Abstract
Objective
Multiple sclerosis (MS) is an inflammatory disease characterized by demyelinating plaques in the white matter. Chronic cerebrospinal venous insufficiency (CCSVI) has been proposed as a new hypothesis for the etiopathogenesis of MS disease. MS-CCSVI includes a significant decrease of cerebrospinal fluid (CSF) flow through the cerebral aqueduct secondary to an impaired venous outflow from the central nervous system. This study aimed to determine whether CSF flow dynamics are affected in MS patients and the contributions to differential diagnosis in active and chronic disease using phase-contrast magnetic resonance imaging (PC-MRI).
Materials and Methods
We studied 16 MS patients with chronic plaques (group 1), 16 MS patients with active plaques-enhanced on MRI (group 2), and 16 healthy controls (group 3). Quantitatively evaluation of the CSF flow was performed from the level of the cerebral aqueduct by PC-MRI. According to heart rates, 14–30 images were obtained in a cardiac cycle. Cardiac triggering was performed prospectively using finger plethysmography.
Results
No statistically significant difference was found between the groups regarding average velocity, net forward volume and the average flow (p > 0.05). Compared with the controls, group 1 and group 2, showed a higher peak velocity (5.5 ± 1.4, 4.9 ± 1.0, and 4.3 ± 1.3 cm/sec, respectively; p = 0.040), aqueductal area (5.0 ± 1.3, 4.1 ± 1.5, and 3.1 ± 1.2 mm2, respectively; p = 0.002), forward volume (0.039 ± 0.016, 0.031 ± 0.013, and 0.021 ± 0.010 mL, respectively; p = 0.002) and reverse volume (0.027 ± 0.016, 0.018 ± 0.009, and 0.012 ± 0.006 mL, respectively; p = 0.000). There were no statistical significance between the MS patients with chronic plaques and active plaques except for reverse volume. The MS patients with chronic plaques showed a significantly higher reverse volume (p = 0.000).
Multiple sclerosis (MS) is an inflammatory demyelinating disease of the central nervous system (CNS) with as yet unknown pathogenesis. A great number of demyelinating plaques of different sizes with sharp borders and round, oval or irregular shapes can be seen in MS (12). Magnetic resonance imaging (MRI) can clearly differentiate between active or chronic plaques based on the contrast involvement of the plaques. The topographic association between focal MS plaques in the white matter and cerebral veins (venocentric distribution of plaques) is of ongoing interest. This association has been shown with MR venography (3456) and postmortem (7) studies. To explain the association, studies on assessment of the extracranial venous drainage with imaging methods have been conducted. Chronic cerebrospinal venous insufficiency (CCSVI) has been proposed as a new hypothesis for MS etiology and pathogenesis and has attracted attention in the scientific community. MS-CCSVI hypothesis suggests that MS develops secondary to impaired venous outlet from CNS; and venous reflux causes iron loading that triggers autoimmune events with the impairment of blood-brain barrier (8). Impaired extracranial venous hemodynamics influences the cerebrospinal fluid (CSF) flow dynamics (91011). Phase contrast MRI (PC-MRI) is a non-invasive technique that can be used to quantitatively evaluate CSF flow dynamics (121314).
The purpose of this study was to determine whether CSF flow dynamics are affected in MS patients and can differentiate active versus chronic disease.
Phase contrast MRI was performed on 48 patients admitted to our institution between January 2012–January 2013. Data on these patients were collected prospectively. The cases were divided into three groups (12 females and 4 males). Group 1 consisted of 16 relapsing-remitting MS (RRMS) patients between the ages of 18–50 years with chronic plaques on MRI (mean: 32.6); group 2, 16 RRMS patients between the ages of 19–52 years with active plaques-enhanced on MRI (mean: 32.8); and group 3, 16 healthy volunteers between the ages of 21–49 years (mean: 32.6). The volunteers did not have any neurological disease. Inclusion criteria for 32 RRMS patients fulfilling the revised McDonald diagnostic criteria (15) were as follows: 1) RR disease process, 2) Expanded Disability Status Scale (16) between 0–5.5, 3) disease duration between 1–15 years (Table 1). Twenty patients (11 chronic MS, 9 active MS) were on disease-modifying treatment (interferon [n = 14], glatiramer-acetate [n = 4], natalizumab [n = 2]). Age and sex distribution showed no significant differences between the three groups (p < 0.05).
Prior to this study, ethics committee (Institutional Review Board) approval was obtained, and informed consent was obtained from all participants before MRI examination.
All MR examinations were conducted using the same protocol, with a 1.5T MRI scanner (Magnetom Avanto, Siemens Healthcare Gmbh, Erlangen, Germany). The PC-MRI sequences had following scan parameters: repetetion time, 31.25 ms; echo time, 8.06 ms; section thickness, 5.5 mm; number of signal averaging, 1; field of view, 16 × 10 cm; matrix size, 128 × 256; flip angle, 10°. According to heart rate, sections ranging between 14–30 were obtained in a cardiac cycle. Cardiac triggering was performed prospectively using finger plethysmography.
The obtained images were evaluated on a Siemens user console (Argus Flow, Siemens Healthcare Gmbh) by two radiologists blinded to the subjects' clinical status. The quantitative evaluation of CSF flow was performed with images that were obtained on the axial plane perpendicular to the ampulla of the cerebral aqueduct by phase contrast MR angiography technique (Fig. 1A). After adequate magnification of images, a region of interest (ROI) was placed manually to cover the whole area of the cerebral aqueduct in every image (ROI area < 1.5 mm2 was an exclusion criteria) (Fig. 1B). Subsequently, a data table was obtained with graphs of peak velocity-time, mean velocity-time, flow-time, and net flow-time for a cardiac cycle (Fig. 1C). The data table contains the following: 1) peak velocity (− or + direction, cm/sec), 2) mean velocity (cm/sec), 3) mean flow (mL/sec), 4) forward volume (craniocaudal CSF volume, mL/cycle), 5) reverse volume (caudocranial CSF volume, mL/cycle), 6) net forward volume (forward-reverse volume, mL/cycle), and 7) average aqueductal area (Fig. 1D). In all the graphs, the area above the horizontal axis (positive values, diastolic) indicates caudocranial CSF flow and the area under the axis (negative values, systolic), craniocaudal CSF flow.
Peak velocity, average velocity, forward volume, reverse volume, net forward volume, average flow values of current flowing through the cerebral aqueduct in a cardiac beat and average aqueductal areas were compared between the three groups. Parameters were expressed using mean and standard deviation. Shapiro-Wilk test was applied as a test of normality. Forward volume, net forward volume and the average flow, indicating a normal distribution, were assessed using the one-way analysis of variance and the groups were compared using the Least Significant Difference test (p = 0.05). The peak velocity, average velocity, reverse volume and aqueductal area parameters were not in a normal distribution and were assessed with the Kruskal-Wallis test (p = 0.05). Moreover, the groups were compared using the Conover test (p = 0.05).
In the group 1 (MS patients with chronic plaques), average peak velocity was 5.5 ± 1.4 cm/sec (range, 3.4–8.4 cm/sec); average velocity, 0.36 ± 0.20 cm/sec (range, 0.04–0.70 cm/sec); average forward volume, 0.039 ± 0.016 mL (range, 0.010–0.080 mL); average reverse volume, 0.027 ± 0.016 mL (range, 0.010–0.080 mL); net forward volume, 0.012 ± 0.007 mL (range, 0.00–0.030 mL); average flow, 0.018 ± 0.010 mL/sec (range, 0.00–0.040 mL/sec); and average aqueductal area, 5.0 ± 1.3 mm2 (range, 2.7–9.1 mm2).
In the group 2 (MS patients with active plaques), average peak velocity was 4.9 ± 1.0 cm/sec (range, 2.7–6.4 cm/sec); average velocity, 0.50 ± 0.30 cm/sec (range, 0.08–1.13 cm/sec); average forward volume, 0.031 ± 0.013 mL (range, 0.010–0.060 mL); average reverse volume, 0.018 ± 0.009 mL (range, 0.00–0.040 mL); net forward volume, 0.013 ± 0.008 mL (range, 0.00–0.040 mL); average flow, 0.019 ± 0.012 mL/sec (range, 0.00–0.040 mL/sec); and average aqueductal area, 4.1 ± 1.5 mm2 (range, 1.8–7.3 mm2).
In the group 3 (controls), average peak velocity was 4.3 ± 1.3 cm/sec (range, 2.9–7.7 cm/sec); average velocity, 0.41 ± 0.27 cm/sec (range, 0.05–1.07 cm/sec); average forward volume, 0.021 ± 0.010 mL (range, 0.010–0.050 mL); average reverse volume, 0.012 ± 0.006 mL (range, 0.00–0.030 mL); net forward volume, 0.008 ± 0.006 mL (range, 0.00–0.020 mL); average flow, 0.013 ± 0.010 mL/sec (range, 0.00–0.040 mL/sec); and average aqueductal area, 3.1 ± 1.2 mm2 (range, 1.6–5.8 mm2).
The average velocity, net forward volume, and average flow were not significantly different between the three groups regarding (p > 0.05).
The MS patients with chronic plaques and active plaques, compared with the controls, showed a higher peak velocity, forward volume and reverse volume (Table 2). There were statistical significance between the MS patients with chronic plaques and the control group (p < 0.05).
As shown in Table 2, the MS patients with active plaques and chronic plaques, compared with the controls, showed a higher aqueductal area, with statistical significance (p < 0.05).
There were no statistical significance between the MS patients with chronic plaques and active plaques, except for reverse volume. The MS patients with chronic plaques showed a significantly higher reverse volume (p = 0.000).
The peak velocity, forward volume, and aqueductal area were higher in MS patients with chronic plaques, without statistical significance (p > 0.05).
Inter-observer reliability of CSF flow measurements was excellent for peak velocity and average velocity (κ = 1, 0.971, respectively). Inter-observer reliability of average flow, forward volume, reverse volume, net forward volume, and average aqueductal area measurements was good (κ = 0.786, 0.755, 0.759, 0.785, and 0.714, respectively).
Multiple sclerosis is an inflammatory disease characterized by demyelination centered around the cerebral veins in the white matter (12). CCSVI has been suggested as a new hypothesis for the etiopathogenesis of MS disease in terms of this topographic relationship. CCSVI-related MS hypothesis suggests that significant decrease in CSF flow occurs through cerebral aqueducts secondary to impaired venous outflow from the CNS (81718). The normal CSF circulation depends on the proper balance between CSF ultrafiltration (from lateral ventricular veins) and venous system clearance from CSF opening at the level of dural sinuses (192021).
Zamboni et al. (17) have described abnormal venous hemodynamics by identifying multiple extracranial venous structures of unknown origin using extracranial-transcranial color Doppler and selective venography. CCSVI-related MS hypothesis claims that venous reflux leads to iron deposition in the CNS, triggering autoimmune process with disruption of the blood-brain barrier (8). PC-MRI facilitates accurate and noninvasive evaluation of the flow direction and flow rate of intracranial blood and CSF (1213142223).
Zamboni et al. (10) reported a strong relationship between CCSVI and MS. However, Zamboni et al. (911) found a significantly higher prevalence of CCSVI in MS patients, as compared to controls; and consequently, a decrease in net CSF flow (mL/beat) passing through the cerebral aqueduct. They concluded that CCSVI has a marked effect especially on intracranial fluid balance in the pathophysiology of brain.
In their study, Zamboni et al. (10) addressed the questions of whether CCSVI affects the clinical process of MS and whether venous stenosis is a cause or a result of MS. Baracchini et al. (24) and Simka et al. (25) reported a cause and effect relationship between MS and CCSVI; while Patti et al. (26) reported no relationship between MS and CCSVI. However, CSF flow dynamics were not mentioned in these studies.
Sundström et al. (27) performed a study to test the hypothesis of CCSVI and, unlike Zamboni et al. (11), did not detect any difference in internal jugular venous outflow and aqueductal CSF flow between RRMS patients and the control group. Gorucu et al. (28) reported that the net CSF flow volume showed no significant differences between MS patients and controls. In the present study, the net CSF flow volume (mL/beat) and average flow (mL/sec) parameters evaluated with PC-MRI, were not found to be statistically significant between MS patients and the control group. Additionally, MS patients were divided into 2 groups according to the condition of plaques that were detected on MRI: MS patients with active plaques who exhibit contrast enhancement and those with chronic disease non-enhancement. To our best knowledge, PC-MRI study for CSF dynamics in the active phase of the disease has not been reported. If CSF flow is decreased due to impaired extracranial venous drainage in MS patients, based on the study of Zamboni et al. (11), we expected lower CSF flow values in the intense period of active inflammation. Therefore, we determined whether PC-MRI contributes to differential diagnosis of active and chronic disease in MS. Craniocaudal (forward) and caudocranial (reverse) CSF flow volumes (mL/beat) and aqueductal area were higher in the MS patients with chronic plaques, as compared to patients with active plaques; and of these parameters, reverse volume was statistically significant. In addition, these parameters were higher in MS patients, as compared with the control group.
Our study compared flow speeds in addition to CSF flow volumes passing from the cerebral aqueduct in MS patients. No significant difference was found in terms of average velocity. However, peak velocity value was found to be significantly higher in MS patients with chronic plaque, as compared with the control group.
Increased craniocaudal and caudocranial CSF flow volume and aqueductal area in MS patients could possibly be due to cerebral atrophic changes that may occur at every stage of MS. CSF spaces expand with cerebral atrophy and consequently, CSF flow volumes in a cardiac cycle pass through the cerebral aqueduct. In MS patients, peak velocity increase can depend on the volume increases in the CSF circulation. Thus, in MS patients with these findings, absence of a significant change in the net CSF flow volume and average velocity, supports this concept. Likewise, Chiang et al. (29) concluded that the aqueductal CSF flow should not be considered independently of ventricular morphology. Zamboni et al. (9) suggested that impaired CSF dynamics in MS patients contributes to an increase in volumes of the third and lateral ventricles. In addition, reverse volume in the definitive diagnosis of active and chronic period MS disease, was higher in MS patients with chronic plaques. This result can be used in the place of contrast-enhanced MRI.
We did not evaluate the venous outflow abnormalities and the ventricle volumes, which is a limitation of our study. In addition, PC-MRI may result in errors in quantitative measurements, due to non-linear gradients, partial volume effects, and errors in placing ROI (123031). In cases with narrow aqueducts, difficulties in placing ROI can increase the error rate (32). For a reliable measurement, the area of aqueducts should be over 1.5 mm2 (14). In our study, the case with the smallest aqueducts area was in the control group with 1.6 mm2.
In conclusion, in the present study, the peak velocity, caudocranial and craniocaudal CSF flow volumes, and aqueductal area were significantly higher in the MS patients. Within the framework of the CCSVI hypothesis, the results of our study did not support the hypothesis that CSF flow decreases in MS patients. Nevertheless, CSF flow, was altered in MS patients. The increase in CSF flow in our study could be due to atrophy-dependent ventricular system dilation and subsequent decrease in the overall cerebral aqueduct resistance. In turn, the altered cerebral flow physiology may be used to differentiate active versus chronic disease. Larger controlled studies are needed to determine the entire scope of CSF dynamics and extracranial venous hemodynamics within the framework of the CCSVI hypothesis on MS etiology and pathogenesis.
References
1. Noseworthy JH, Lucchinetti C, Rodriguez M, Weinshenker BG. Multiple sclerosis. N Engl J Med. 2000; 343:938–952. PMID: 11006371.


2. Frohman EM, Racke MK, Raine CS. Multiple sclerosis--the plaque and its pathogenesis. N Engl J Med. 2006; 354:942–955. PMID: 16510748.
3. Ge Y, Zohrabian VM, Grossman RI. Seven-Tesla magnetic resonance imaging: new vision of microvascular abnormalities in multiple sclerosis. Arch Neurol. 2008; 65:812–816. PMID: 18541803.
4. Kermode AG, Thompson AJ, Tofts P, MacManus DG, Kendall BE, Kingsley DP, et al. Breakdown of the blood-brain barrier precedes symptoms and other MRI signs of new lesions in multiple sclerosis. Pathogenetic and clinical implications. Brain. 1990; 113(Pt 5):1477–1489. PMID: 2245307.
5. Kidd D, Barkhof F, McConnell R, Algra PR, Allen IV, Revesz T. Cortical lesions in multiple sclerosis. Brain. 1999; 122(Pt 1):17–26. PMID: 10050891.


6. Tan IL, van Schijndel RA, Pouwels PJ, van Walderveen MA, Reichenbach JR, Manoliu RA, et al. MR venography of multiple sclerosis. AJNR Am J Neuroradiol. 2000; 21:1039–1042. PMID: 10871010.
7. Fog T. The topography of plaques in multiple sclerosis with special reference to cerebral plaques. Acta Neurol Scand Suppl. 1965; 15:1–161. PMID: 5213727.
8. Singh AV, Zamboni P. Anomalous venous blood flow and iron deposition in multiple sclerosis. J Cereb Blood Flow Metab. 2009; 29:1867–1878. PMID: 19724286.


9. Zamboni P, Menegatti E, Weinstock-Guttman B, Schirda C, Cox JL, Malagoni AM, et al. CSF dynamics and brain volume in multiple sclerosis are associated with extracranial venous flow anomalies: a pilot study. Int Angiol. 2010; 29:140–148. PMID: 20351670.
10. Zamboni P, Galeotti R, Menegatti E, Malagoni AM, Tacconi G, Dall'Ara S, et al. Chronic cerebrospinal venous insufficiency in patients with multiple sclerosis. J Neurol Neurosurg Psychiatry. 2009; 80:392–399. PMID: 19060024.


11. Zamboni P, Menegatti E, Weinstock-Guttman B, Schirda C, Cox JL, Malagoni AM, et al. The severity of chronic cerebrospinal venous insufficiency in patients with multiple sclerosis is related to altered cerebrospinal fluid dynamics. Funct Neurol. 2009; 24:133–138. PMID: 20018140.
12. Barkhof F, Kouwenhoven M, Scheltens P, Sprenger M, Algra P, Valk J. Phase-contrast cine MR imaging of normal aqueductal CSF flow. Effect of aging and relation to CSF void on modulus MR. Acta Radiol. 1994; 35:123–130. PMID: 8172735.
13. Gideon P, Thomsen C, Ståhlberg F, Henriksen O. Cerebrospinal fluid production and dynamics in normal aging: a MRI phase-mapping study. Acta Neurol Scand. 1994; 89:362–366. PMID: 8085434.


14. Unal O, Kartum A, Avcu S, Etlik O, Arslan H, Bora A. Cine phase-contrast MRI evaluation of normal aqueductal cerebrospinal fluid flow according to sex and age. Diagn Interv Radiol. 2009; 15:227–231. PMID: 19862673.
15. Polman CH, Reingold SC, Edan G, Filippi M, Hartung HP, Kappos L, et al. Diagnostic criteria for multiple sclerosis: 2005 revisions to the “McDonald Criteria”. Ann Neurol. 2005; 58:840–846. PMID: 16283615.


16. Kurtzke JF. Rating neurologic impairment in multiple sclerosis: an expanded disability status scale (EDSS). Neurology. 1983; 33:1444–1452. PMID: 6685237.


17. Zamboni P, Menegatti E, Bartolomei I, Galeotti R, Malagoni AM, Tacconi G, et al. Intracranial venous haemodynamics in multiple sclerosis. Curr Neurovasc Res. 2007; 4:252–258. PMID: 18045150.


18. Ghezzi A, Comi G, Federico A. Chronic cerebro-spinal venous insufficiency (CCSVI) and multiple sclerosis. Neurol Sci. 2011; 32:17–21. PMID: 21161309.


19. Ursino M, Lodi CA. A simple mathematical model of the interaction between intracranial pressure and cerebral hemodynamics. J Appl Physiol (1985). 1997; 82:1256–1269. PMID: 9104864.
20. Kim J, Thacker NA, Bromiley PA, Jackson A. Prediction of the jugular venous waveform using a model of CSF dynamics. AJNR Am J Neuroradiol. 2007; 28:983–989. PMID: 17494684.
21. Schaller B. Physiology of cerebral venous blood flow: from experimental data in animals to normal function in humans. Brain Res Brain Res Rev. 2004; 46:243–260. PMID: 15571768.


22. Evans AJ, Iwai F, Grist TA, Sostman HD, Hedlund LW, Spritzer CE, et al. Magnetic resonance imaging of blood flow with a phase subtraction technique. In vitro and in vivo validation. Invest Radiol. 1993; 28:109–115. PMID: 8444566.
23. Pelc LR, Pelc NJ, Rayhill SC, Castro LJ, Glover GH, Herfkens RJ, et al. Arterial and venous blood flow: noninvasive quantitation with MR imaging. Radiology. 1992; 185:809–812. PMID: 1438767.


24. Baracchini C, Perini P, Calabrese M, Causin F, Rinaldi F, Gallo P. No evidence of chronic cerebrospinal venous insufficiency at multiple sclerosis onset. Ann Neurol. 2011; 69:90–99. PMID: 21280079.


25. Simka M, Ludyga T, Kazibudzki M, Latacz P, Swierad M. Multiple sclerosis, an unlikely cause of chronic cerebrospinal venous insufficiency: retrospective analysis of catheter venography. JRSM Short Rep. 2012; 3:56. PMID: 23301144.


26. Patti F, Nicoletti A, Leone C, Messina S, D'Amico E, Lo Fermo S, et al. Multiple sclerosis and CCSVI: a population-based case control study. PLoS One. 2012; 7:e41227. PMID: 22870210.


27. Sundström P, Wåhlin A, Ambarki K, Birgander R, Eklund A, Malm J. Venous and cerebrospinal fluid flow in multiple sclerosis: a case-control study. Ann Neurol. 2010; 68:255–259. PMID: 20695018.


28. Gorucu Y, Albayram S, Balci B, Hasiloglu ZI, Yenigul K, Yargic F, et al. Cerebrospinal fluid flow dynamics in patients with multiple sclerosis: a phase contrast magnetic resonance study. Funct Neurol. 2011; 26:215–222. PMID: 22364942.
29. Chiang WW, Takoudis CG, Lee SH, Weis-McNulty A, Glick R, Alperin N. Relationship between ventricular morphology and aqueductal cerebrospinal fluid flow in healthy and communicating hydrocephalus. Invest Radiol. 2009; 44:192–199. PMID: 19300098.


30. Nitz WR, Bradley WG Jr, Watanabe AS, Lee RR, Burgoyne B, O'Sullivan RM, et al. Flow dynamics of cerebrospinal fluid: assessment with phase-contrast velocity MR imaging performed with retrospective cardiac gating. Radiology. 1992; 183:395–405. PMID: 1561340.


31. Henry-Feugeas MC, Idy-Peretti I, Blanchet B, Hassine D, Zannoli G, Schouman-Claeys E. Temporal and spatial assessment of normal cerebrospinal fluid dynamics with MR imaging. Magn Reson Imaging. 1993; 11:1107–1118. PMID: 8271897.


32. Lee JH, Lee HK, Kim JK, Kim HJ, Park JK, Choi CG. CSF flow quantification of the cerebral aqueduct in normal volunteers using phase contrast cine MR imaging. Korean J Radiol. 2004; 5:81–86. PMID: 15235231.


Fig. 1
Measurement of flow of cerebrospinal fluid with phase-contrast MRI.
A. Saggital T2 scan showing positioning for cine MRI acquisition. B. Cerebral aqueduct region-of-interest drawing on phase image, after sufficient magnification. C. Peak velocity-time graph obtained for cardiac beat. D. Data table obtained using Argus software.
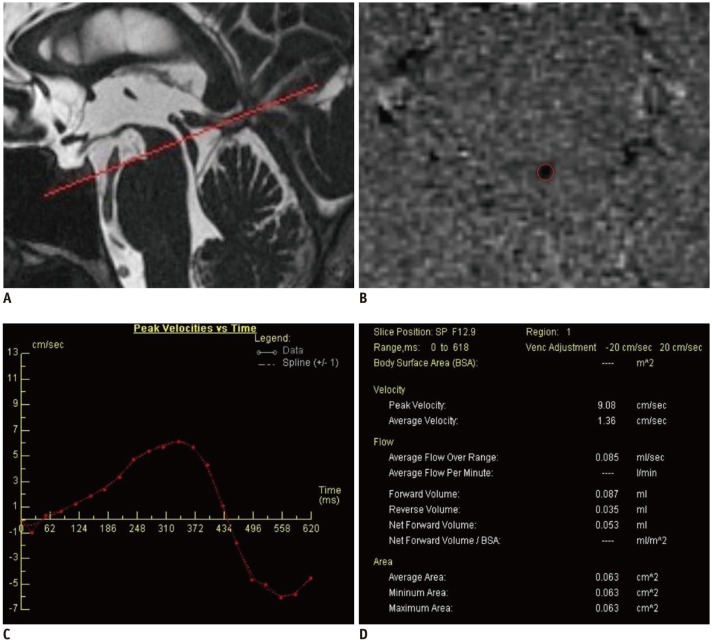
Table 1
Demographic and Clinical Characteristics of MS Patients and Control Subjects
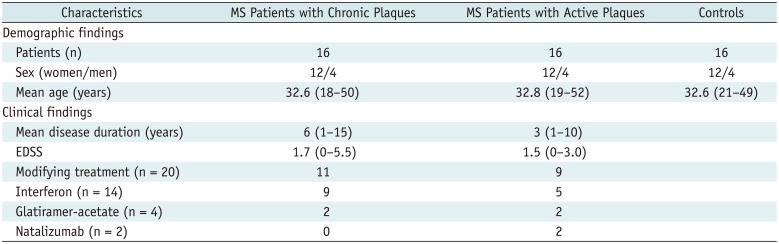
Table 2
Cerebrospinal Fluid Dynamics in Relapsing-Remitting MS Patients and Controls
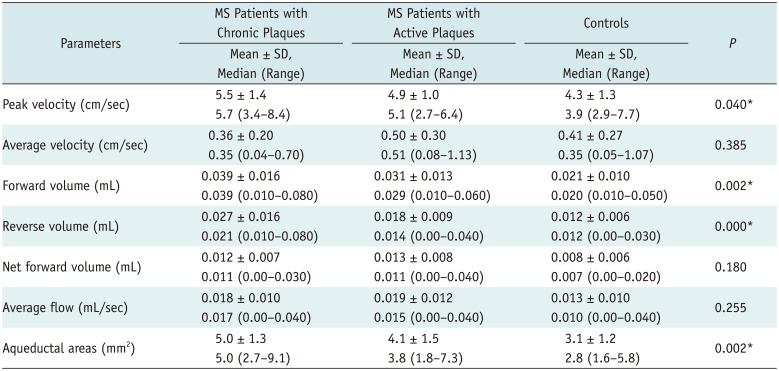