Abstract
Purpose
Intimal hyperplasia (IH) is the main cause of restenosis or occlusion after vascular procedures. Imatinib mesylate and rapamycin are known to prevent IH. The purpose of this study was to evaluate the effect of these drugs on the regression of preformed IH in rat carotid injury model.
Methods
IH was established in rat carotid arteries using a balloon catheter. The drug effects were assessed in vitro on proliferation, migration, and apoptosis of vascular smooth muscle cells (VSMC) in the neointima. And in vivo studies were carried out in 4 groups: imatinib, rapamycin, combined, and no medication. After 2-week oral medication, morphometric analysis evaluated the number and density of neointimal cells, intima-to-media (I/M) ratio and cross-sectional area. Cell proliferation, apoptosis, and collagen changes were also investigated by immunohistochemical staining (IHCS).
Results
Imatinib and rapamycin significantly inhibited VSMC proliferation and migration, and promoted apoptosis in vitro. In morphometric analysis, the number and density of neointimal cells decreased significantly in all medication groups compared with control group (P < 0.01). However, there was no significant difference in neointimal cross-sectional area and I/M ratio among groups. In IHCS, imatinib and rapamycin inhibited neointimal cell proliferation significantly. However, there was no significant change in cell apoptosis and collagen composition.
Conclusion
Combined treatment of with imatinib and rapamycin induced reduction of cell mass in preformed intimal hyperplasia, but failed to induce regression of intimal mass in this short-term medication study. Further studies will be needed with additional strategies of inducing lysis of the extracellular matrix.
The long-term success of vein graft bypass or endovascular therapy (angioplasty or stenting) is limited by the development of stenosis or restenosis in the target lesion and intimal hyperplasia (IH) is considered as the major etiology of this phenomenon [1]. The mechanism of IH has been widely investigated in carotid denudation model. It is a process where activated vascular smooth muscle cells (VSMC) proliferate to form a neointima on top of the injured vascular endothelium [2].
There have been a lot of studies to prevent restenosis after vascular intervention and the introduction of stents coated with mTOR inhibitor rapamycin (Rapamune, Pfizer Inc., New York, NY, USA) has dramatically reduced restenosis rates after coronary revascularization [3]. Prevention of restenosis with oral rapamycin has been successful after angioplasty in animal models [4].
Imatinib mesylate (Glivec, Novartis International AG, Basel, Switzerland) is an inhibitor of the platelet-derived growth factor (PDGF) receptor kinase, bcr-abl kinase, and c-Kit receptor kinase [5], and is clinically used for the treatment of leukemia and gastrointestinal stromal tumor. In animal models, it has shown inhibitory effects on IH [6], transplant arteriopathy [7] and diabetes-associated atherosclerosis [8]. Recently, Vamvakopoulos et al. [9] reported that rapamycin and imatinib mesylate successfully inhibited IH and showed synergistic effect in rat carotid injury model.
The treatment strategies for IH can be prevention of the development of IH or induction of atrophy in the established IH. However, besides the prevention of IH, there is no study about medical treatment for the established restenosis. This study was designed to investigate a potential novel therapy inducing the regression of IH with rapamycin or imatinib mesylate in rat carotid injury model.
Male Sprague-Dawley rats weighing about 300 g were purchased from OrientBio Inc. (Seongnam, Korea), and underwent endothelial denudation of carotid artery using a balloon catheter as described elsewhere [10]. Briefly, animals were anesthetized with Zoletil (Virbac, Carros, France) 10 mg/kg and xylazine (Rompun, Bayer, Leverkusen, Germany) 5 mg/kg intraperitoneally. Left carotid artery was dissected via midline cervical incision. A Fogarty balloon catheter 2 Fr (Edwards Lifesciences Corp., Irvine, CA, USA) was introduced, advanced to the innominate artery, inflated with 0.2-mL saline, and passed 5 times in the common carotid artery. After wound closure, the animals were allowed to recover from anesthesia.
All rats were housed in pairs for the duration of study and fed standard chow and water. Access to food was not restricted except during 4 hours prior to dosing. Humane care was applied in compliance with the Guide for the Care and Use of Laboratory Animals (NIH publication No. 85-23, revised 1996). The protocol for this study was approved by the Seoul National University Hospital Institutional Animal Care and Use Committee (SNUH-IACUC, No. 07-0256).
VSMCs from the neointima of the left carotid artery at 4 weeks after injury were extracted and cultured with the enzymatic isolation method as previously described [11]. Extracted VSMCs were cultured using the Dulbecco's modified eagle's medium (DMEM, GIBCO, Grand Island, NY, USA) media containing 10% fetal bovine serum (FBS). VSMCs on passage 3-6 were used for the experiments.
VSMC viability and proliferation were measured by soluble tetrazolium salt method (WST-1, Roche applied Science, Indianapolis, IN, USA) and bromodeoxyuridine (BrdU) assay. For WST-1 method, VSMCs of 5 × 103 per well were cultured in 96-well plate with DMEM for 48 hours. After changing to serum-free media, various concentrations of drugs were added in the well containing PDGF-BB 10 ng/mL. Study groups were divided into imatinib mesylate, rapamycin and combined groups. Control was treated only with PDGF-BB. After 24 hours, ELISA absorption under 450 nm was measured in the reaction with WST solution 15 µL for 4 hours. For BrdU assay, VSMCs of 5 × 103 per well were cultured in 96-well plate with DMEM for 48 hours. After cell starvation, same concentrations of drugs were treated in study groups and control group as WST-1 method. After 24 hours, ELISA absorption under 370 nm was measured in the reaction with BrdU solution 15 µL for 2 hours.
To evaluate the effect of the drugs on VSMC migration, scratch-wound migration method was used as described previously [12]. In brief, VSMCs were cultured as 70%-90% confluence monolayer in 6-well plate containing DMEM with 10% FBS. After cell starvation for 48 hours, VSMCs were scratched with cell scraper to make the wound area and washed with PBS. Prescribed dose of drugs (10-9-10-7M) were applied in the well and incubated for 2 hours. The migration distance was quantified using LEICA microsystem DFC 290 (Heerbrugg, Switzerland) and LEICA application suite (ver. 3.4.1) software in 24 hours.
Cell cycle status was evaluated by fluorescence-activated cell sorter (FACS), as described previously [13]. After the incubation with drugs for 24 hours, VSMCs of 5 × 105 were collected and evaluated for the degree of cell apoptosis using Annexin V-FITC Apoptosis Detection kit (BD Biosciences, Franklin Lakes, NJ, USA). Flow cytometric analysis was performed on FACStar Plus (BD Biosciences), and the histograms were analyzed using Modifit LT software (Verity Software House, Topsham, ME, USA).
Total thirty-two Sprague-Dawley rats were used and divided to 4 groups (each, n = 8): rapamycin, imatinib mesylate, combined drugs and control with saline. After carotid injury operation, rats were fed for 14 days to allow the IH to develop. Then, designated drug or saline was administered once a day with oral gavage needle for 14 days. Dosages of each drug were rapamycin 1.0 mg/kg/day and imatinib mesylate 10 mg/kg/day [9].
Two weeks after drug administration, rats were anesthetized and carotid arteries were fixed by perfusion with 4% paraformaldehyde. The arteries were additionally fixed by immersion in the same fixative and the tissues were embedded in paraffin and sections were stained with H&E. The extent of neointima formation was quantified by computed planimetry of histologically stained sections.
The cross-sectional areas of arterial wall, including the lumen area, intimal area, and medial area, were quantified by using LEICA microsystem DFC 290 (Switzerland) and LEICA application suite (ver. 3.4.1) software. The intima-to-media (I/M) ratios were calculated from the mean of these determinations. The numbers of VSMC were also evaluated under 4 high power fields and used as the mean values.
IHCS was performed as previously described [10]. To detect proliferating cells, IHCS against proliferating cell nuclear antigen (PCNA) was performed. Proliferation index was assessed by quantifying the percentage of PCNA positive cells against total nucleated cells in 4 different sectors per tissue section. Detection of apoptotic cells was also performed using the terminal deoxynucleotidyl transferase-mediated dUTP nick end labeling (TUNEL) method with minor modifications. Apoptosis was quantified by counting the percentage of TUNEL-positive cells against total nucleated cells in 4 different sectors per tissue section. IHCS for the detection of cell apoptosis was performed with SignalStain Cleaved Caspase-3 (Asp175) IHC Detection kit (Cell Signaling Technology Inc., Danvers, MA, USA) [14].
To investigate the change of extrecellular matrix (ECM), Picrosirius red staining using Direct Red 80 (Sigma-Aldrich, St. Louis, MO, USA) was performed as described previously [15,16]. When examined through crossed polars, the larger collagen fibers are bright yellow or orange, and the thinner fibers, including reticular fibers, are green.
The degree of neointimal formation after carotid injury was check serially at day 3, 7, 14, and 28. The neointima was built up enough at 2 weeks after injury, comparable to that at 4 weeks and it was considered that neointimal formation process was entering stable state. Hence, we decided 2 weeks after injury as the starting point of drug administration to animals (Fig. 1).
WST-1 assay showed that cell viability did not decrease along with various drug concentrations except 10-5M combined group (data not shown). BrdU assay showed that cell proliferation significantly decreased along with the drug concentrations, especially in more than 10-7M of all medication groups (Fig. 2). In addition, cell proliferation was significantly inhibited in all medication groups in accordance with drug concentration compared to control. Interestingly, the degree of proliferation inhibition showed a synergistic effect in combined treatment group.
VSMC migration activity was significantly inhibited in all medication groups, especially in more than 10-8M. In addition, there was synergistic effect of imatinib mesylate and rapamycin in combined treatment group (Fig. 3).
FACS assay showed an increase of the portion of apoptotic cells in rapamycin group compared to control, which is not statistical significant (10.3% ± 1.9% vs. 7.3% ± 3.4%, P > 0.05). However, there was significant increase of apoptotic cell portion in imatinib mesylate and combined groups compared to control (19.6 ± 3.4, 21.2 ± 1.8, P < 0.001) (Fig. 4).
In comparison to control (72.15 ± 10.65), neointimal cell counts under the four random high-power fields (×400) significantly decreased in all medication groups (57.90 ± 11.67 in rapamycin, P = 0.011; 57.40 ± 10.98 in imatinib mesylate, P = 0.002; 53.05 ± 15.25 in combined, P = 0.007) (Fig. 5A). However, cross-sectional area was not different significantly among groups (2.06 ± 0.58 × 105 µm2 in rapamycin, 2.21 ± 0.61 × 105 µm2 in imatinib mesylate, 1.96 ± 0.77 × 105 µm2 in combined, 1.91 ± 0.71 × 105 µm2 in control, Fig. 5B). Comparing I/M ratio, there was no significant difference among groups (2.45 ± 0.46 in rapamycin, 2.65 ± 0.94 in imatinib mesylate, 2.30 ± 0.81 in combined, 2.50 ± 0.68 in control, Fig. 5C).
IHCS using PCNA showed PCNA-positive cells were significantly decreased in all medication groups compared to control (Fig. 6), which represented that cell proliferation was inhibited by each drug medication (14.35% ± 7.45% in rapamycin, 11.88% ± 6.50% in imatinib mesylate, 10.80% ± 5.29% in combined, 31.25% ± 11.76% in control, P < 0.001).
TUNEL and IHCS against cleaved caspase-3 showed that there was no significant difference in apoptosis at the time point of 4 weeks among medication groups compared to control. The change of collagen in the extracellular matrix was investigated with picro-sirius red staining. But there was no difference among the medication groups (data not shown).
IH is considered as the main cause of treatment failure of peripheral arterial disease due to restenosis and has significant impact on the patency of balloon angioplasty, stenting and bypass graft [9]. It has been known that IH is a complex process and is induced by VSMC proliferation and migration. There have been lots of studies on the prevention of IH with inhibition of VSMC proliferation and migration and drug-eluting stent is clinically used in coronary arterial disease [17,18,19]. Previously we investigated the possibility of new strategy of treating IH by inducing reduction of the preformed IH [20]. However, although the regression of preformed IH was inducible in animal studies using external wrapping or flow reduction model, which is unrealistic to be applied in clinics, there is no effective treatment for the patients with established IH at present. This study was designed to investigate a potential for the regression of IH by oral medication with rapamycin and imatinib mesylate, and to observe any synergistic effect of two drugs.
In vitro study using the cultured VSMC extracted from preformed IH, imatinib mesylate and rapamycin significantly inhibited the proliferation and migration of VSMCs and also showed synergistic effects of two drugs. In cell cycle analysis, the proportion of apoptotic cells significantly increased in imatinib mesylate and combined therapy groups, but it is not significant in rapamycin. These results are in concordance with previous studies [21,22]. The difference is that VSMCs used in this study were extracted and cultured from preformed IH at 4 weeks after denudation injury.
Based on these results, we also performed in vivo study to investigate the potential for inducing the regression of IH with medication starting from 2 weeks after carotid injury operation. As a result, VSMC proliferation was significantly inhibited in all medication groups compared to control group. However cross-sectional area and I/M ratio were not decreased. Furthermore, collagen change of ECM was not significantly different among groups.
There can be possible explanations of these results, including ineffective dosage of the drugs, too short duration of the medication to result in ECM mass reduction, or lack of effect with this regimen on reducing ECM mass. Dosage of drugs (imatinib mesylate 10 mg/kg, rapamycin 1.0 mg/kg) was based on the previous study which is known to be submaximal not inducing other drug-related systemic complication [9]. There is a limiting factor of systemic use of rapamycin in higher doses, aside from its potential drug-specific side effects. The drug's suppression of the immune system implicitly limits the patient's ability to respond appropriately to infectious, fungal, and carcinogenic threats [23].
Second issue is the timing and the duration of medication. We decided 2 weeks after injury as the starting point of medication because IH was fully established at 2 weeks in the pilot study (Fig. 1), and it seems a proper time period of stable state of VSMC proliferation [24]. Medication for 2-week duration was determined by previous study which showed successful effect to result in the prevention of VSMC proliferation after carotid injury. We used oral medication method using curved animal gavage, which is technically demanding and possibly cause injury to esophagus if used long-term. The duration of medication for 2 weeks would not be enough to induce the change of ECM, considering the fact that the change of ECM component develops later than that of VSMC itself. Longer duration of the medication possibly show reduction in the intimal mass. Because the intimal mass is the result of dynamic remodeling and balance between ECM deposition and lysis, reduction of cell mass which produce the ECM might cause later ECM mass reduction. But this hypothesis should be proven with another study. In a case report of cardiac allograft vasculopathy, rapamycin treatment for 1 year induced angiographic regression of the intimal mass and arterial stenosis in a patient [25].
Another explanation is that reducing the cell mass has little effect on reducing the neointimal mass unless accompanied by ECM lysis. ECM such as collagen is an important component of IH. ECM deposition is the fibrotic process including both an increased biosynthesis and reduced degradation of ECM components, particularly that of fibrillar collagens. Alternatively, reduced matrix metalloproteinase expression and subsequent inhibition of collagen degradation may also contribute to the fibrotic process [26].
Some study showed that imatinib mesylate and rapamycin inhibited ECM synthesis, which is different from this study about the regression of ECM [27]. Some anti-inflammatory effects of rapamycin have been demonstrated suggesting that they may be considered as antifibrogenic [28]. However, the molecular mechanisms underlying the effects of rapamycin on ECM gene expression remain poorly understood. It has been suggested that rapamycin inhibit types 1 and 3 collagen gene expression and synthesis by the activation c-Jun N-terminal kinase cascade and induction of transforming growth factor-β [29]. In addition, it has been demonstrated that imatinib mesylate significantly inhibit the pulmonary and hepatic fibrosis induced by the expression of PDGF in several studies [30]. But there is no report about the effect of these drugs on the lysis of ECM, which seems to be unlikely regarding the result of this study.
This study showed some discrepancy in the results between in vitro and in vivo. Imatinib mesylate and rapamycin inhibited VSMC proliferation and migration, but, in vivo, it failed to induce regression of ECM mass. Because ECM consists of the 80% of the intimal mass, cell mass reduction might be not enough to cause IH reduction. Additional method to induce ECM lysis might be needed in future researches.
Albeit with some limitations, this study showed some potential of oral medication for the induction of VSMC apoptosis in vivo, based on the finding of increase in cell apoptosis in vitro. Better determination of the dosage and duration of the medication might increase the efficacy of the medication. But, besides some positive results in vitro, the induction of regression for IH with imatinib mesylate and rapamycin was not apparent in this study. Further study will be needed about the methods of reducing extracellular matrix mass in this model.
Figures and Tables
Fig. 1
Cross-sectional images of the injured carotid arteries serially at 3 (A), 7 (B), 14 (C), and 28 days (D) after injury (H&E, light microscopy, ×100). Intimal hyperplasia was developed progressively after the injury. The neointima was built up enough at 14 days after injury, comparable to that at 28 days.
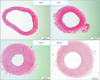
Fig. 2
Cell proliferation study with bromodeoxyuridine assay. Vascular smooth muscle cells from the neointimal of the injured left common carotid artery were used. Cell proliferation significantly decreased along with the drug concentrations, especially in more than 10-7M of all medication groups (*P < 0.05 vs. control; **P < 0.01 vs. control).
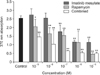
Fig. 3
Cell migration study with wound scratch assay. Vascular smooth muscle cells migration activity was significantly inhibited in all medication groups, especially in more than 10-8M (*P < 0.05 vs. control; **P < 0.01 vs. control).
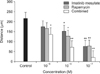
Fig. 4
Cell apoptosis by fluorescence-activated cell sorter (FACS) assay. Representative data of FACS analysis showed that apoptotic cells significantly increased in imatinib mesylate (B) and combined (D) groups. However, rapamycin (C) group showed no significant difference (***P < 0.001 vs. control [A]).
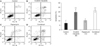
Fig. 5
Morphometric analysis. (A) Mean neointimal cell counts were assessed under the four random high-power fields (×400). Mean neointimal cell counts significantly decreased in all medication groups compared to control (*P < 0.05 vs. PBS, **P < 0.01 vs. PBS). (B) Neointimal cross-sectional area was not significantly different among the groups. (C) The ratio of intima and media thickness was not significantly different among the groups.
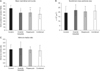
Fig. 6
Cell Proliferation Index. Immunohistochemical staining (×400) against proliferating cell nuclear antigen (PCNA) showed that PCNA-positive cells were significantly decreased in all medication groups compared to control, which represented that cell proliferation was inhibited by each drug medication (***P < 0.001 vs. control).
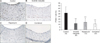
ACKNOWLEDGEMENTS
This work was supported by grants from the SNUH Research Fund (0420080870) and partly from the SNUH Research Fund (1120080006).
References
1. Bauters C, Isner JM. The biology of restenosis. Prog Cardiovasc Dis. 1997; 40:107–116.
2. Mitra AK, Gangahar DM, Agrawal DK. Cellular, molecular and immunological mechanisms in the pathophysiology of vein graft intimal hyperplasia. Immunol Cell Biol. 2006; 84:115–124.
3. Moses JW, Leon MB, Popma JJ, Fitzgerald PJ, Holmes DR, O'Shaughnessy C, et al. Sirolimus-eluting stents versus standard stents in patients with stenosis in a native coronary artery. N Engl J Med. 2003; 349:1315–1323.
4. Gregory CR, Huie P, Billingham ME, Morris RE. Rapamycin inhibits arterial intimal thickening caused by both alloimmune and mechanical injury. Its effect on cellular, growth factor, and cytokine response in injured vessels. Transplantation. 1993; 55:1409–1418.
5. Buchdunger E, Cioffi CL, Law N, Stover D, Ohno-Jones S, Druker BJ, et al. Abl protein-tyrosine kinase inhibitor STI571 inhibits in vitro signal transduction mediated by c-kit and platelet-derived growth factor receptors. J Pharmacol Exp Ther. 2000; 295:139–145.
6. Wang CH, Anderson N, Li SH, Szmitko PE, Cherng WJ, Fedak PW, et al. Stem cell factor deficiency is vasculoprotective: unraveling a new therapeutic potential of imatinib mesylate. Circ Res. 2006; 99:617–625.
7. Sihvola R, Koskinen P, Myllarniemi M, Loubtchenkov M, Hayry P, Buchdunger E, et al. Prevention of cardiac allograft arteriosclerosis by protein tyrosine kinase inhibitor selective for platelet-derived growth factor receptor. Circulation. 1999; 99:2295–2301.
8. Lassila M, Allen TJ, Cao Z, Thallas V, Jandeleit-Dahm KA, Candido R, et al. Imainib attenuates diabetes-associated atherosclerosis. Arterioscler Thromb Vasc Biol. 2004; 24:935–942.
9. Vamvakopoulos JE, Petrov L, Aavik S, Lehti S, Aavik E, Hayry P. Synergistic suppression of rat neointimal hyperplasia by rapamycin and imatinib mesylate: implications for the prevention of accelerated arteriosclerosis. J Vasc Res. 2006; 43:184–192.
10. Park KW, Yang HM, Youn SW, Yang HJ, Chae IH, Oh BH, et al. Constitutively active glycogen synthase kinase-3beta gene transfer sustains apoptosis, inhibits proliferation of vascular smooth muscle cells, and reduces neointima formation after balloon injury in rats. Arterioscler Thromb Vasc Biol. 2003; 23:1364–1369.
11. Klouche M, Peri G, Knabbe C, Eckstein HH, Schmid FX, Schmitz G, et al. Modified atherogenic lipoproteins induce expression of pentraxin-3 by human vascular smooth muscle cells. Atherosclerosis. 2004; 175:221–228.
12. Johnson C, Galis ZS. Matrix metalloproteinase-2 and -9 differentially regulate smooth muscle cell migration and cell-mediated collagen organization. Arterioscler Thromb Vasc Biol. 2004; 24:54–60.
13. Kim HS, Cho HJ, Cho HJ, Park SJ, Park KW, Chae IH, et al. The essential role of p21 in radiation-induced cell cycle arrest of vascular smooth muscle cell. J Mol Cell Cardiol. 2004; 37:871–880.
14. Gown AM, Willingham MC. Improved detection of apoptotic cells in archival paraffin sections: immunohistochemistry using antibodies to cleaved caspase 3. J Histochem Cytochem. 2002; 50:449–454.
15. Junqueira LC, Bignolas G, Brentani RR. Picrosirius staining plus polarization microscopy, a specific method for collagen detection in tissue sections. Histochem J. 1979; 11:447–455.
16. Puchtler H, Waldrop FS, Valentine LS. Polarization microscopic studies of connective tissue stained with picro-sirius red FBA. Beitr Pathol. 1973; 150:174–187.
17. Suh JW, Park JS, Cho HJ, Kim MS, Kang HJ, Cho YS, et al. Sirolimus-eluting stent showed better one-year outcomes than paclitaxel-eluting stent in a real life setting of coronary intervention in Koreans. Int J Cardiol. 2007; 117:31–36.
18. Stone GW, Ellis SG, Cannon L, Mann JT, Greenberg JD, Spriggs D, et al. Comparison of a polymer-based paclitaxel-eluting stent with a bare metal stent in patients with complex coronary artery disease: a randomized controlled trial. JAMA. 2005; 294:1215–1223.
19. Dawkins KD, Grube E, Guagliumi G, Banning AP, Zmudka K, Colombo A, et al. Clinical efficacy of polymer-based paclitaxel-eluting stents in the treatment of complex, long coronary artery lesions from a multicenter, randomized trial: support for the use of drug-eluting stents in contemporary clinical practice. Circulation. 2005; 112:3306–3313.
20. Min SK, Kenagy RD, Clowes AW. Induction of vascular atrophy as a novel approach to treating restenosis. A review. J Vasc Surg. 2008; 47:662–670.
21. Vallieres K, Petitclerc E, Laroche G. On the ability of imatinib mesylate to inhibit smooth muscle cell proliferation without delaying endothelialization: an in vitro study. Vascul Pharmacol. 2009; 51:50–56.
22. Matter CM, Rozenberg I, Jaschko A, Greutert H, Kurz DJ, Wnendt S, et al. Effects of tacrolimus or sirolimus on proliferation of vascular smooth muscle and endothelial cells. J Cardiovasc Pharmacol. 2006; 48:286–292.
23. Fandrich F, Ruhnke M, Dresske B, Kremer B. Tolerance-inducing strategies in transplantation surgery-current status and perspectives. Langenbecks Arch Surg. 2004; 389:60–66.
24. Nasuno A, Toba K, Ozawa T, Hanawa H, Osman Y, Hotta Y, et al. Expression of coxsackievirus and adenovirus receptor in neointima of the rat carotid artery. Cardiovasc Pathol. 2004; 13:79–84.
25. Ruygrok PN, Webber B, Faddy S, Muller DW, Keogh A. Angiographic regression of cardiac allograft vasculopathy after introducing sirolimus immunosuppression. J Heart Lung Transplant. 2003; 22:1276–1279.
26. Uitto J, Kouba D. Cytokine modulation of extracellular matrix gene expression: relevance to fibrotic skin diseases. J Dermatol Sci. 2000; 24:Suppl 1. S60–S69.
27. Distler JH, Jungel A, Huber LC, Schulze-Horsel U, Zwerina J, Gay RE, et al. Imatinib mesylate reduces production of extracellular matrix and prevents development of experimental dermal fibrosis. Arthritis Rheum. 2007; 56:311–322.
28. Zhu J, Wu J, Frizell E, Liu SL, Bashey R, Rubin R, et al. Rapamycin inhibits hepatic stellate cell proliferation in vitro and limits fibrogenesis in an in vivo model of liver fibrosis. Gastroenterology. 1999; 117:1198–1204.
29. Poulalhon N, Farge D, Roos N, Tacheau C, Neuzillet C, Michel L, et al. Modulation of collagen and MMP-1 gene expression in fibroblasts by the immunosuppressive drug rapamycin. A direct role as an antifibrotic agent? J Biol Chem. 2006; 281:33045–33052.
30. Abdollahi A, Li M, Ping G, Plathow C, Domhan S, Kiessling F, et al. Inhibition of platelet-derived growth factor signaling attenuates pulmonary fibrosis. J Exp Med. 2005; 201:925–935.