to their satisfactory mechanical properties at less thickness and their hardness, elasticity, and tensile strength compared with precious alloys and all ceramic systems.1,2,3
In metal-porcelain restorations, corruption of the initial fit of the metal framework has been reported after the porcelain firing cycle and observed clinically as a marginal discrepancy.4,5,6 Gemalmaz and Alkumru7 attributed marginal discrepancies to porcelain-metal thermal contraction mismatch, the design of the margin, and the type of alloy used. Whatever the cause of such distortion, if not corrected, it will result in bacterial plaque accumulation, followed by caries and gingival inflammation.8 To correct these distortions, restorations can be evaluated and adjusted internally by grinding in the preclinical phase. However, such internal adjustment with stone dies does not guarantee that the final intraoral fit due to possible wear of the stone dies.9 Thus, the internal fit must be verified clinically at the porcelain try-in stage. A variety of materials can be used for this purpose, such as a film of chloroform and rouge, a fit checker, thinned typewriter correction fluid, disclosing wax, a dry aerosol indicator and an irreversible hydrocolloid.10 Any premature contact detected can be grinded with a handpiece in the clinical porcelain try-in stage.
To modify the prosthesis quickly, grinding can be performed rapidly under high force with coarse burs. The structure of the porcelain can be damaged by the grinding because of factors such as removal forces, material removal mechanisms, and surface/subsurface damage.11
Over the past years, under the closest oral conditions, several authors have evaluated the metal-porcelain bond strength. Various tests have evaluated properties such as color stability,12 shear,1 tensile,13 flexural,14,15 and torsional strength16 of metal-porcelain FDPs. The aim of all these studies was to improve the metal-porcelain bond strength.17 Although grinding at the try-in stage may weaken the restoration, there have been no studies of the effects of metal surface grinding on metal-porcelain bonding. In particular, the effect of grinding performed after porcelain firing on the metal-porcelain restoration is still unknown.
The purpose of this study was to evaluate the effect of inner metal surface grinding on the metal-porcelain bond strength with shear bond strength (SBS) test while considering the maximum temperature and the vibration of the samples.
Four stainless steel templates were produced with a milling machine (TMC-500-V CNC, Taksan, Kayseri, Turkey) for the different stages of this study. First template (Fig. 1) has 1 cm3 cubic process and used for producing standard metallic framework. The second template (Fig. 2) was fabricated 20% larger than desired porcelain dimensions to compensate for porcelain shrinkage. The third template was used to verify the desired dimensions of the porcelain. The last template (Fig. 3) was used during the grinding protocol and contained the samples and the sensors.
Template #1 (Fig. 1) was duplicated with an agar-agar hydrocolloid (Megafeel, Megadental, Büdingen, Germany) and investment material (Bellavest, BEGO, Bremen, Germany). For fabricating the samples, a 0.3 mm thick wax plate (SEL-WAX, Arge Dental, Ankara, Turkey) was wrapped around the axial surfaces of the duplicates. The upper surfaces of the duplicates were filled to 5 mm with wax (Fig. 4A). These wax patterns were then mounted in a silicone ring and poured with the same investment material following the manufacturer's recommendation.
After the investment material set, the silicone ring and the sprue former were separated from the investment mold. The metallic frameworks were cast in Ni-Cr alloy (Rubyalloy, Rubydent, Istanbul, Turkey) in an electrical induction furnace (Infratherm-II AT, GMG Electronic, Istanbul, Turkey). Carbide discs at low speed and air-particle abrasion with 125 µm Al2O3 under 2 bar pressure (EasyBlast&Perlablast, BEGO, Bremen, Germany) were used to eliminate the sprues and separate the metallic strips before the application of the porcelain (Fig. 4B).
The metal framework samples were cleaned in an ultrasonic bath and completely dried. Bonding agent (3C-BOND, Ceka Attachments Preci-Line, Waregem, Belgium) was then applied in the center of two opposite exterior axial surfaces and fired (Vacumat 2500 Premium Line, VITA, Bad Säckingen, Germany) prior to the application of the porcelain. A commercial leucite glass-ceramic (Ceramco 3, Dentsply, Philadelphia, USA) was used in this study. Two layers of opaque ceramic (wash and powder opaque) were applied according to the manufacturer's recommendations.
To simulate the critical porcelain thickness,12 1 mm thick porcelain layer was generated in this study. Template #2 (Fig. 2) was used to standardize the dimensions of the unfired porcelain (1.2 × 6 × 6 mm) in the center of the axial outer surface of the sample. All the firing procedures are illustrated in Table 1. After cooling the samples, template #3 was used to verify the desired dimensions (1 × 5 × 5 mm) of the porcelain. Any part of the porcelain not compatible with template #3 was adapted with a diamond bur at low speed. The procedures were performed by the same operator to standardize the process.
Ninety-one samples were divided into 13 groups (n=7) (Table 2). The first group served as the control and was not subjected to grinding. The grinding was performed from both opposite axial sides of the inner metal surfaces of each sample. The effect of the type of bur, the grinding force (3.5 N, 7 N, 14 N), and the duration (5 seconds, 10 seconds) on bond strength was evaluated. A black-coded diamond bur (a-diamant, Acurata, Thurmansbang, Germany) and a tungsten-carbide bur (Imperial, Acurata, Thurmansbang, Germany) with a round diameter and ISO 18 burs were used as different types of abrasives. The grinding force values used were those developed by ourselves and based on a pilot study with a precision scale (HT300NH, Dikomsan, Istanbul, Turkey). To simulate the grinding forces under light, medium, and high force, 3.5 N, 7 N and 14 N were used, respectively. These values were achieved by adding weights on the upper part of the parallelometer (Paraskop M, Model No. 26060, Bego, Bremen, Germany). The duration of the grinding (5 and 10 seconds) was selected randomly.
To standardize the grinding of the inner metal surface of the metal-porcelain samples, a low-speed handpiece (IS-205 &S-Max M65, NSK, Tochigi, Japan) was mounted on the upper part of the parallelometer (Fig. 3). All the grinding was performed at 25,000 rpm. To simulate the tactile sense, an elastomeric impression material (Impregum Penta Soft, 3M ESPE, Seefeld, Germany) approximately 1.5 mm thick was formed between template #4 and samples, for holding the samples. The thermocouple sensor (E-RTO9-1P-04, Elimko, Ankara, Turkey) was mounted on this template, and the vibro analyzer sensor (4524 - Triaxial CCLD piezoelectric accelerometer, Brüel & Kjær, Nærum, Denmark) was mounted on the samples. The vibration and thermal data were obtained from experimental setup shown in Fig. 3.
After the grinding procedures, the samples were overglazed according to the Ceramco 3 finishing procedure. Zinc polycarboxylate cement (Adhesor Carbofine, Spofa Dental, Bioggio, Switzerland) was mixed according to the manufacturer's recommendations and applied with a thin brush onto the grinded surfaces. The internal space of the samples was then filled with pattern resin (Pattern Resin LS, GC, Leuven, Belgium) to simulate cemented fixed prostheses.
Six thousand thermal cycles (Termalsiklus, Dentall Teknik, Konya, Turkey) were performed (bath time 25 seconds, transfer time 5 seconds, bath temperatures 5℃ and 55℃). All the samples were loaded with a crosshead speed of 0.05 mm/min until fracture with a universal testing machine (Instron, Canton, MA, USA).
Statistical analyzes were performed with SPSS 20.0 (IBM Corporation Software Group, Armonk, NY, USA). The data were evaluated first with the Kolmogorov-Smirnov normality test. The normal distributed SBS data were then tested with three-way ANOVA and Bonferroni's posthoc test. The abnormal distribution of maximum vibration and temperature data were tested with the Mann-Whitney U test, the Kruskal-Wallis test, and Friedman's test to compare values between the groups, with 95% confidence interval.
Adhesive failure occurred in all the samples in group D/14/10 during the grinding. Hence, this group was removed from the study. According to the results of the ANOVA, the SBS of the metal-porcelain system was affected by the force applied (P<.001) but not by the type of bur (P=.965) or duration of the grinding (P=.679) (Fig. 5). The samples exposed to grinding at 3.5 N (21.431 ± 5.83) showed a higher SBS than those subjected to 7 N (18.269 ± 4.67) and 14 N (17.705 ± 4.31)(P<.05), but the SBS did not differ from that of the control (18.256 ± 3.57)(P>.05). On the other hand, there was no statistically significant difference between the samples subjected to grinding at 7 N and 14 N and the control (P>.05).
All three factors (the type of bur, the force applied, and the duration of the grinding) significantly affected the maximum temperatures of the samples (Fig. 6). The samples subjected to grinding with the diamond bur (35.46 ± 4.2) showed a higher temperature than those exposed to grinding with the tungsten-carbide bur (33.87 ± 2.2)(P=.003). The samples subjected to grinding for 10 seconds (35.45 ± 3.3) displayed a higher temperature than those that underwent grinding for 5 seconds (33.87 ± 3.2)(P=.010). According to the pairwise comparison of the force applied (P<.001), grinding at 3.5 N (32.13 ± 2.5) was associated with a lower maximum temperature than grinding at 7 N (35.61 ± 3.7) or 14 N (36.51 ± 1.6)(P<.05). There was no difference in the maximum temperatures of the samples for grinding at 7 N and 14 N (P>.05).
According to the results of Friedman's test, the z axis (35.88 m/sn2 ± 20.8) showed lower vibrations than the x (60.42 m/sn2 ± 29.0) and y (57.47 m/sn2 ± 23.7) axes (P<.001). Although there was no difference in the vibration values of the x and y axes (P>.05), the vibration values on the x axis was chosen because of the relatively high average of values. According to the results of the analyses of the maximum vibration on the x axis (Fig. 7), the tungsten-carbide bur (76.51 ± 20.0) produced more vibration than the diamond bur (41.11 ± 26.2)(P<.001). However, neither duration of the grinding (P=.307) nor the force applied (P=.135) affected the maximum vibration on the x axis (Fig. 8).
The fit of the inner surfaces of metal-porcelain FDPs to abutments is a primary requirement for a successful restoration. Although metal copings fit well at the framework try-in stage, metal-porcelain FDPs may not fit at the porcelain try-in stage. Few studies have investigated this problem. Investigators have attributed this issue to reduced metal collar thickness,4 thermal coefficient mismatch, margin design,5 and margin spee.6 Whatever the cause of the distortion, the mismatch of the restoration-abutment complex has to be corrected because an unacceptable marginal gap could result in cement washout,12 with subsequent biological complications, such as secondary caries, periodontal problems and pulpitis.13,14
Grinding the inner surfaces of metal-porcelain is an easy and effective way to correct FDP misfits at the porcelain try-in stage. In the present study, this stage was simulated without water cooling and with samples that had minimum metal and porcelain thicknesses and porcelain thicknesses allowed in dental practice.2 Thus, according to the authors of this study, microcracks and microfractures may occur more frequently in situations where the metal and the porcelain is so thin.
Song et al.11 investigated the effects of rapid grinding of the porcelain surface and concluded that caution should be observed during rapid clinical adjustments because greater forces and force ratios, higher specific removal energy, and severe surface and subsurface damage can be induced in dental practice. However, there has been no study about the effects of grinding of the inner surface of metal-porcelain on the resulting bond strength.
ISO 969315 is a flexural standardization test of the bonding strength of the metal-porcelain complex. However, some authors reported that flexural tests are not sufficient to determine the bonding strength because of the influence of the modulus of elasticity of the metal alloy on the strength values obtained. In addition, in the SBS test, the bond strength may be measured directly from the metal-porcelain interface.17 The metal-porcelain thicknesses and the grinding process in the present study are not compatible with the ISO protocol. Thus, the bond strengths of the samples were measured with a customized SBS test method. Additionally, the maximum temperature and the vibration data during the grinding were recorded for possible interrelation between these two parameters.
According to the results of this study, grinding the inner surfaces of the metal-porcelain complex did not affect the metal-porcelain bond strength when compared with the control group. This may be due to the overglazing of the samples. Overglazing is the last stage of routine laboratory process of metal-porcelain FDPs. Overglazing can produce smooth and shiny FDPs. It also has a positive effect on the strength of the porcelain by reducing the number of flaws present on the surface of the FDP.18,19,20 The 7 N and 14 N groups showed reduced bond strength compared to the 3.5 N group. According to this result, if grinding is needed at the porcelain try-in stage, it should be performed at low forces.
The maximum temperature and the vibration values of the samples during the grinding were recorded in the present study. We preferred maximum values because any possible bond strength failure between the metal and the porcelain layer may occur at these peak values. According to the results for the maximum temperature of the samples, as expected, increasing the duration of grinding resulted in higher temperatures because of increased friction. In addition, the diamond bur was associated with higher temperatures than the tungsten-carbide bur. This may be due to the surface topography of the diamond bur, which has more contact area during grinding than the tungsten-carbide bur.
The evaluation of the effect of the grinding force factor on the maximum temperature of the samples showed that the 3.5 N group showed less of a temperature increase than the 7 N and the 14 N groups. However, there was no statistical difference between the 7 N and the 14 N group. As noted earlier, the D/14/10 group was not included in the statistical analyses because the burs of this group became incandescent, causing porcelain layer debonding. The results suggest that increasing the grinding force generates a higher temperature and that porcelain debonding may occur beyond a critical temperature limit.
All the burs used in the present study were unused. However, a bur may be used several times in a dental practice. Thus, prolonged duration and increased force may be required with used burs to achieve a similar amount of grinding. In this situation, the critical temperature limit may be reached earlier. Thus, care must be exercised when used burs are employed.
In the present study, the burs were in contact with the samples before grinding, and the contact continued during grinding. In this situation, the first rotation of the bur causes a sudden shock-like effect. Thus, in all the vibration recordings, the maximum vibration values occurred at the first rotation. After the first rotation, the vibration values decreased and showed similar curves (Fig. 7). Thus, the duration of the grinding (5 seconds, 10 seconds) did not affect the maximum vibration values. In addition, different forces (3.5 N, 7 N, 14 N) may produce similar vibration values because of the ongoing contact. In contrast to the duration and the force of the grinding, the type of bur used affected the maximum vibration values, with the tungsten-carbide burs vibrating the samples more than the diamond burs. This may be due to the aggressive topography of the tungsten-carbide burs.
All three factors (type of bur, grinding force, grinding duration) evaluated in this study affected the maximum temperature. Among these three factors, only the type of bur affected the maximum vibration. However, none of these factors affected the SBS when compared with the control group. Thus, the maximum temperature and the vibration of the samples did not affect the SBS.
The findings of the present study seem to have important relevance for the effect of grinding on the metal-porcelain bond strength at the porcelain try-in stage. However, some limitations should be considered. First, the size and the shape of the samples are not the same as those used clinically and may not indicate actual clinical performance. Second, Ni-Cr alloy and one type of commercial porcelain (Ceramco 3) were used as restorative materials in the present study. The effect of different types and brands of restorative materials should be researched in further studies. A low-speed handpiece at 25,000 rpm was used in the present study. The effect of different rotations per minute on the SBS should be investigated in other studies. Further in vitro long-term studies using mechanical fatigue tests on teeth restored with metal-ceramic crowns, in addition to prospective clinical studies, should be conducted to yield more clinically relevant results. The grindings on the porcelain layer for standardizing dimensions were not considered in this study. Further studies may focus on effects of grinding on porcelain layer to the bond strength of metal porcelain fixed dental prostheses.
Under the conditions in this study, grinding the inner surface of metal-porcelain FDPs at the porcelain try-in stage did not affect the bond strength. Although the grinding affected the maximum temperature and the vibration values of the samples, it did not influence the bonding strength. The authors recommend not exceeding 5 seconds when using high grinding force with diamond bur on the inner surface of metal-porcelain fixed prostheses at the porcelain try-in stage.
Figures and Tables
![]() | Fig. 2Standardizing unfired porcelain (b) forming by sliding template #2 (a) on metallic framework (c) vertically (A) and horizontally (B). |
![]() | Fig. 7Grinding vibration graphs for the x axes (Maximum vibration values were pointed out with arrows). |
Table 1
Firing procedures performed in this study
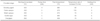
References
1. Lombardo GH, Nishioka RS, Souza RO, Michida SM, Kojima AN, Mesquita AM, Buso L. Influence of surface treatment on the shear bond strength of ceramics fused to cobalt-chromium. J Prosthodont. 2010; 19:103–111.
2. Shillingburg HT, Hobo S, Whitsett LD, Jacobi R, Brackett SE. Fundamentals of Fixed Prosthodontics. 3rd ed. Chicago: Quintessence Publishing Co., Inc.;1997. p. 455–484.
3. do Prado RA, Panzeri H, Fernandes Neto AJ, das Neves FD, da Silva MR, Mendonça G. Shear bond strength of dental porcelains to nickel-chromium alloys. Braz Dent J. 2005; 16:202–206.
4. Shillingburg HT Jr, Hobo S, Fisher DW. Preparation design and margin distortion in porcelain-fused-to-metal restorations. J Prosthet Dent. 1973; 29:276–284.
5. Faucher RR, Nicholls JI. Distortion related to margin design in porcelain-fused-to-metal restorations. J Prosthet Dent. 1980; 43:149–155.
6. Tao J, Han D. The effect of finish line curvature on marginal fit of all-ceramic CAD/CAM crowns and metal-ceramic crowns. Quintessence Int. 2009; 40:745–752.
7. Gemalmaz D, Alkumru HN. Marginal fit changes during porcelain firing cycles. J Prosthet Dent. 1995; 73:49–54.
8. Papazoglou E, Brantley WA, Johnston WM. Evaluation of high-temperature distortion of high-palladium metal-ceramic crowns. J Prosthet Dent. 2001; 85:133–140.
9. Ushiwata O, de Moraes JV, Bottino MA, da Silva EG. Marginal fit of nickel-chromium copings before and after internal adjustments with duplicated stone dies and disclosing agent. J Prosthet Dent. 2000; 83:634–643.
10. Keys LG. An alternate method of verifying the seating of all-ceramic restorations. J Prosthet Dent. 2002; 87:411.
11. Song XF, Yin L, Han YG, Wang H. In vitro rapid intraoral adjustment of porcelain prostheses using a high-speed dental handpiece. Acta Biomater. 2008; 4:414–424.
12. Kourtis SG, Tripodakis AP, Doukoudakis AA. Spectrophotometric evaluation of the optical influence of different metal alloys and porcelains in the metal-ceramic complex. J Prosthet Dent. 2004; 92:477–485.
13. Aladağ A, Cömlekoğlu ME, Dündar M, Güngör MA, Artunç C. Effects of soldering and laser welding on bond strength of ceramic to metal. J Prosthet Dent. 2011; 105:28–34.
14. de Vasconcellos LG, Buso L, Lombardo GH, Souza RO, Nogueira L Jr, Bottino MA, Ozcan M. Opaque layer firing temperature and aging effect on the flexural strength of ceramic fused to cobalt-chromium alloy. J Prosthodont. 2010; 19:471–477.
15. ISO 9693-1:2012. Dentistry--Compatibility testing-Part 1: Metal-ceramic systems. Geneva: Switerland: International Organization for Standardization;2013.
16. Hero H, Syverud M. Carbon impurities and properties of some palladium alloys for ceramic veneering. Dent Mater. 1985; 1:106–110.
17. Hammad IA, Talic YF. Designs of bond strength tests for metal-ceramic complexes: review of the literature. J Prosthet Dent. 1996; 75:602–608.
18. Brackett SE, Leary JM, Turner KA, Jordan RD. An evaluation of porcelain strength and the effect of surface treatment. J Prosthet Dent. 1989; 61:446–451.
19. Leinfelder KF. Porcelain esthetics for the 21st century. J Am Dent Assoc. 2000; 131:47S–51S.
20. Isgrò G, Pallav P, van der Zel JM, Feilzer AJ. The influence of the veneering porcelain and different surface treatments on the biaxial flexural strength of a heat-pressed ceramic. J Prosthet Dent. 2003; 90:465–473.