Abstract
Purpose
Acute renal graft rejection can only be definitively diagnosed by renal biopsy. However, biopsies carry a risk of renal transplant injury and loss. Micro-CT is widely used in preclinical studies of small animals. Here, we propose micro-CT could noninvasively monitor and evaluate renal location and function in a mouse kidney transplant model.
Methods
Orthotopic kidney transplantation was performed in a BALB/c -to- C57BL/6j or C57BL/6j-to- C57BL/6j mouse model. After optimizing imaging techniques, five mice were imaged with micro-CT and the findings were verified histologically.
Transplant research has depended on animal models like the rat and the mouse for substantiation of positive results. Mouse kidney transplant models are an important means of exploring transplantation immunity and treatment development [1,2]. The relationship between the fields of immunology and transplantation is an archetype for translational research. Transplantation immunology research continues to endeavor to discover novel agents and alternatives to whole-organ transplantation. However, the assessment of kidney function by autopsy is cumbersome and time consuming and therefore this has led to the use of noninvasive imaging. Anatomical imaging for small animals is represented mostly with micro-CT because micro-CT has excellent spatial resolution (less than 50 µm) [3]. Thus, our goal was to evaluate the best way for detecting and monitoring renal location and function in a mouse kidney transplant model in the hope that these results might be generalized to other mouse models.
Male C57BL/6j and BALB/c mice that weighed 20 to 25 g were purchased from Shizuoka Laboratory Animal Center (Shizuoka, Japan). The mice were housed with food and tap water in accordance with the guidelines of the Animal Use and Care Committee of the National Research Institute for Child Health and Development, Tokyo, Japan. All animal experiments were approved by this committee and performed according to its recommendations.
The following mice strain combinations were studied across full main histocompatibility complex (MHC) and non-MHC disparities: BALB/c (H-2D, I-Ad) into C57BL/6j (H-2KbDb, I-Ab) and C57BL/6j into C57BL/6j. Vascularized kidney transplantation was performed as described previously [4,5] with minor modifications.
Micro-CT (LCT-200 scanner, Hitachi Aloka Medical Ltd., Tokyo, Japan) was performed at 55 kVp, with an anode current of 500 µA and a shutter speed of 500 ms. Scans were completed over 360 degrees of rotation of the x-ray tube with 450 projections to reduce signal-to-noise. Reconstructions were performed using a cone-beam filtered back projection algorithm. The axial field of view was set to 4.6 cm with an inplane spatial resolution of 91 µm. The mouse was anesthetized with isoflurane/oxygen general anesthesia systems. The tail veins of the mice were cannulated, and approximately 0.3 mL of the contrast agent Fenestra Liver Contrast (Advanced Research Technologies Inc., Saint-Laurent, QC, CA) was injected (0.013 mL of Fenestra LC per gram of body weight of animal). The total scan time for each micro-CT scan was approximately 5-10 minutes. Image acquisition started 5 and 30 minutes after contrast agent injection. Micro-CT image data were acquired reconstructed and analyzed using VGS tudio MAX 2.0 software (Volume Graphics GmbH, Heidelberg, Germany).
Animals were euthanized with carbon dioxide after the last imaging study. An autopsy was performed and tissue was removed then stored in 10% formalin. Histological sections were stained with hematoxylin and eosin. Comparison of the histopathology and imaging results were used to validate results from anatomical imaging.
We first imaged the mouse native kidney to establish baseline renal structural and functional parameters and to serve as the comparison group for transplanted kidneys. The statistical atlas-based registration was validated based on both non-contrast micro-CT images and contrast-enhanced micro-CT images (Fig. 1A/A' and B/B').
The transplanted mouse kidneys were imaged regularly every two days for two weeks after surgery. The syngeneic transplanted mouse kidneys were imaged for two months. Early (5 minutes) and delayed (30 minutes) micro-CT scans were obtained after the administration of Fenestra LC. Only by comparing these two images was it possible to confirm the presence of living renal transplant. As shown in the following figures, transplanted kidneys were detected in left abdomen at two days after surgery (Fig. 1C/C' and D/D'). In syngeneic transplanted models, the living renal transplant can be detected at 7 days after surgery (Fig. 1E/E') and more than two months (Fig. 1G/G'). However, allograft transplanted kidneys were found nonfunctioning at postoperative days 7 and 14 (Fig. 1F/F' and H/H'). There were no mice deaths while renal transplants were failure or nonfailure.
Native kidneys had no significant pathologic changes. Histological analysis of the syngeneic transplanted kidneys were performed by light microscopy at day 7 (Fig. 2A), day 60 (Fig. 2B) and beyond showed minimal pathology consisting of mild glomerular collapse with slight neutrophil infiltration, mild-tomoderate tubular dilatation, occasional tubular epithelial cell necrosis, and mild medullary interstitial congestion. In contrast, histological analysis of the allograft transplanted kidneys by light microscopy at day 7 (Fig. 2C) showed venulitis, mild tubulitis, and interstitial infiltration. Severe tubulitis lesions of a rejecting kidney allograft were observed at day 14 (Fig. 2D).
Small animal in vivo imaging plays an important role in preclinical studies [6]. Micro-CT is an important technology that provides in vivo anatomy of the animal body [7]. In this study, we investigated the feasibility and utility of using noninvasive imaging, micro-CT, to monitor renal allograft in mouse kidney transplantation models. To our knowledge, this is the first study of noninvasive monitor of renal allograft in mice. The findings herein suggest that micro-CT is a promising novel modality to noninvasively and laterally examine mouse (and likely other small rodent) models of disease including kidney and liver disease [8] or hepatic graft versus host disease [9]. As such, it holds potential as a new tool to better study disease pathogenesis and therapeutic response in existing models of kidney transplantation and facilitate development of novel and more clinically relevant models.
In humans, imaging CT is generally preferred because scanning times are very short and the contrast media exhibits rapid renal excretion from the body. In recent years, developments in biomedical research have focused on the development of micro-CT with the application of studying bone and lung disease, for which the natural and significantly visible contrast between bone, air and the surrounding soft tissues is met [10]. The main purpose for development of small-animal micro-CT is for use in imaging and monitoring the progression of various diseases over time (longitudinal imaging), tumor development and growth, visualization of blood vessels and angiogenesis, and following the tumors and various disease responses to preclinical therapeutic intervention [11,12]. This is all possible due to its excellent spatial resolution and the possibility of acquiring detailed anatomical structures. However, the lack of intra-abdominal structural contrast in CT often jeopardizes the detection of abdominal tumors.
Transplantation immunology research continues to endeavor to discover novel agents and alternatives to whole-organ transplantation. However, due to the complexity and redundancy of the immune system, in vitro findings frequently do not correlate with in vivo observations. Hence, transplant research has depended on animal models like the rat and the mouse for substantiation of positive results. Creating a successful animal model of renal transplant is important to the goal of developing successful therapies [2]. Graft outcomes after kidney transplantation continue to be adversely affected by ischemia-reperfusion injury and rejection. However, the assessment of kidney function by autopsy is cumbersome and time consuming and this has led to the use of noninvasive imaging. We demonstrated in this manuscript that micro-CT enabled high-resolution, real-time imaging of renal grafts in a live mouse and made possible the quantitation of functional changes in the renal allograft in the first few hours after transplantation. And, we are also able to follow the renal transplant with micro-CT for more than two months with the location and function detected.
It should be noted, though, that other methods of studying murine noninvasive imaging including MRI or PET/CE have been reported [13,14]. CT components are important for anatomical support and can be used also for improving the reconstruction process. While both MRI and CT are used in clinical practice, it is unclear whether micro-CT or MRI is superior for lesion localization. But scanning times of CT are very short and the contrast media exhibits rapid renal excretion from the body. The cost of CT is also lower than MRI. Most of the PET tracers are highly sensitive for their molecular targets, but provide relatively little anatomical information.
In conclusion, we demonstrated in this manuscript the feasibility of noninvasive imaging of the transplanted mouse kidney using micro-CT. The technique enabled high-resolution, real-time imaging of renal grafts in a live mouse after transplantation. We were able to simultaneously assess transplanted renal location and function.
Figures and Tables
Fig. 1
Imaging of the mouse native and transplanted kidney. The statistical atlas-based registration was validated based on both noncontrast (A/A') and contrast-enhanced (B/B') micro-CT images. Micro-CT images of the transplanted mouse kidneys day 2 (C/C' and D/D'), 7 (E/E' and F/F'), 14 (G/G'), and 60 (H/H') post operation were obtained. Early (5 minutes; without apostrophe: C to H) and delayed (30 minutes: with apostrophe: C' to H') micro-CT scans were taken to confirm the presence of living renal transplant. Living transplanted kidneys were detected by micro-CT in left abdomen at two days after surgery (C/C' and D/D'). In syngeneic transplanted models, the living renal transplant can be detected at more than two months (G/G'). However, allograft transplanted kidneys were found nonfunctioning at day 7 (F/F') and 14 (H/H') post operation.
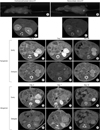
Fig. 2
Histological assessment. H&E staining was performed on the syngeneic transplanted kidneys at day 7 (A) and 60 (B) and allograft transplanted kidneys at day 7 (C) and 14 (D). Comparison with syngeneic transplanted kidney, the allograft transplanted kidneys showed venulitis, mild tubulitis, interstitial infiltration, and severe tubulitis. Bar indicates 200 µm and Bar in inset indicates 100 µm.
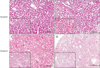
References
1. Ge F, Gong W. Strategies for successfully establishing a kidney transplant in a mouse model. Exp Clin Transplant. 2011; 9:287–294.
2. Tse GH, Hughes J, Marson LP. Systematic review of mouse kidney transplantation. Transpl Int. 2013; 26:1149–1160.
3. Schambach SJ, Bag S, Schilling L, Groden C, Brockmann MA. Application of micro-CT in small animal imaging. Methods. 2010; 50:2–13.
4. Azuma H, Isaka Y, Nomi H, Inamoto T, Li XK, Hounig T, et al. Induction of donorspecific tolerance using superagonistic CD28 antibody in rat renal allografts: regulatory T-cell expansion before engraftment may be important. Transplantation. 2010; 90:1328–1335.
5. Abe T, Li XK, Yazawa K, Hatayama N, Xie L, Sato B, et al. Hydrogen-rich University of Wisconsin solution attenuates renal cold ischemia-reperfusion injury. Transplantation. 2012; 94:14–21.
6. Willmann JK, van Bruggen N, Dinkelborg LM, Gambhir SS. Molecular imaging in drug development. Nat Rev Drug Discov. 2008; 7:591–607.
7. Soltysiak P, Saxena AK. Micro-computed tomography for implantation site imaging during in situ oesophagus tissue engineering in a live small animal model. J Tissue Eng Regen Med. 2009; 3:573–576.
8. Masyuk TV, Radtke BN, Stroope AJ, Banales JM, Masyuk AI, Gradilone SA, et al. Inhibition of Cdc25A suppresses hepato-renal cystogenesis in rodent models of polycystic kidney and liver disease. Gastroenterology. 2012; 142:622–633.e4.
9. Vierling JM, Hreha G, Wang H, Braun M. The role of biliary epithelial cells in the immunopathogenesis of non-suppurative destructive cholangitis in murine hepatic graft-versus-host disease. Trans Am Clin Climatol Assoc. 2011; 122:326–335.
10. Paulus MJ, Gleason SS, Kennel SJ, Hunsicker PR, Johnson DK. High resolution X-ray computed tomography: an emerging tool for small animal cancer research. Neoplasia. 2000; 2:62–70.
11. Marx J. Imaging. Animal models: live and in color. Science. 2003; 302:1880–1882.
12. Maehara N. Experimental microcomputed tomography study of the 3D microangioarchitecture of tumors. Eur Radiol. 2003; 13:1559–1565.
13. Cai QY, Lee H, Kim EJ, Moon H, Chang K, Rho J, et al. Magnetic resonance imaging of superparamagnetic iron oxide-labeled macrophage infiltrates in acute-phase renal ischemia-reperfusion mouse model. Nanomedicine. 2012; 8:365–373.
14. Reuter S, Schnockel U, Schroter R, Schober O, Pavenstadt H, Schafers M, et al. Noninvasive imaging of acute renal allograft rejection in rats using small animal FFDG-PET. PLoS One. 2009; 4:e5296.