Abstract
Purpose
The aim of this study was to establish an in vitro method to purify human multipotent adult progenitor cells (hMAPCs) and assess their possible differentiation into hepatocytes by coculture with human hepatocyte line L02.
Methods
hMAPCs were isolated by magnetic activated cell sorting (MACS) depletion selection using CD45 and GlyA microbeads. After indirect or direct coculture of hMAPCs and human hepatocyte line L02, the expression of albumin (ALB), alpha-fetoprotein (AFP), cytokeratin (CK) 18, and CK19 by hMAPCs was detected by immunocytochemistry.
Results
With the MACS method, (5-10) × 104/mL hMAPCs could be separated from 1 × 106/mL bone marrow mononuclear cells. The purity of CD45-/GlyA- cells separated from bone marrow adherent cells was more than 98%, as determined by flow cytometry. In the coculture without cell-to-cell contact, hMAPCs expressed high AFP on day 1, and then tapered daily to low expression on day 7; ALB expression reached its peak on day 5, and remained high on day 7; CK18 was initially expressed on day 5 and was higher on day 7; CK19 was negative in all assays. In the coculture with cell-to-cell contact, ALB and CK18 were expressed by most cells while AFP appeared in only a few on day 5.
Human multipotent adult progenitor cells (hMAPCs), a subgroup of highly-purified mesenchymal stem cells (MSCs), can differentiate into hepatocytes [1,2] and thus have important potential for future clinical applications. At present, common methods for inducement of differentiation of hMAPCs to hepatocytes include use of cellular factors and pathological serum [3,4], which require long periods of time to produce mature phenotypic hepatocytes, and therefore do not meet the requirements of hybrid bioartificial liver (HBAL), which calls for production of a large quantity of hepatocytes having specific functions in a short time period. In addition, the practical application of such methods is also restricted due to the high cost of cellular factors. As another method to induce stem cell differentiation, coculture is advantageous because of its low cost. Although the method lacks previous intensive investigation and a clear description of the mechanisms involved, it has been shown previously that embryonic stem cells (ESCs) cocultured with hepatocytes express premature hepatocyte phenotypes in 2 days, and that this was more effective than methods using cellular factors requiring 15 days [5]. Meanwhile, cell proliferation may be promoted by the mutual support of cells in contact in coculture [6]. In a word, coculture methods could fulfill the requirements of HBAL because of the short time required to induce stem cells to differentiate into massive quantities of relatively mature hepatocytes for relatively low cost. In this study, the possibility of differentiating hMAPCs into hepatocytes by coculture with human hepatocyte line L02 in vitro was assessed, in order that new resources for HBAL and hepatocyte transplantation might be provided.
Bone marrow tissues were obtained by aspirating proper volumes of bone marrow from volunteers; mononuclear cells were isolated by gradient and density centrifugation, and were then cultivated by adherent culture. Bone marrow adherent cells were collected and isolated by magnetic activated cell sorting (MACS) depletion selection using CD45 and GlyA microbeads. The purity of CD45- and GlyA- cell isolates was determined by flow cytometry, according to previously reported methods [7]. Expression of CD29, CD44, CD34, and human leukocyte antigen (HLA)-DR (Sigma-Aldrich Co., St. Louis, MO, USA) by hMAPCs determined by flow cytometry.
The human hepatocyte line L02 (Shanghai Cytobiology Institution) was cocultured indirectly with hMAPCs in order to induce differentiation into hepatocyte-like cells. In brief, hMAPCs and L02 hepatocytes were spread on separate cover slips, both at 1 × 105/mL, and then were put together into a culture dish (10 cm) in medium containing 5% fetal calf serum (FCS) and no leukemia inhibitory factor. During cell cultivation and inducement, morphology changes were observed using an inverted phase contrast microscope. The expressions of albumin (ALB), alpha-fetoprotein (AFP), cytokeratin (CK) 18, and CK19 (Sigma-Aldrich Co.) on hMAPCs were detected by immunocytochemical analysis at different time points (after 1, 3, 5, and 7 days of indirect coculture). Separate cultures of L02 hepatocytes and hMAPCs served as the positive and negative controls, respectively. The start of coculture was defined as Day 0. Semiquantitative immunocytochemical findings were obtained by the following method [8]: 5 representative cells/high power field (×200) were chosen for each experimental sample, cells were counted, and the percentage of positive cells was ascertained. Take the mean value and take every 20% as a "+".
hMAPCs were labeled by 5,6-carboxyfluorescein diacetate succinimidyl ester (green, Molecular Probes, a division of Life Technologies, Carlsbad, CA, USA) and then were cocultured with L02 cells (1 × 104/mL of each cell type). Subsequently, the mixed cells were seeded on a spearal dish for detection by laser scanning confocal microscope (LSCM) (TCSP-II, Leica Microsystems, Wetzlar, Germany). Strept Avidin-Biotin Complex (SABC)-Cy3 (Boshide Co., Wuhan, China) was used for double indirect immunofluorescence staining. Experiments were performed using standard methods and manufacturers' protocols. The expression of ALB, AFP, and CK18 on hMAPCs was determined by LSCM: nondifferentiated hMAPCs were green; positive, differentiated hMAPCs/hepatocytes were yellow; and control L02 cells were red.
When cultivated alone, hMAPCs looked like endothelial cells (shuttle-shaped) or like fibroblasts (oblong-shaped) (Fig. 2A). In both coculture conditions (direct and indirect), the MAPCs became spindle-like, irregular, circular or polygonal with the passage of time, similar to the L02 hepatocytes (Fig. 2B, C).
As shown in Table 1, expression of ALB, AFP, CK18, and CK19 on hMAPCs was detected by immunocytochemistry at different time points (after 1, 3, 5, and 7 days of indirect culture). On day 1, hMAPCs expressed high AFP, as do premature hepatocytes (Fig. 3A). The expression of ALB was very weak, while CK18 was not expressed on day 1 (data not shown). On day 3, ALB (expressed by mature hepatocytes) increased while AFP decreased (data not shown). On day 5, ALB reached its peak (Fig. 3B) while AFP continued to decrease, and the initial expression of CK18 (also expressed by mature hepatocytes) occurred. On day 7, ALB remained high and CK18 increased (Fig. 3C), whereas there was little expression of AFP.
In the direct coculture of hMAPCs and human hepatocyte line L02 (with cell-to-cell contact), on day 5, the expression of ALB, AFP, and CK18 on hMAPCs was determined by LSCM (as qualitative observation). The yellow immunofluorescence means expression of ALB and CK18 appeared on many cells, while AFP appeared on only a few (Fig. 4A-C).
Since 1999, when Petersen et al. [9] reported for the first time that bone marrow-derived cells could differentiate into hepotacytes, these cells have been the focus of much investigation. Thus far, many such subgroups of marrow-derived cells have been identified [8]. In this study, the subgroup of hMAPCs isolated by MACS depletion selection using CD45 and GlyA microbeads actively proliferated in vitro. Flow cytometric analysis indicated that these isolated and purified hMAPCs were characteristic of MSCs and that they were relatively pure [10].
Methods using cellular factors have been most commonly used to induce stem cells to differentiate into hepatocytes [11,12,13]. Jang et al. [14] cocultured hematopoietic stem cells (HSCs) indirectly with injured liver tissue, separated by a semipermeable membrane, and found that HSCs differentiated into hepatocyte-like cells, indicating a decisive role for the pathological microenvironment in this inducement, but not a course of cellular integration. Does this inducing effect take place in the normal hepatic microenvironment? Yamazaki et al. [15] cocultured mice bone marrow-derived cells with nonparenchymal hepatic cells and detected strong expression of genes specific to hepatocytes. Recently, Lange et al. [16] cocultured mouse original-generation hepatocytes with MSC and induced expression of specific mature hepatocytic phenotypes, and further pointed out that the mechanism involved stimulation by the organ-specific microenvironment. Results of the current study, in which hMAPCs were cocultured both directly and indirectly with a highly differentiated human hepatocyte line, L02, derived from normal hepatocytes of adults, were consistent with previous reports.
In coculture without cell-to-cell contact, hMAPCs expressed high AFP, as do premature hepatocytes. The expression of ALB was very weak, while CK18 was not expressed. On day 3, ALB (expressed by mature hepatocytes) increased while AFP decreased. On day 5, ALB reached its peak while AFP continued to decrease, and the initial expression of CK18 (also expressed by mature hepatocytes) occurred. On day 7, ALB remained high and CK18 increased, whereas there was little expression of AFP. There was no expression of CK19 (expressed by mature biliary duct cells) at any of the time points assayed. These results suggest that, in coculture without cell-to-cell contact, hMAPCs differentiated into relatively mature hepatocyte-like cells in 7 days. This study demonstrated the advantages of coculture, consistent with investigations by other researchers. The microenvironment inducement theory, as an explanation of the mechanism of for lateral differentiation of stem cells, is supported by the current findings.
Later, coculture with cell-to-cell contact was performed. Results showed that ALB- and CK18-producing cells were widespread, while AFP only appeared in a few cells. This indicated that hMAPCs were induced into relatively mature hepatocyte-like cells on the fifth day of direct coculture, even earlier than in indirect coculture, suggesting that, apart from specific features of the microenvironment, contact between cells, which permits signaling communication, may also play a role in this inducement. Another explanation for the earlier differentiation in direct coculture may be the relatively high concentration of cellular factors inducing differentiation in the location of contact. Moore et al. [17] examined the effects of cocultivated hepatocytes on the hepatospecific differentiation of murine ESCs, utilizing an established mouse ESC line expressing high or low levels of E-cadherin. They compared cocultures of cadherin-expressing ESCs (CE-ESCs) with cultured rat hepatocytes, allowing for either paracrine interactions (indirect cocultures) or both juxtacrine and paracrine interactions (direct cocultures, random and patterned). Comparison of single ESC cultures versus cocultures show that direct contact cocultures of hepatocytes and CE-ESCs maximally promoted ESC commitment towards hepatodifferentiation, suggesting cooperative effects of cadherin-based juxtacrine and paracrine interactions. In contrast, E-cadherin deficient mouse ESCs cocultured with hepatocytes failed to show increased G6P expression, confirming the role of E-cadherin expression.
Mizuguchi et al. [6] demonstrated that, when cocultured with hepatocytes, bone marrow-derived matrix cells proliferated more actively, compared to when they are cultured separately, and the functions of hepatocytes were enhanced by coculturing. In light of this finding, we assume that massive proliferation of hMAPCs could occur in vitro. These could then be cocultured with human hepatocyte lines, or an original generation of human hepatocytes. With the development of cell culture methods such as sandwich collagen gel formation [18], we can generate 109 cells differentiated from hMAPCs and having comparatively good functions specific to hepatocytes in a short time period, as required for HBAL.
Figures and Tables
Fig. 1
Expression of CD29, CD44, CD34, and human leukocyte antigen (HLA)-DR on human multipotent adult progenitor cells (hMAPCs). (A) The purity of CD45- GlyA- cells separated from bone marrow adherent cells was more than 98%, as determined by flow cytometry. Expression of CD29 (B), CD44 (C), CD34 (D), and HLA-DR (E) on hMAPCs. Panel F is for negative control. FITC, fluorescein isothiocyanate.
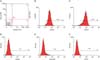
Fig. 2
Morphology observations during human multipotent adult progenitor cell (hMAPC) coculture (A) hMAPC growing morphology (6 generation, ×100). (B) hMAPC morphology on day 7, indirect coculture (×100). (C) Direct coculture morphology on day 5 (×100). (D) L-02 morphology (×100).
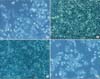
Fig. 3
Immunocytochemistry staining of indirect cocultures. (A) On day 1, indirect coculture, AFP cells (ABC method, DAB developing, ×200), Positive: light brown staining. (B) On day 5, indirect coculture, ALB cells (S-P method AEC developing, ×200), Positive: reddish brown staining. (C) On day 7, indirect coculture, cytokeratin 18 cells (ABC method, DAB developing, ×200), Positive: light brown staining. (D) L02 hepotocytes ALB cells (S-P method, AEC developing, positive control, ×200), Positive: red stain. (E) Undifferentiated human multipotent adult progenitor cells AFP immunocytochemistry (ABC method, DAB developing, no light brown staining, negative control, ×200). ABC, avidin-biotin complex; DAB, diaminobezidin; S-P, streptavidin-perosidase; AEC, 3-amino-9-ethylcarbazole.
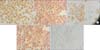
Fig. 4
Immunofluorescence staining of direct cocultures by laser scanning confocal microscope (LSCM). (A) On day 5, direct coculture, ALB immunofluorescence LSCM (×400). (B) On day 5, direct coculture, CK18 immunofluorescence LSCM (×400). (C) On day 5, direct coculture, AFP immunofluorescence LSCM (×400). (D) Separately cultured L02 hepatocytes, ALB immunofluorescence LSCM (positive control, ×400). (E) Separately cultured human multipotent adult progenitor cell (hMAPC), ALB immunofluorescence LSCM (negative control, ×400). CFSE and SABC-Cy3 double staining indirectly, Positive cells: yellow fluorescence. ALB, albumin; AFP, alpha-fetoprotein; CFSE, carboxyfluorescein diacetate succinimidyl ester; SABC, strept avidinbiotin complex; CK18, cytokeratin 18.
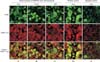
Table 1
Immunocytochemistry results
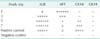
Five representative cells/high power field (×200) for each condition were chosen, and the percentage of positive cells was determined. Mean values were scored as follows: -, 0%; ±, <10%; +, 20%; ++, 30%; +++, 40%; +++++, 50%; +++++, 60%; ++++++, >70%.
ALB, albumin; AFP, alpha-fetoprotein; CK, cytokeratin.
ACKNOWLEDGEMENTS
This work was supported by grants from National Nature Science Foundation of China (No. 81370016), and Midwestern Excellent Young Scientist Foundation of Chinese Medical Doctor Association (2012).
This article was supported by the grant from The Natural Science Foundation of fujian province (2014J01431).
References
1. Li M, Ikehara S. Bone-marrow-derived mesenchymal stem cells for organ repair. Stem Cells Int. 2013; 2013:132642.
2. Prasajak P, Leeanansaksiri W. Developing a new two-step protocol to generate functional hepatocytes from Whartons Jelly-derived mesenchymal stem cells under hypoxic condition. Stem Cells Int. 2013; 2013:762196.
3. Ayatollahi M, Soleimani M, Tabei SZ, Kabir Salmani M. Hepatogenic differentiation of mesenchymal stem cells induced by insulin like growth factor-I. World J Stem Cells. 2011; 3:113–121.
4. Chen XW, Zhu DJ, Ju YL, Zhou SF. Therapeutic effect of transplanting magnetically labeled bone marrow stromal stem cells in a liver injury rat model with 70%-hepatectomy. Med Sci Monit. 2012; 18:BR375–BR382.
5. Jiang Y, Henderson D, Blackstad M, Chen A, Miller RF, Verfaillie CM. Neuroectodermal differentiation from mouse multipotent adult progenitor cells. Proc Natl Acad Sci U S A. 2003; 100:Suppl 1. 11854–11860.
6. Mizuguchi T, Hui T, Palm K, Sugiyama N, Mitaka T, Demetriou AA, et al. Enhanced proliferation and differentiation of rat hepatocytes cultured with bone marrow stromal cells. J Cell Physiol. 2001; 189:106–119.
7. Reyes M, Lund T, Lenvik T, Aguiar D, Koodie L, Verfaillie CM. Purification and ex vivo expansion of postnatal human marrow mesodermal progenitor cells. Blood. 2001; 98:2615–2625.
8. Zhang L, Ye JS, Decot V, Stoltz JF, de Isla N. Research on stem cells as candidates to be differentiated into hepatocytes. Biomed Mater Eng. 2012; 22:105–111.
9. Petersen BE, Bowen WC, Patrene KD, Mars WM, Sullivan AK, Murase N, et al. Bone marrow as a potential source of hepatic oval cells. Science. 1999; 284:1168–1170.
10. Monga SP, Tang Y, Candotti F, Rashid A, Wildner O, Mishra B, et al. Expansion of hepatic and hematopoietic stem cells utilizing mouse embryonic liver explants. Cell Transplant. 2001; 10:81–89.
11. Ouyang J, Shao J, Zou H, Lou Y, Yu Y. Hepatic differentiation of rat mesenchymal stem cells by a small molecule. ChemMedChem. 2012; 7:1447–1452.
12. Tan Y, Xiao EH, Xiao LZ, Yuan YH, Ma C, Shang QL, et al. VEGF(165) expressing bone marrow mesenchymal stem cells differentiate into hepatocytes under HGF and EGF induction in vitro. Cytotechnology. 2012; 64:635–647.
13. Magner NL, Jung Y, Wu J, Nolta JA, Zern MA, Zhou P. Insulin and IGFs enhance hepatocyte differentiation from human embryonic stem cells via the PI3K/AKT pathway. Stem Cells. 2013; 31:2095–2103.
14. Jang YY, Collector MI, Baylin SB, Diehl AM, Sharkis SJ. Hematopoietic stem cells convert into liver cells within days without fusion. Nat Cell Biol. 2004; 6:532–539.
15. Yamazaki S, Miki K, Hasegawa K, Sata M, Takayama T, Makuuchi M. Sera from liver failure patients and a demethylating agent stimulate transdifferentiation of murine bone marrow cells into hepatocytes in coculture with nonparenchymal liver cells. J Hepatol. 2003; 39:17–23.
16. Lange C, Bassler P, Lioznov MV, Bruns H, Kluth D, Zander AR, et al. Hepatocytic gene expression in cultured rat mesenchymal stem cells. Transplant Proc. 2005; 37:276–279.
17. Moore RN, Dasgupta A, Rajaei N, Yarmush ML, Toner M, Larue L, et al. Enhanced differentiation of embryonic stem cells using co-cultivation with hepatocytes. Biotechnol Bioeng. 2008; 101:1332–1343.
18. Choi HJ, Choi D. Successful mouse hepatocyte culture with sandwich collagen gel formation. J Korean Surg Soc. 2013; 84:202–208.