Abstract
Purpose
The aim of the present study was to investigate the protective effects of ischemic preconditioning for different periods of time and to elucidate the optimal safe ischemic preconditioning time for renal ischemia-reperfusion (I/R) injury in mice.
Methods
A total of 25 male C57BL/6 mice were randomly divided into 5 groups (sham, I/R, ischemic preconditioning [IP]-3, IP-5, and IP-7 groups), in which the kidney was preconditioned with IP of various durations and then subjected to I/R injury (the last 3 groups). To induce renal ischemia, the left renal pedicle was occluded with a nontraumatic microaneurysm clamp for 30 minutes followed by reperfusion for 24 hours. The effects of IP on renal I/R injury were evaluated in terms of renal function, tubular necrosis, apoptotic cell death and inflammatory cytokines.
Results
Results indicated that BUN and creatinine (Cr) levels increased significantly in the I/R group, but the elevations were significantly lower in IP groups, especially in the IP-5 group. Histological analysis revealed that kidney injury was markedly decreased in the IP-5 group compared with the I/R group, as evidenced by reduced renal necrosis/apoptosis. In addition, IP significantly inhibited gene expression of pro-inflammatory cytokines (TNF-α, IL-1β, and IL-6) and chemokines (monocyte chemoattractant protein-1). Western blot analysis indicated that the expression levels of Toll-like receptor 4 (TLR4) and nuclear factor-kappa B (NF-κB) were upregulated in the I/R group, while expression was inhibited in the IP groups.
Renal ischemia-reperfusion (I/R) injury, the leading cause of acute kidney injury, delays graft function and is associated with early mortality in patients undergoing kidney transplantation [1]. Rather than restoring normal function, reperfusion causes damage through the generation of reactive oxygen species and inflammation [2]. Therefore, the need for additional therapeutic modalities to prevent renal I/R injury is quite urgent.
Ischemic preconditioning (IP) is a phenomenon that promotes tissue tolerance to I/R injury via a brief period of ischemia and subsequent reperfusion before ischemic injury is established. Numerous signal transduction pathways have been shown to be mediators affording protection by IP.
Recent studies indicated that Toll-like receptors (TLRs) are involved in the IP process [34]. TLRs are a family of transmembrane proteins that can activate various signaling pathways in response to inflammatory reactions. TLR4, a member of the TLRs, is a pattern recognition receptor that plays a key role in innate immunity against invading pathogens, as well as in sterile inflammatory responses [5]. TLR4 has increasingly been shown to play a role in the pathogenesis of renal I/R injury [67], and the transcription factor nuclear factor-kappa B (NF-κB) has been implicated as a key mediator of reperfusion injury [8]. Although numerous studies have investigated IP and its effects, there has been no established or verified protocol to maximize the organ protective effects of IP. This could be one of the reasons that IP is not currently recommended. For these reasons, we conducted the present study to investigate the protective effects of ischemic preconditioning for different time periods and to elucidate the optimal safe ischemic preconditioning time for renal I/R injury in mice.
Male C57BL/6 mice weighing 23–25 g were used (Damul Science, Daejeon, Korea). They were kept at room temperature (23℃–25℃), 40%–60% relative humidity on a 12/12-hour light/dark cycle and were fed a standard pellet diet with water ad libitum. Mice (including those in the sham group) were anesthetized by intraperitoneal administration of a mixture of xylazine (10 mg/kg) and ketamine (100 mg/kg) and were placed on a heating pad under a warming light to maintain their body temperature between 36℃ and 38℃. Bilateral flank incisions were made and a right nephrectomy was performed. The left renal pedicle was occluded with a nontraumatic microaneurysm clamp to induce renal ischemia. Reperfusion was initiated by removing the clamp. Mice were randomly divided into five experimental groups (n = 5 for each group, Table 1). In the I/R group, the renal pedicle was clamped for 30 minutes and then reperfused for 24 hours. In the IP groups, just before the sustained ischemia, kidneys were subjected to 3, 5, or 7 minutes of ischemia followed by 10 minutes of reperfusion. Soon after, the renal pedicle was clamped for 30 minutes then reperfused. The data from each of these groups were compared to those of a sham group in which animals underwent only right nephrectomy. Twenty-four hours after reperfusion the mice were sacrificed and serum and tissue samples were collected and immediately fixed in 10% formalin or stored at −80℃ until further analysis. All animal procedures and experiments were performed according to a protocol approved by the Institutional Animal Care and Use Committee of the Clinical Research Institute at Daejeon St. Mary's Hospital at the Catholic University of Korea (institutional review board #CMCDJ-AP-2012-018).
Blood samples were collected 24 hours after reperfusion. BUN and serum Cr were measured using IDEXX VetTest Chemistry Analyzer (IDEXX Laboratories Inc., Westbrook, ME, USA).
Kidney tissues were fixed in 10% neutral buffered formalin and then embedded in paraffin. Tissue was sectioned at 0.5-µm and then stained with hematoxylin and eosin (H&E) and periodic acid-Schiff reagent (PAS) for light microscopic analyses. Histopathologic damage was defined as tubular epithelial swelling, loss of brush border, vacuolar degeneration, necrotic tubules, cast formation, and desquamation. The degree of tubular injury was estimated at ×400 magnification using 5 randomly selected fields for each kidney using the following standard: 0, normal; 1, damage involving <25% of tubules; 2, damage involving 25%–50% of tubules; 3, damage involving 50%–75% of tubules; and 4, damage involving 75%–100% of tubules.
Apoptotic nuclei were identified with deoxynucleotidyl transferase biotin-dUTP nick end labeling (TUNEL) staining using the ApopTag Plus Peroxidase in situ Apoptosis Kit (Millipore, Billerica, MA, USA). TUNEL-positive areas from 2 to 3 fields (×400)/animal were quantified with Image J. Results are expressed as total area percent and were quantified from 5 animals per group.
Kidney tissues were homogenized using TissueLyser II (Qiagen, Hilden, Germany) in radioimmunoprecipitation assay (RIPA) buffer (Elpis Biotech., Daejeon, Korea) containing protease inhibitor cocktail tablets (Roche Diagnostics GmbH, Mannheim, Germany). Homogenates were centrifuged at 13,000 rpm for 15 minutes at 4℃, and the supernatants were collected. Nuclear proteins were extracted using a Nuclear/Cytosol Fractionation kit (BioVision, Mountain View, CA, USA) according to the manufacturer's instructions. After measuring the protein concentration (BCA Protein Assay Kit; Pierce, Rockford, IL, USA), equal amounts of protein were separated by electrophoresis on a 10% sodium dodecyl sulfate-polyacrylamide gel and transferred electrophoretically onto a nitrocellulose membrane (Pall Corporation, Ann Arbor, MI, USA). After blocking with 5% nonfat powdered milk, the membrane was incubated overnight at 4℃ with primary antibodies directed against Cleaved caspase-3 (Cell Signaling Technology, Beverly, MA, USA), Bax (Cell Signaling Technology), Bcl-2 (Cell Signaling Technology), and TLR4 (Abcam, Cambridge, MA, USA) or NF-κB (Abcam). They were then incubated with horseradish peroxidase-conjugated anti-rabbit IgG (Santa Cruz Biotechnology, Santa Cruz, CA, USA) or anti-mouse IgG (Invitrogen, Carlsbad, CA, USA). Positive bands were detected and analyzed by chemiluminescence technology using the ChemiDoc XRS+ (Bio-Rad Laboratories, Hercules, CA, USA). As loading controls, each membrane was probed with GAPDH (Cell Signaling Technology) or Histone H3.1 (Santa Cruz Biotechnology).
Total RNA was extracted from kidney tissues with TRIzol reagent (Invitrogen) according to the manufacturer's instructions. cDNA was synthesized from 1 µg RNA using Reverse Transcriptase Premix (Elpis Biotech). The expression levels were quantified by Power SYBR Green PCR Master Mix (Applied Biosystems, Warrington, UK) according to the manufacturer's instructions. The following primer sets (sense sequence and antisense sequence, respectively) were used: Tumor necrosis factor-α (TNF-α) forward, 5'-ACG GCA TGG ATC TCA AAG AC-3', reverse, 5'-GTG GGT GAG GAG CAC GTA GT-3'; Interleukin-1β (IL-1) forward, 5'-GCC CAT CCT CTG TGA CTC AT-3', reverse, 5'-AGG CCA CAG GTA TTT TGT CG-3'; Interleukin-6 (IL-6) forward, 5'-GAT GCT ACC AAA CTG GAT ATA ATC-3', reverse, 5'-GGT CCT TAG CCA CTC CTT CTG TG-3'; monocyte chemoattractant protein-1 (MCP-1) forward, 5'-CCA GCA AGA TGA TCC CAA TGA G-3', reverse, 5'-CTC TCT CTT GAG CTT GGT GAC AAA A-3'; glyceraldehyde 3-phosphate dehydrogenase (GAPDH) forward, 5'-TGC AGT GGC AAA GTG GAG ATT-3', reverse, 5'-CGT GAG TGG AGT CAT ACT GGA ACA-3'. Each reaction was performed by ABI 7500 FAST (Applied Biosystems, Foster City, CA, USA) in triplicate. The relative levels of mRNA were normalized to GAPDH.
Graph Pad Prism 5 (Graph Pad Inc., La Jolla, CA, USA) was used to analyze and present data. Differences between groups were analyzed using a paired parametric t-test or a one-way analysis of variance. Values are expressed as the mean ± standard error of the mean. A P-value of <0.05 was considered statistically significant.
The levels of serum BUN and Cr were significantly increased in the I/R group and were decreased in the IP-5 group (P < 0.01) (Fig. 1A). Histopathologic analysis revealed swelling in the renal tubular epithelial cells, vacuole degeneration, disappearance of the brush border, coagulation necrosis, and massive inflammatory cell infiltration in the I/R group compared with the sham group. However, IP reduced this severe renal damage (Fig. 1C). Quantitative analysis revealed a markedly decreased tubular injury score in the IP groups compared with the I/R group (P < 0.05) (Fig. 1B).
Although the major cause of cell death during renal I/R injury is necrosis, apoptotic cell death is also observed during the reperfusion process. The extent of apoptosis was evaluated by TUNEL staining (Fig. 2A). The number of TUNEL-positive apoptotic cells was markedly decreased in IP-injured mice compared to that in the I/R group (P < 0.05) (Fig. 2B). Increased protein levels of anti-apoptotic Bcl-2 and decreased protein levels of pro-apoptotic cleaved caspase-3 and Bax were observed in the IP groups (Fig. 2C, D).
The inflammatory response following I/R injury is related to renal dysfunction. To investigate whether IP mitigated renal dysfunction, we investigated the mRNA expression of TNF-α, IL-1β, IL-6, and MCP-1, which are key inflammatory cytokines produced by infiltrated inflammatory cells. Real-time RT-PCR analysis confirmed decreased accumulation of macrophages and suppressed inflammation in IP groups compared to the I/R group (P < 0.05) (Fig. 3).
Kidney TLR4 protein expression was significantly elevated after I/R, but not in the IP groups (Fig. 4A, B). IP groups had significantly decreased NF-κB expression induced by IR (Fig. 4C, D).
In this study, we demonstrated the protective effects of IP and the optimal safe ischemic preconditioning time for renal I/R injury in mice. IP is a potent protective strategy that causes a brief episode of I/R end-organ injury and enables tissues to be resistant to subsequent long-term I/R injury. Even though the protective effect of IP on renal I/R injury has been demonstrated in many animal studies, there is a large number of IP protocol types and the protective mechanism of renal IP is still not clear [91011]. There is no consensus on how many ischemic stimuli should be applied, and what the duration of the ischemic and intermediate reperfusion period should be [12]. In this study, we used one of the commonly used AKI mouse models of unilateral I/R injury with contralateral nephrectomy before 30 minutes of renal ischemia, and a 10 minutes window between IP and subsequent ischemia [13]. Here, we examined whether a one-cycle schedule of IP attenuated renal damage and then determined the optimal IP time.
Studies on IP have been conducted for the heart [14], liver [1516], small intestine [17], brain tissues [18], and kidney. Although the mechanism of IP is unclear, heat shock protein, endothelin, nitric oxide, and adenosine are factors believed to play important roles. Torras et al. [19] reported that IP induces local formation of nitric oxide and has a protective effect on renal function in a transplanted rat model. Pathologic characteristics of renal I/R injury include tubular epithelial cell necrosis and monocyte infiltration. This is due to sequential inflammatory responses controlled by NF-κB including cell adhesion, infiltration and cytokine production [20].
Our study demonstrates that IP treatment attenuated acute ischemic kidney injury as determined by improved renal function and histopathology and decreased inflammatory cytokines. As a potential renoprotective mechanism of IP, we evaluated the TLR4/NF-κB pathway and found that 3 through 5 minutes of IP treatment down-regulated TLR4 and NF-κB expression. In addition, 5 minutes of IP treatment significantly inhibited mRNA expression of TNF-α, IL-1β, and IL-6. NF-κB plays a critical role in the regulation of I/R-mediated gene expression and consecutive protein synthesis during the IP process [8].
TLR4 has increasingly been shown to play a role in the pathogenesis of renal IR injury [67]. It is not known how TLR4 signaling is initiated during I/R injury, although TLR4 recognizes endogenous ligands released from cells disrupted during I/R injury. TLR4 engagement by its ligands triggers downstream activation and expression of inflammatory cytokines and chemokines [21]. A high level of TLR4 expression was observed in renal I/R injury, and induction of its upregulation may be caused by infiltrating macrophages as well as intrinsic renal cells [22]. In the present study, we also examined the activation of TLR4/NF-κB according to various IP durations after I/R injury. All 3 IP groups showed significantly improved renal function and histopathology 24 hours after I/R injury. We observed that 5 minutes was the optimal IP duration in this mouse model. In spite of the small difference in IP time among groups (3, 5, and 7 minutes), we observed a significant difference in renoprotective effects. There are 2 possible reasons for this: the short time window (between IP and subsequent I/R) and the small size mouse model (23–25 g).
There are some limitations to this study. First, we did not investigate possible ligands that initiate TLR4 signaling during I/R injury and how these were related to optimum IP. Second, our assessments were made over a very short time period. It is possible that the relationship between IP and TLR4 may have different effects in the long-term. Thus, ligands and the long-term consequences of IP and TLR4 may need to be investigated in future studies.
In summary, the optimal duration of IP attenuates ischemic acute kidney injury by inhibition of renal inflammation, and downregulation of TLR4/NF-κB pathway is one of the protective mechanisms of IP treatment in renal I/R injury.
Figures and Tables
Fig. 1
Effects of ischemic preconditioning (IP) on ischemia-reperfusion (I/R)-induced acute kidney injury in mice. (A) Effects of IP on serum creatinine (Cr) and BUN levels after renal I/R injury. (B) Tubular damage was semiquantified by scoring H&E stained slides. (C) Representative images after hematoxylin and eosin (H&E) and Periodic acid-Schiff (PAS) staining of tissue taken 24 hours after I/R. Original magnification ×400. Data are presented as the mean ± standard error of the mean. *P < 0.05, **P < 0.01, ***P < 0.001 compared with the I/R group.
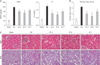
Fig. 2
Effects of ischemic preconditioning (IP) on ischemia-reperfusion (I/R)-induced apoptosis. (A) Kidney apoptosis was examined by transferase biotin-dUTP nick end labeling (TUNEL) staining (×400). (B) The level of apoptosis was expressed as the percent TUNEL-positive area. (C) The expression levels of cleaved caspase-3, Bax, and Bcl-2 were examined by Western blotting (one of three independent experiments). (D) Densitometric analysis of Western blot results. The density of each lane of glyceraldehyde-3-phosphate dehydrogenase (GAPDH) was divided by that of cleaved caspase-3, Bax, and Bcl-2. Data are presented as the mean ± standard error of the mean. *P < 0.05, **P < 0.01, ***P < 0.001 compared with the I/R group.
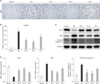
Fig. 3
IP attenuates inflammation induced by ischemia-reperfusion (I/R). Effects of ischemic preconditioning (IP) on mRNA expression of proinflammatory cytokines (A; TNF-α, B; IL-1β, and C; IL-6) and a chemokine (D; monocyte chemoattractant protein-1) were analyzed in ischemic kidneys by real-time RT-PCR analysis. Expression was normalized to that of glyceraldehyde-3-phosphate dehydrogenase (GAPDH). Data are presented as the mean ± standard error of the mean. *P < 0.05, **P < 0.01, ***P < 0.001 compared with the I/R group.
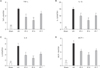
Fig. 4
Effects of ischemic preconditioning (IP) on the protein levels of Toll-like receptor 4 (TLR4) and nuclear factor-kappa B (NF-κB). (A) Representative Western blotting for TLR4. (B) Quantification analysis of TLR4 protein expression compared with glyceraldehyde-3-phosphate dehydrogenase (GAPDH) as the reference. (C) Western blotting for NF-κB p65 and (D) quantification analysis of NF-κB p65 protein expression with Histone H3.1 as the reference. Data are presented as the mean ± standard error of the mean. *P < 0.05, ***P < 0.001 compared with the I/R group.
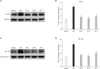
ACKNOWLEDGEMENTS
This research was supported by a Clinical Research Institute grant (CMCDJ-P-2013–021) funded by The Catholic University of Korea Daejeon St. Mary's Hospital, and the financial support of the Catholic Medical Center Research Foundation made in the program year of 2012.
References
1. Yoshida T, Kumagai H, Kohsaka T, Ikegaya N. Relaxin protects against renal ischemia-reperfusion injury. Am J Physiol Renal Physiol. 2013; 305:F1169–F1176.
2. Noiri E, Nakao A, Uchida K, Tsukahara H, Ohno M, Fujita T, et al. Oxidative and nitrosative stress in acute renal ischemia. Am J Physiol Renal Physiol. 2001; 281:F948–F957.
3. Chao W. Toll-like receptor signaling: a critical modulator of cell survival and ischemic injury in the heart. Am J Physiol Heart Circ Physiol. 2009; 296:H1–H12.
4. Valeur HS, Valen G. Innate immunity and myocardial adaptation to ischemia. Basic Res Cardiol. 2009; 104:22–32.
5. Zhao H, Perez JS, Lu K, George AJ, Ma D. Role of Toll-like receptor-4 in renal graft ischemia-reperfusion injury. Am J Physiol Renal Physiol. 2014; 306:F801–F811.
6. Gluba A, Banach M, Hannam S, Mikhailidis DP, Sakowicz A, Rysz J. The role of Toll-like receptors in renal diseases. Nat Rev Nephrol. 2010; 6:224–235.
7. Wu H, Chen G, Wyburn KR, Yin J, Bertolino P, Eris JM, et al. TLR4 activation mediates kidney ischemia/reperfusion injury. J Clin Invest. 2007; 117:2847–2859.
8. Jiang SH, Liu CF, Zhang XL, Xu XH, Zou JZ, Fang Y, et al. Renal protection by delayed ischaemic preconditioning is associated with inhibition of the inflammatory response and NF-kappaB activation. Cell Biochem Funct. 2007; 25:335–343.
9. Jia RP, Xie JJ, Luo FY, Zhu JG. Ischemic preconditioning improves rat kidney allograft function after ischemia/reperfusion injury: the role of tumor necrosis factor-alpha. Transplant Proc. 2008; 40:3316–3320.
10. Joo JD, Kim M, D'Agati VD, Lee HT. Ischemic preconditioning provides both acute and delayed protection against renal ischemia and reperfusion injury in mice. J Am Soc Nephrol. 2006; 17:3115–3123.
11. Chen X, Liu X, Wan X, Wu Y, Chen Y, Cao C. Ischemic preconditioning attenuates renal ischemia-reperfusion injury by inhibiting activation of IKKbeta and inflammatory response. Am J Nephrol. 2009; 30:287–294.
12. Wever KE, Menting TP, Rovers M, van der, Rongen GA, Masereeuw R, et al. Ischemic preconditioning in the animal kidney, a systematic review and meta-analysis. PLoS One. 2012; 7:e32296.
13. Wei Q, Dong Z. Mouse model of ischemic acute kidney injury: technical notes and tricks. Am J Physiol Renal Physiol. 2012; 303:F1487–F1494.
14. Mitchell MB, Meng X, Ao L, Brown JM, Harken AH, Banerjee A. Preconditioning of isolated rat heart is mediated by protein kinase C. Circ Res. 1995; 76:73–81.
15. Peralta C, Closa D, Xaus C, Gelpi E, Rosello-Catafau J, Hotter G. Hepatic preconditioning in rats is defined by a balance of adenosine and xanthine. Hepatology. 1998; 28:768–773.
16. Peralta C, Hotter G, Closa D, Gelpi E, Bulbena O, Rosello-Catafau J. Protective effect of preconditioning on the injury associated to hepatic ischemia-reperfusion in the rat: role of nitric oxide and adenosine. Hepatology. 1997; 25:934–937.
17. Hotter G, Closa D, Prados M, Fernandez-Cruz L, Prats N, Gelpi E, et al. Intestinal preconditioning is mediated by a transient increase in nitric oxide. Biochem Biophys Res Commun. 1996; 222:27–32.
18. Heurteaux C, Lauritzen I, Widmann C, Lazdunski M. Essential role of adenosine, adenosine A1 receptors, and ATP-sensitive K+ channels in cerebral ischemic preconditioning. Proc Natl Acad Sci U S A. 1995; 92:4666–4670.
19. Torras J, Herrero-Fresneda I, Lloberas N, Riera M, Ma Cruzado J, Ma Grinyó J. Promising effects of ischemic preconditioning in renal transplantation. Kidney Int. 2002; 61:2218–2227.
20. Ferencz A, Racz B, Gasz B, Benko L, Jancso G, Kurthy M, et al. Intestinal ischemic preconditioning in rats and NF-kappaB activation. Microsurgery. 2006; 26:54–57.
21. Liu M, Gu M, Xu D, Lv Q, Zhang W, Wu Y. Protective effects of Toll-like receptor 4 inhibitor eritoran on renal ischemia-reperfusion injury. Transplant Proc. 2010; 42:1539–1544.
22. Bergler T, Hoffmann U, Bergler E, Jung B, Banas MC, Reinhold SW, et al. Toll-like receptor 4 in experimental kidney transplantation: early mediator of endogenous danger signals. Nephron Exp Nephrol. 2012; 121:e59–e70.