Abstract
Dogs commonly serve as a model for various human conditions, including periodontal diseases. The aim of this study was to identify the anaerobic bacteria that colonize the subgingival areas in dogs and humans by using rapid real-time polymerase chain reaction (RT-PCR)-based tests and to compare the results obtained in each species. Bacterial microflora evaluations, both quantitative and qualitative, were performed by applying ready-made tests on twelve dogs and twelve humans. Five samples were collected from each subject's deepest gingival pockets and joined to form a collective sample. The results of the study revealed interspecies similarities in the prevalences of Porphyromonas (P.) gingivalis, Treponema denticola, Tannerella forsythia, and Fusobacterium nucleatum. Red complex bacteria comprised the largest portion of the studied bacterial complexes in all study groups, with P. gingivalis being the most commonly isolated bacterium. The results show similarities in the prevalence of bacterial microflora in dogs and humans. Microbiological analysis of gingival pockets by using rapid real-time PCR-based tests in clinical practice, both veterinary and human, can facilitate the choice of appropriate pharmacological treatment and can provide a basis for subsequent verification of the treatment's effectiveness.
Periodontal disease involves chronic inflammation of the periodontal tissues (gingiva, periodontium, cement, and alveolar process) that surround a tooth and maintain its proper alignment in the alveolus. The etiology of periodontal diseases in both dogs and humans has been the subject of numerous studies. In particular, dogs have commonly served as a model for the course of various human conditions, including diseases of the oral cavity [1831].
The main factor responsible for the development of periodontal disease are bacteria [1437], and the oral cavity is the habitat for over 700 bacterial species. Microbial stability (homeostasis) is the result of both synergistic and antagonistic interactions between bacteria, while the condition of the bacterial flora is largely dependent on aerobic bacteria [1337]. Due to microbial aggregation on tooth surfaces, bacteria which naturally inhabit the oral cavity may become pathogenic toward the host organism. Under favorable conditions, normally beneficial bacterial flora coating the surfaces of the tongue, lips, cheeks, and gums in a permanent and unalterable manner, will overcome the host's immune system and penetrate into tissue [2429]. With a progressing periodontal disease, the tooth surfaces are colonized by Gram-negative bacteria. Hindered oxygen diffusion and decreased oxidative and reductive potentials facilitate the growth of anaerobic organisms [21]. As a result of gum swelling and deepening of the gingival pocket, in both dog and human, a subgingival biofilm is formed, which includes colonies of anaerobic, Gram-negative (Bacterioides spp., Capnocytophaga spp., Fusobacterium spp., Porphyromonas spp., Prevotella spp., Tannerella spp., and Treponema spp.) as well as Gram-positive bacteria (Actinomyces spp., Corynebacterium spp., Eubacterium spp., Peptostreptococcus spp., and Streptococcus spp.) [1823].
Micro-organisms are considerably interdependent and form a complex biofilm on the surfaces of the teeth. Microcolonies within the biofilm have the ability to bind to solid surfaces (soft oral tissue, teeth, or other bacteria). Many species of bacteria have surface appendages such as fimbriae, which make it easier for them to adhere to various surfaces [36]. By grouping into complexes, bacteria become more effective in protecting themselves from the host's defense mechanisms and are able to better utilize available nutrients. Socransky et al. [35] have identified a number of biofilm bacterial complexes. Previously reported data indicate that red complex bacteria are the most important to periodontal disease and are considered to be marker bacteria for periodontitis in humans. Red complex bacteria include Porphyromonas (P.) gingivalis, Treponema (T.) denticola, and Tannerella forsytha. The main pathogen responsible for causing periodontitis in humans is P. gingivalis [25]. The virulence factors (such as the ability to destroy immunoglobulins G and A) for P. gingivalis indicate it is a primary pathogen associated with periodontal diseases in human [18]. Another complex significantly influencing the course of periodontal disease is the green complex (e.g., Capnocytophaga [C.] gingivalis, Campylobacter concisus, and Eikenella corrodens). Furthermore, the orange complex (e.g., Fusobacterium [F.] nucleatum, Prevotella intermedia, Peptostreptococcus micros, and Campylobacter rectus) is important because the presence of bacteria from this group determines the formation of red complex bacteria [36]. Colonization of specific bacterial species in the biofilm is a significant etiological contribution to periodontitis, and identification of the pathogens present therein greatly facilitates the choice of proper local and systematic therapy.
While there has been much research into the subgingival bacterial microflora in humans, the same remains considerably less explored in dogs, particularly in terms of anaerobic bacterial microflora [12]. Regardless, in the course of numerous studies, various micro-organisms have been isolated that originate from the subgingival region and actively contribute to the onset of periodontitis [26]. The aim of this study was to identify the anaerobic bacteria that colonize the subgingival regions in both dogs and humans, and to compare the results obtained in each species. By juxtaposing the particular bacteria, we hoped to elucidate further the etiology of periodontal diseases in both humans and canines.
All study protocols were approved by the local ethics committees of the University of Life Sciences, Lublin and the Medical University of Lublin, Poland. Dog owners and human participants were informed of the purpose of the study and gave approval for their participation. The research material was obtained from 24 subjects: 12 dogs (group D) and 12 humans (group H). Both dog and human subjects included in the study were selected based on medical history and clinical examination for systemic diseases. Subjects with diseases of the endocrine system, cancer, or generalized infectious diseases were excluded from the study. The presence of periodontal complications such as apical abscesses, fractured teeth, or other non-infectious conditions excluded subjects from the study. Microbiological analysis for dogs included 6 males aged 5–13 years (Dm) and 6 females aged 9–16 years (Df) of several different breeds, whereas the analysis for humans included 6 males aged 29–60 years (Hm) and 6 females aged 30–63 years (Hf). All subjects had been diagnosed with chronic periodontitis on the basis of a dental examination, which evaluated parameters such as depth of periodontal pockets, clinical attachment level and bleeding on probing. Measurements were made by using a calibrated periodontal probe. Subjects were tested for the presence of periodontitis/peri-implantitis pathogens by using real-time polymerase chain reaction (PCR)-based tests (PET plus; MIP Pharma, Germany). Specifically, the subjects were tested for: Aggregatibacter (A.) actinomycetemcomitans, P. gingivalis, T. denticola, Tannerella forsythia, Prevotella intermedia, Peptostreptococcus micros, F. nucleatum, Eubacterium (E.) nodatum, and C. gingivalis [6739].
Bacterial microflora evaluations, both quantitative and qualitative, were performed by using PET plus (MIP Pharma, Germany) diagnostic tests. Using sterile paper points, five samples were collected from each subject's deepest periodontal pockets (i.e, from five teeth with the deepest periodontal pockets) and were combined to form a collective sample. In dogs, the samples were collected from pockets ≥ 4 mm deep and in humans from pockets ≥ 5 mm deep. Among the subjects, the loss of connective tissue attachment averaged approximately ≥ 1 mm. Among the sampled teeth there was bleeding during probing. All samples for PET plus (MIP Pharma) testing were collected in accordance with the procedure recommended by the manufacturer. Before introducing paper points into a gingival pocket, supragingival plaque was cleared by using sterile cotton pellets (taking care not to induce bleeding). Next, the sites were dried with compressed air. Sterile paper points were introduced by using tweezers and were inserted to the full depth of the sampled pocket for 15 sec. Collectively, 24 samples were harvested, placed in the transport vials provided with the PET plus test kit, and shipped to a MIP Pharma laboratory in Germany, where sample processing was performed. Sample analysis was performed by using real-time PCR, which allows rapid and easy identification of particular micro-organisms. The PCR-based test utilizes fast duplication of selected DNA/RNA strand sections as products and allows quantitative analysis of the copied products within the study's samples. Such testing has high sensitivity as a single strand section can produce up to 106 products. Moreover, it allows analysis of material with a low initial DNA concentration. Free strand sections of DNA were obtained from lysed bacterial cells and were subsequently subjected to amplification and hybridization with fluorescence-stained starters characteristic of particular periopathogens. The PCR probes were designed for human bacteria. Quantitative analysis was performed with a reader that measures the intensity of fluorescence compared to that in reference specimens. According to the manufacturer, the threshold determination for all subjects bacterial pathogens was approximately 103 bacteria. Threshold determination adopted in this research work was approximately 104 bacteria.
The significance of the differences detected between particular groups was assessed by using the Mann-Whitney U test provided as part of Statistica 10.0 software (Quest Software, USA). Statistical significance was accepted for p ≤ 0.05. Descriptive parameters (means and standard deviations) were calculated by using Microsoft Excel software (Microsoft, USA).
The percentages of each respective bacterial group within the total pathogenic bacterial count in the samples isolated from gingival pockets of dogs and humans are presented in Fig. 1. The highest percentage pathogenic bacteria in dogs were P. gingivalis in the Dm group (67%) and P. gingivalis and T. denticola in the Df group (each 44%). In humans, the most commonly isolated bacteria were C. gingivalis (28%) in the Hm group and P. gingivalis (34%) in the Hf group. In dogs of both sexes, the levels of E. nodatum isolated were negligible. Furthermore, Tannerella forsythia was not isolated in male dogs, while in female dogs Tannerella forsythia accounted for only 1% of the total pathogenic bacteria. E. nodatum was only isolated in negligible quantities in male and female humans. A. actinomycetemcomitans was not isolated in dogs.
In male dogs, the second most common bacterium was T. denticola (17%), and the percentages of F. nucleatum, C. gingivalis, Prevotella intermedia, and Peptostreptococcus micros isolated in the Dm group were 7%, 6%, 2%, and 1 %, respectively. In female dogs, F. nucleatum, Peptostreptococcus micros, and Prevotella intermedia comprised 5%, 3%, and 2%, respectively, of the pathogenic bacterial pool, while C. gingivalis and Tannerella forsythia were 1% each of the total bacterial pool in the Df group. In male humans, the second most common bacterium was T. denticola (20%), followed by P. gingivalis (17%), Tannerella forsythia (16%), F. nucleatum (10%), and Peptostreptococcus micros (7%). Less commonly isolated bacteria in the Hm group included Prevotella intermedia and A. actinomycetemcomitans (1% each). In female humans, the isolated bacteria included F. nucleatum (14%), Tannerella forsythia (12%), T. denticola and C. gingivalis (11% each), Prevotella intermedia (10%), Peptostreptococcus micros (6%), and A. actinomycetemcomitans (2%).
The results for specific bacteria counts in samples isolated from the gingival pockets of the four study groups are presented in Table 1 and Fig. 2. Red complex bacteria comprised the largest portion of the identified bacterial complexes in all study groups, with P. gingivalis being the most commonly isolated bacterium. The next most common bacterial complex was the orange complex. In female humans, the predominant pathogenic bacterium in the studied samples was Prevotella intermedia, while in males (both human and canine) the predominant bacterium was F. nucleatum. C. gingivalis abundance was higher in male humans than in any other group, but the difference was not statistically significant. However, statistically significant differences between humans and dogs (regardless of sex) were observed in Prevotella intermedia, Peptostreptococcus micros, and C. gingivalis bacteria (p ≤ 0.05). These bacteria were isolated in larger amounts in humans than in dogs. The C. gingivalis count was significantly higher in the Dm group than in the Df group. Moreover, the number of C. gingivalis was significantly higher than in the Dm group than in the Hm group. The difference in Prevotella intermedia counts in the Df and Hf groups were also significantly different with the higher Prevotella intermedia count observed in the Hf group. Furthermore, there were statistically significant different T. denticola counts between the Hm and Hf groups (the number of T. denticola was higher in females than in males).
In both humans and dogs, periodontal disease poses a serious diagnostic and therapeutic problem. The bacteria causing periodontitis, apart from irreversibly damaging periodontal tissues, can also produce certain systemic diseases. Hence the importance of their identification in both human and veterinary medicines. Compared to other bacteria, the bacteria isolated from gingival pockets have a different sensitivity to antibiotics, and their treatment may lead to the proliferation of resistant pathogenic bacteria and/or reduce their susceptibility to chemotherapeutics. Moreover, introduction of an antibiotic treatment may destroy beneficial bacteria, upset the balance of the entire oral cavity ecosystem, and, as a consequence, cause a relapse of the original disease. Given the above, the predominance of specific bacteria isolated from gingival pockets must be considered when planning an antibiotic therapy. A real-time PCR-based testing method, although expensive, allows for relatively easy and quick microbiological analysis of gingival pocket samples, making it easier to select an appropriate pharmacological treatment and providing grounds for subsequent verification of the treatment's effectiveness. Provision of a correctly targeted antibiotic therapy is important in both species because of therapeutic difficulties that occur during the course of periodontal disease.
In dogs, plaque accumulation and periodontal disease progression is considerably faster than in humans, most likely due to insufficiencies in daily oral hygiene [18]. In addition, it has been confirmed that age plays a significant part in the progression of periodontitis in both species [1519]. Yamasaki et al. [40] demonstrated that the total bacteria count in dogs is positively correlated with animal age.
Mixed microbial infections are common in the course of periodontitis [327]. Our study has revealed considerable diversity in the anaerobic bacteria present in that disease. Previous study has indicated that most anaerobic bacteria isolated from gingival pockets in both dogs and humans belong to three genera: Fusobacterium, Porphyromonas, and Prevotella, which for many years, have been considered as key micro-organisms responsible for periodontal diseases in humans. In dogs, the count of Porphyromonas spp. isolated from plaque is reported to increase with age and to be correlated with the stage of the periodontal disease [18]. P. gingivalis is commonly considered as the bacterium responsible for the onset of periodontal disease and is generally strongly associated with this disease [21]. Other researchers have described T. denticola and Tannerella forsythia as significant pathogens in this disease [34]. Despite numerous studies into the bacterial microflora in humans and canines, there are contradictory results. On the one hand, it has been demonstrated that the character of the microflora is largely similar in the two species, while other studies have reported significant differences in terms of particular bacterial species and strains, despite phenotypical similarities [912]. The results of our study have revealed interspecies similarities in the prevalences of P. gingivalis, T. denticola, Tannerella forsythia, and F. nucleatum infections with no statistically significant differences in the prevalence of those bacteria between dogs and humans.
Kato et al. [20] studied the prevalences of Tannerella forsythia and A. actinomycetemcomitans in dogs with gingivitis. One of the most common bacteria they isolated was Tannerella forsythia, which contradicts the results of the present study wherein, in the case of male dogs, that bacterium was not isolated at all, while in female dogs it was found in a negligible amount in only one subject. In terms of A. actinomycetemcomitans, the results of Kato et al. [20] matched our own in that they did not observe this bacterium in any of the dogs. In a study by Yamasaki et al. [40] the most commonly isolated pathogenic bacterium was Tannerella forsythia (77.3%), while P. gingivalis, T. denticola, Prevotella intermedia, and A. actinomycetemcomitans were rarely isolated, both in dogs and in their owners.
Davis et al. [13], in their study of dogs with periodontal disease, demonstrated the predominance of Actinomyces, Peptostreptococcaceae, and Porphyromonas. Moreover, Fusobacterium spp. and Treponema spp., which are associated with periodontitis in humans, were reported to be at lower level in dogs. In addition, the authors revealed a relationship between the presence of T. denticola and the prevalence of mild periodontal inflammation. Allaker et al. [5] did not identify Treponema spp. in their study on dogs. The results they obtained are therefore in direct contradiction to our own as, in our study, T. denticola was very commonly isolated and constituted 44% of the whole pathogenic bacterial pool in female dogs and 17% of that pool in male dogs.
After reviewing relevant literature we conclude that the genus most frequently isolated from plaque in dogs is Porphyromonas. Sturgeon et al. [38], in their metagenetic analysis of bacteria colonizing the oral cavities of dogs, demonstrated that the dominant type of bacteria was Porphyromonas. In our study, that bacterium constituted 44% of the total count of pathogenic bacteria in female dogs and 67% in male dogs. Similar to T. denticola, Porphyromonas was observed in all tested animals (male and female). Allaker et al. [5] identified P. gingivalis in 68% of their test subjects and Nishiyama et al. [26] also reported its high prevalence (64%). Di Bello et al. [15] reported identifying red complex bacteria in dogs with periodontitis and observed a mix of P. gingivalis and Tannerella forsythia infections in 49.3% of their subjects. They found a significant association between gingivitis/periodontitis and the presence of P. gingivalis, in combination with Tannerella forsythia. Dahlén et al. [12] also reported Porphyromonas spp. to be the most dominant type of bacteria in their study dogs. Fusobacterium spp. and Peptostreptococcus spp. also had a considerable presence in their dogs; however, Prevotella spp. was not present in their dogs.
F. nucleatum and Prevotella intermedia were observed in 83% and 67%, respectively, of all animals in our study. In terms of the total pathogenic bacterial count, however, the percentage presence of those two bacteria was negligible. Other researchers have reported higher prevalence rates of these two bacteria. In a study by Allaker et al. [5] F. nucleatum was observed in 16% of dogs with periodontitis and Prevotella intermedia in as many as 20% of the dogs. In another study, Senhorinho et al. [34] reported the presence of F. nucleatum in the subgingival region in 16% of their study dogs with periodontitis, and suggested that Fusobacterium has an important role in the development of periodontal disease in dogs. That bacterium has demonstrated synergism with other bacteria and has the ability to aggregate with other micro-organisms inside a gingival pocket [7].
In our study, Peptostreptococcus micros was found in only one animal and E. nodatum was not present in half of the dogs. Given the relatively low presence of these pathogenic bacteria in our samples, and consequently, their low apparent value compared to the other pathogenic bacteria present, we conclude that these bacteria have a negligible part in the pathogenesis of canine periodontal disease. C. gingivalis was isolated in our study in all canine subjects tested and constituted 6% of the pathogenic bacteria pool isolated from male dogs.
In humans, among the pool of pathogenic bacteria isolated, higher C. gingivalis counts were observed in the Hm group while greater amounts of P. gingivalis were observed in the Hf group. In the bacterial red complex, we isolated P. gingivalis in 75% of the human subjects and isolated T. denticola and Tannerella forsythia in 92% of those subjects. Da Silva-Boghossian et al. [11] observed a high prevalence of red complex bacteria in subjects with periodontal diseases, and Abiko et al. [1] identified Tannerella forsythia and P. gingivalis as the predominant pathogens in the course of periodontitis in humans. In contrast, Al-Hebsi et al. [4], in their study of bacterial microflora in humans with chronic periodontitis, reported a low correlation between P. gingivalis and periodontal disease when compared to the correlations with other evaluated bacteria; those results contradict the results in this study.
Abusleme et al. [2] reported that the most numerous bacteria isolated from subgingival regions in their study were of the Fusobacterium and Treponema genera. While Griffen et al. [16] observed high counts of Prevotella bacteria in periodontitis, Abusleme et al. [2] recorded lesser numbers of that bacterium and attributed the discrepancy in results to the depth of the gingival pockets from which the samples were harvested. In our study, Prevotella intermedia was isolated in 34% of the subjects but accounted for a small percentage of total pathogenic bacteria (1% in males and 10% in females).
In our study, E. nodatum was found in subjects of both sexes (one male and four females) but in negligible amounts. In contrast to our results, Haffajee et al. [17] reported a considerably high correlation between E. nodatum and chronic periodontitis, both in subjects with high numbers of Tannerella forsythia and P. gingivalis, as well as in subjects whose levels of red complex bacteria were relatively low. Moore and Moore [23] documented elevated levels of E. nodatum in various forms of periodontitis, while in healthy people and those suffering from gingivitis the presence of E. nodatum was either negligible or absent. In other studies, E. nodatum was related to periodontitis and positive correlations were reported with the depth of gingival pockets and the extent of connective tissue detachment [1028].
Previous study has emphasized the role of A. actinomycetemcomitans in the etiology of localized aggressive periodontitis in humans [30]. Schacher et al. [33] observed higher numbers of this bacteria in people with aggressive periodontitis than that in people with general severe periodontitis. In our research, A. actinomycetemcomitans was isolated in 50% of the studied subjects, but the pathogen counts among the harvested samples were not statistically significant. This may be due to the fact that none of the subjects had a localized form of aggressive periodontitis.
Salari et al. [32] studied the presence of subgingival periopathogens in humans with chronic periodontitis. In terms of the bacteria identified in our study, they reported isolating P. gingivalis (21.9%), Prevotella intermedia (10.5%), Peptostreptococcus micros (2.9%), C. gingivalis (2.2%), and F. nucleatum (0.4%) among their total pathogen pool. In comparison, our results indicated far greater percentages of C. gingivalis, Peptostreptococcus micros, and F. nucleatum. Other authors have also reported considerably different percentages of these bacteria. For instance, Mane et al. [22] isolated the following: P. gingivalis (48%), F. nucleatum (24%), Peptostreptococcus micros (23%), and Prevotella spp. (26%). In their study, the prevalences of P. gingivalis, F. nucleatum, and Prevotella intermedia isolation were significantly different between the group of subjects with periodontitis and those with a healthy periodontium.
The prevalence of specific bacteria varies not only among the studied species but also between the sexes. The study results confirm that periodontal disease is the result of bacterial complexes rather than from an individual pathogen. Indeed, the presence of individual pathogens is insufficient to produce periodontitis. One should consider that the isolation of bacteria from a subgingival biofilm is not independent of the technique used to harvest the samples [139]. The real-time PCR method used in this study allows specific and sensitive identification and quantification of periodontal pathogens. Boutaga et al. [7] compared real-time PCR and cell culture results for five periopathogens to determine the methods' identification and quantitative analysis capacities and demonstrated a high level of concordance between the two methods. They described real-time PCR as a viable alternative for quantitative assessment of anaerobic bacteria isolated from the subgingival region. In another study [8], the authors deemed the real-time PCR method as effective in detecting very small amounts of periopathogens in collected samples (indeed, it could detect amounts that are below the detection limit of the cell culture technique). We must also consider the fact that discrepancies between results of studies may be due not only to the method of periopathogen detection but also to factors such as ambient conditions and the host's immunological status and genetic background.
A limitation of the present study is that there was no control group. For this reason, it was impossible to compare the count of individual bacteria isolated in periodontal disease with that in a healthy periodontium. Regardless, our study was differentiating and comparative, thus we checked differences between groups of dogs and humans in the course of periodontal disease.
In conclusion, on the basis of this study, there are similarities in the prevalence of bacterial microflora in dogs and humans. As transmission of oral bacteria during normal contacts between dogs and humans or through dog bites is feasible, one might expect correlations between the oral microflora of dogs and humans, suggesting the possibility of zoonotic effects, which has been reported by other researchers [1540]; however, such effects should be further investigated on a large scale. Moreover, rapid testing based on the real-time PCR method facilitates the choice of an appropriate pharmacological treatment, which is of particular importance considering the differences in the occurrence of individual bacteria in the subgingival area and their different sensitivity to antibiotics.
Figures and Tables
Fig. 1
Percentages of specific micro-organisms within the total pathogenic bacterial count in samples isolated from gingival pockets in dog and human study groups. Dm, male dogs; Df, female dogs; Hm, men; Hf, women; Aa, Aggregatibacter actinomycetemcomitans; Pg, Porphyromonas gingivalis; Td, Treponema denticola; Tf, Tannerella forsythia; Pi, Prevotella intermedia; Pm, Peptostreptococcus micros; Fn, Fusobacterium nucleatum; En, Eubacterium nodatum; Cg, Capnocytophaga gingivalis. The symbols indicate statistically significant differences: *between the count of Porphyromonas gingivalis isolated from male dogs and those in other groups; †between the count of Treponema denticola isolated from female dogs to those in other groups; ‡between the count of Tannerella forsythia isolated from female dogs to those in men and women; §between the count of Prevotella intermedia isolated from women to those in other groups; and ∥between the count of Capnocytophaga gingivalis isolated from men to those in other groups, respectively.
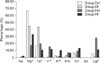
Fig. 2
Comparison of the prevalence of specific bacteria isolated from the four study groups. Dm, male dogs; Df, female dogs; Hm, men; Hf, women; Aa, Aggregatibacter actinomycetemcomitans; Pg, Porphyromonas gingivalis; Td, Treponema denticola; Tf, Tannerella forsythia; Pi, Prevotella intermedia; Pm, Peptostreptococcus micros; Fn, Fusobacterium nucleatum; En, Eubacterium nodatum; Cg, Capnocytophaga gingivalis. *Statistically significant differences between humans and dogs (regardless of sex). †The most commonly isolated bacterium.
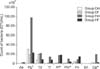
Table 1
Counts of bacteria isolated from gingival pockets among the four study groups
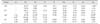
Data are presented as mean (x) ± SD. “x” means bacteria count (×104 CFU/mL). Dm, male dogs; Df, female dogs; Hm, men; Hf, women. The different letters indicate statistically significant differences within a column at p ≤ 0.05. Aa, Aggregatibacter actinomycetemcomitans; Pg, Porphyromonas gingivalis; Td, Treponema denticola; Tf, Tannerella forsythia; Pi, Prevotella intermedia; Pm, Peptostreptococcus micros; Fn, Fusobacterium nucleatum; En, Eubacterium nodatum; Cg, Capnocytophaga gingivalis.
Acknowledgments
The authors thank the human subjects and the owners of the dogs for their participation in this study.
References
1. Abiko Y, Sato T, Mayanagi G, Takahashi N. Profiling of subgingival plaque biofilm microflora from periodontally healthy subjects and from subjects with periodontitis using quantitative real-time PCR. J Periodontal Res. 2010; 45:389–395.


2. Abusleme L, Dupuy AK, Dutzan N, Silva N, Burleson JA, Strausbaugh LD, Gamonal J, Diaz PI. The subgingival microbiome in health and periodontitis and its relationship with community biomass and inflammation. ISME J. 2013; 7:1016–1025.


4. Al-hebshi NN, Al-Alimi A, Taiyeb-Ali T, Jaafar N. Quantitative analysis of classical and new putative periodontal pathogens in subgingival biofilm: a case-control study. J Periodontal Res. 2015; 50:320–329.


5. Allaker RP, Young KA, Langlois T, de Rosayro R, Hardie JM. Dental plaque flora of the dog with reference to fastidious and anaerobic bacteria associated with bites. J Vet Dent. 1997; 14:127–130.


6. Boutaga K, van Winkelhoff AJ, Vandenbroucke-Grauls CMJE, Savelkoul PHM. Comparison of real-time PCR and culture for detection of Porphyromonas gingivalis in subgingival plaque samples. J Clin Microbiol. 2003; 41:4950–4954.


7. Boutaga K, van Winkelhoff AJ, Vandenbroucke-Grauls CMJE, Savelkoul PHM. Periodontal pathogens: a quantitative comparison of anaerobic culture and real-time PCR. FEMS Immunol Med Microbiol. 2005; 45:191–199.


8. Boutaga K, van Winkelhoff AJ, Vandenbroucke-Grauls CMJE, Savelkoul PHM. The additional value of real-time PCR in the quantitative detection of periodontal pathogens. J Clin Periodontol. 2006; 33:427–433.


9. Charalampakis G, Abrahamsson I, Carcuac O, Dahlén G, Berglundh T. Microbiota in experimental periodontitis and peri-implantitis in dogs. Clin Oral Implants Res. 2014; 25:1094–1098.


10. Colombo AP, Teles RP, Torres MC, Souto R, Rosalém W Jr, Mendes MCS, Uzeda M. Subgingival microbiota of Brazilian subjects with untreated chronic periodontitis. J Periodontol. 2002; 73:360–369.


11. da Silva-Boghossian CM, do Souto RM, Luiz RR, Colombo APV. Association of red complex, A. actinomycetemcomitans and non-oral bacteria with periodontal diseases. Arch Oral Biol. 2011; 56:899–906.


12. Dahlén G, Charalampakis G, Abrahamsson I, Bengtsson L, Falsen E. Predominant bacterial species in subgingival plaque in dogs. J Periodontal Res. 2012; 47:354–364.


13. Davis IJ, Wallis C, Deusch O, Colyer A, Milella L, Loman N, Harris S. A cross-sectional survey of bacterial species in plaque from client owned dogs with healthy gingiva, gingivitis or mild periodontitis. PLoS One. 2013; 8:e83158.


14. Dewhirst FE, Klein EA, Thompson EC, Blanton JM, Chen T, Milella L, Buckley CM, Davis IJ, Bennett ML, Marshall-Jones ZV. The canine oral microbiome. PLoS One. 2012; 7:e36067.


15. Di Bello A, Buonavoglia A, Franchini D, Valastro C, Ventrella G, Greco MF, Corrente M. Periodontal disease associated with red complex bacteria in dogs. J Small Anim Pract. 2014; 55:160–163.


16. Griffen AL, Beall CJ, Campbell JH, Firestone ND, Kumar PS, Yang ZK, Podar M, Leys EJ. Distinct and complex bacterial profiles in human periodontitis and health revealed by 16S pyrosequencing. ISME J. 2012; 6:1176–1185.


17. Haffajee AD, Teles RP, Socransky SS. Association of Eubacterium nodatum and Treponema denticola with human periodontitis lesions. Oral Microbiol Immunol. 2006; 21:269–282.


18. Hardham J, Dreier K, Wong J, Sfintescu C, Evans RT. Pigmented-anaerobic bacteria associated with canine periodontitis. Vet Microbiol. 2005; 106:119–128.


19. Hirai N, Shirai M, Kato Y, Murakami M, Nomura R, Yamasaki Y, Takahashi S, Kondo C, Matsumoto-Nakano M, Nakano K, Asai F. Correlation of age with distribution of periodontitis-related bacteria in Japanese dogs. J Vet Med Sci. 2013; 75:999–1001.


20. Kato Y, Shirai M, Murakami M, Mizusawa T, Hagimoto A, Wada K, Nomura R, Nakano K, Ooshima T, Asai F. Molecular detection of human periodontal pathogens in oral swab specimens from dogs in Japan. J Vet Dent. 2011; 28:84–89.


21. Khazandi M, Bird PS, Owens J, Wilson G, Meyer JN, Trott DJ. In vitro efficacy of cefovecin against anaerobic bacteria isolated from subgingival plaque of dogs and cats with periodontal disease. Anaerobe. 2014; 28:104–108.


22. Mane AK, Karmarkar AP, Bharadwaj RS. Anaerobic bacteria in subjects with chronic periodontitis and in periodontal health. J Oral Health Community Dent. 2009; 3:49–51.


24. Mueller HP. Periodontology. Lublin: Thieme;2003. p. 15–29.
25. Nakayama K. Porphyromonas gingivalis and related bacteria: from colonial pigmentation to the type IX secretion system and gliding motility. J Periodont Res. 2015; 50:1–8.


26. Nishiyama SAB, Senhorinho GNA, Gioso MA, Avila-Campos MJ. Detection of putative periodontal pathogens in subgingival specimens of dogs. Braz J Microbiol. 2007; 38:23–28.


28. Papapanou PN, Neiderud AM, Papadimitriou A, Sandros J, Dahlén G. “Checkerboard” assessments of periodontal microbiota and serum antibody responses: a case-control study. J Periodontol. 2000; 71:885–897.


29. Paster BJ, Boches SK, Galvin JL, Ericson RE, Lau CN, Levanos VA, Sahasrabudhe A, Dewhirst FE. Bacterial diversity in human subgingival plaque. J Bacteriol. 2001; 183:3770–3783.


30. Raja M, Ummer F, Dhivakar CP. Aggregatibacter actinomycetemcomitans - a tooth killer? J Clin Diagn Res. 2014; 8:ZE13–ZE16.
31. Riggio MP, Lennon A, Taylor DJ, Bennett D. Molecular identification of bacteria associated with canine periodontal disease. Vet Microbiol. 2011; 150:394–400.


32. Salari MH, Kadkhoda Z. Rate of cultivable subgingival periodontopathogenic bacteria in chronic periodontitis. J Oral Sci. 2004; 46:157–161.


33. Schacher B, Baron F, Rossberg M, Wohlfeil M, Arndt R, Eickholz P. Aggregatibacter actinomycetemcomitans as indicator for aggressive periodontitis by two analysing strategies. J Clin Periodontol. 2007; 34:566–573.


34. Senhorinho GN, Nakano V, Liu C, Song Y, Finegold SM, Avila-Campos MJ. Occurrence and antimicrobial susceptibility of Porphyromonas spp. and Fusobacterium spp. in dogs with and without periodontitis. Anaerobe. 2012; 18:381–385.


35. Socransky SS, Haffajee AD, Cugini MA, Smith C, Kent RL Jr. Microbial complexes in subgingival plaque. J Clin Periodontol. 1998; 25:134–144.


36. Socransky SS, Haffajee AD. Dental biofilms: difficult therapeutic targets. Periodontol 2000. 2002; 28:12–55.


37. Struillou X, Boutigny H, Soueidan A, Layrolle P. Experimental animal models in periodontology: a review. Open Dent J. 2010; 4:37–47.


38. Sturgeon A, Stull JW, Costa MC, Weese JS. Metagenomic analysis of the canine oral cavity as revealed by high-throughput pyrosequencing of the 16S rRNA gene. Vet Microbiol. 2013; 162:891–898.

