Abstract
Purpose
To determine the optimum culture conditions by investigating isolated rat hepatocytes cultured in medium containing different glucose concentrations.
Methods
Hepatocytes were isolated from rats using a two-step perfusion technique and divided into the following two groups cultured in medium containing different glucose concentrations: (1) low-glucose group and (2) high-glucose group. Total cell count and viability of cultured rat hepatocytes and liver function parameters (i.e., concentrations of albumin, ammonia, and urea in the culture medium) were measured. The morphology of cultured rat hepatocytes was examined by staining with hematoxylin and eosin, and albumin receptor expression was confirmed by immunofluorescence.
Results
Total cell count and viability showed smaller increases in the low-glucose group than the high-glucose group, although the difference was not statistically significant (P = 0.112 and P = 0.147, respectively). The levels of albumin (P = 0.943), ammonia (P = 0.744), and urea (P = 0.709) were not significantly different between the two groups. In both groups, the function of cultured hepatocytes decreased significantly over time. The morphology of hepatocytes was well maintained in both groups at 3 days. On day 7, the cytoplasm was transformed into a spindle shape. On day 10, these changes were exaggerated, and were more prominent in the high-glucose group.
Conclusion
Morphological assessment indicated that low-glucose culture medium is better than high-glucose culture medium for culturing of hepatocytes, although there was not significantly different in functional assessment. The cultured hepatocytes with low-glucose culture medium could be maintained for 7 days.
Acute liver failure is a life-threatening disease, and is encountered commonly in clinical practice [1]. Although various treatment methods have been developed, the results were disappointing and liver transplantation remains the only definitive treatment [2,3]. However, not all patients with acute liver failure are able to receive liver transplantation in time because of the lack of donor organs. The problems of liver transplantation include high mortality and morbidity rates related to the surgery as well as the requirement for long-term immunosuppressant use. Upon recovering from acute liver failure, patients generally do not suffer any sequelae [4]. Therefore, alternative treatments that can provide at least temporary liver support are necessary until spontaneous recovery or a suitable donor liver becomes available.
Hepatocyte transplantation has been investigated as an alternative treatment for acute liver failure patients. Hepatocyte transplantation has several advantages over whole-liver transplantation [5]. A single donor could potentially provide hepatocytes for several patients, and hepatocyte transplantation does not interfere with subsequent liver transplantation. While intact livers can only be transplanted within short time after procurement, hepatocyte may be cryopreserved or cultured for immediate availability in emergencies. Hepatocyte transplantation is a less-invasive treatment than whole-liver transplantation. This minimally invasive treatment can be performed with low morbidity and mortality, a short period of hospitalization, and at low cost.
However, for the clinical application of hepatocyte transplantation, some problems remain to be resolved, such as enabling long-term survival of isolated hepatocytes and preservation without decreasing hepatocyte function [6]. This study was performed to establish the fundamental basis of hepatocyte transplantation for the purpose of clinical application. We examined the ideal culture conditions by investigating isolated rat hepatocytes cultured in media containing different glucose concentrations.
Male Sprague-Dawley rats weighing 200-250 g were used. A 1-week period was allowed prior to the experiment for acclimatization to the laboratory environment. The animal facility had a 12-hour light/dark cycle, ambient temperature of 25℃, and humidity of 40%. All experiments were performed after obtaining approval from the Ethics Committee for animal experiments and in accordance with the animal experimentation guidelines.
Experimental rats were anesthetized by intraperitoneal injection of zolazepam (Zoletil, Virbac, Carros Cedex, France) and xylazine (Rompum, Bayer, Toronto, ON, Canada). The liver was exposed by U-shaped incision, and 1 mL of freshly prepared heparin solution (0.1 mL of heparin [5,000 IU/mL] + 2.4 mL of physiological saline) was injected into the infrarenal inferior vena cava (IVC); the final heparin concentration was 200 IU/mL. The portal vein was catheterized with a 22-gauge intravenous needle 5 mm inferior to the liver, which was immobilized, and used for application of the perfusion solution. In addition, the infrahepatic and suprahepatic IVC were cut.
The two-step perfusion method described by Seglen [7] was employed. First, Ca2+-free medium (Gibco 17701, Life Technologies, Grand Island, NY, USA) was perfused through the portal vein at a rate of 25 mL/min for 10 minutes. Second, Ca2+ and collagenase medium (Gibco 17703, Life Technologies) was perfused through the same route at the same rate. The perfused rat liver was disconnected from the perfusion apparatus and resected (Fig. 1).
The resected liver was transferred to a plastic petri dish containing Hank's Balanced Salt Solution (HBSS; Gibco 14175-095, Life Technologies). The Glisson's capsule of the resected liver was opened. While the liver was being held in the portal region with forceps and gently shaken, the cells were liberated from the connective-vascular tissue. The resulting cell suspension was filtered through a sterile Perlon filter (100 µm) and allowed to sediment for 15 minutes. The supernatant was removed, and the remaining cell suspension was washed with 40 mL of HBSS and dispensed into sterile centrifuge tubes. Other cells, connective tissue, and contaminants were removed by centrifugation at 500 rpm for 5 minutes three times. A trypan blue exclusion test was performed to calculate the number of viable hepatocytes and the liver cells were prepared for primary culture (Fig. 2).
Aliquots of 1.0 × 106 viable cells were seeded in 100 × 15-mm petri dishes (Nunclon 150350, Sigma-Aldrich Co., St. Louis, MO, USA) containing culture medium according to the experimental group. The cells were cultures in Dulbecco's modified Eagle's Medium (DMEM) supplemented with 10% fetal bovine serum, penicillin, and streptomycin at 37℃ in a 5% CO2 incubator. DMEM contains 1,606 mg/L of amino acids, 31.6 mg/L of vitamins, 200 mg/L of calcium chloride, and 3,700 mg/L of sodium bicarbonate. The culture medium was changed on days 3, 7, 10, and 14.
Experiments groups were classified according to the glucose concentration in the medium.
(1) Low-glucose group (n = 6): 1-g/L D-Glucose DMEM (Gibco 11885-084, Life Technologies)
(2) High-glucose group (n = 6): 4.5-g/L D-Glucose DMEM (Gibco 11965-092, Life Technologies)
The cultured rat hepatocytes were evaluated by morphological and functional methods at 3, 7, 10, and 14 days.
Total cell count and viability of cultured rat hepatocytes were measured using the trypan blue exclusion test and a hemocytometer on days 3, 7, 10, and 14.
Total cell count = volume of cells (mL) × 2 (dilution factor) × mean number of cells (stained cells + unstained cells) × 104
Viability (%) = viable cell count (unstained cell count)/total cell count (stained cell count + unstained cell count) × 100.
The concentrations of albumin, ammonia, and urea were measured in culture medium after 3, 7, 10, and 14 days in culture. The albumin concentration was measured by enzyme-linked immunosorbent assay using affinity-purified anti-rat albumin antibody (A110-134A, Bethyl, Montgomery, TX, USA), rat reference serum (RS10-100, Bethyl), and horseradish peroxidase-conjugated antirat albumin detection antibody (A110-134P, Bethyl). The ammonia and urea concentrations were measured 3 days after the addition of 1-mM NH4Cl when replacing the culture medium. Ammonia concentration was measured using an Ammonia Assay Kit (AA0100, Sigma-Aldrich Co.). Urea concentration was determined by coupled enzyme reaction, which yields a colorimetric (570 nm) product proportional to the amount of urea present, using a Sigma Urea Assay Kit (MAK006, Sigma-Aldrich).
The morphology of cultured rat hepatocytes was examined by hematoxylin and eosin staining. The albumin receptor was confirmed by immunofluorescence analysis. The cultured cell suspension was fixed with 10% formalin for 15 minutes at room temperature and incubated for 10 minutes in phosphate-buffered saline containing 0.25% Triton X-100 (PBST) for permeabilization. The cells were incubated with 1% bovine serum albumin in PBST for 30 minutes to block nonspecific antibody binding. Finally, the cells were incubated sequentially with antialbumin antibody (ab106582, Abcam, Cambridge, UK) and rabbit antichicken IgY H&L secondary antibody (ab6749, Abcam).
All data are expressed as means ± standard deviation. Experimental results were tested by one-way analysis of variance with repeated measures and Bonferroni post hoc test. Comparisons between two groups were performed using the Mann-Whitney U test. Statistical analysis was performed using PASW Statistics ver. 18.0 (SPSS Inc., Chicago, IL, USA). In all analyses, P < 0.05 was taken to indicate statistical significance.
Total cell count showed smaller increases in the low-glucose group than the high-glucose group, although the difference was not statistically significant (F(1,10) = 3.045, P = 0.112), and total cell count in the low-glucose group differed significantly between time points (F(3,15) = 14.552, P < 0.001). Total cell count in the low glucose group on day 7 was significantly different from those on days 10 (P = 0.024) and 14 (P = 0.040). There were no significant differences between the two groups at each time point (Fig. 3A).
The viability of cultured rat hepatocytes was greater in the low-glucose group than the high-glucose group, but the difference was not statistically significant (F(1,10) = 2.477, P = 0.147). The viability in the low-glucose group differed significantly between time points (F(3,15) = 6.606, P = 0.005). The viability in the low-glucose group on day 3 was significantly different from that on day 14 (P = 0.025). There were no significant differences between the two groups at any time point (Fig. 3B).
The concentrations of albumin, ammonia, and urea were measured in culture medium as a means of determining hepatocyte function. The albumin level was not significantly different between the two groups (F(1, 10) = 0.005, P = 0.943), but differed significantly between time points in both the low-glucose group (F(1.339, 6.693) = 24.753, P = 0.001) and the high-glucose group (F(1.822, 9.111) = 14.583, P = 0.002). The albumin level in the low-glucose group on day 3 was significantly different from those on day 7 (P = 0.034), 10 (P = 0.017), and 14 (P = 0.018), and that in the high-glucose group on day 7 was significantly different from those on days 10 (P = 0.033) and 14 (P = 0.030) (Fig. 4A).
The ammonia level was not significantly different between the two groups (F(1, 10) = 0.113, P = 0.744), but differed significantly between time points in both the low-glucose group (F(3, 15) = 20.758, P < 0.001) and the high-glucose group (F(3, 15) = 19.309, P < 0.001). The ammonia level in the low-glucose group on day 3 was significantly different from those on days 7 (P = 0.030), 10 (P = 0.005), and 14 (P = 0.012), and that in the high-glucose group on day 3 was significantly different from those on days 7 (P = 0.040), 10 (P = 0.034), and 14 (P = 0.014) (Fig. 4B).
The urea level was not significantly different between the two groups (F(1, 10) = 0.012, P = 0.709), and did not differ significantly between time points in either the low-glucose group (F(1.506, 7.529) = 3.698, P = 0.084) or the high-glucose group (F(1.591, 7.957) = 4.400, P = 0.058) (Fig. 4C).
The results of histological examination indicated that the typical shape of hepatocytes; i.e., as round nucleus, prominent nucleoli, and polygonal cytoplasm, was well maintained in both groups after 3 days in culture. On day 7, the shape of the nucleus was maintained, but the cytoplasm was transformed into a spindle shape. These changes were exaggerated on day 10, and were more prominent in the high glucose group. Although there were no significant morphological differences between the two groups, in the low-glucose group, the cellularity was more abundant and cytoplasm transformed more slowly compared to the high-glucose group (Fig. 5).
Immunofluorescence staining for albumin indicated that there were no significant morphological differences between the two groups. On day 3, strong staining was detected in the cytoplasm in both groups. However, only weak staining was detected on day 7 and there was no staining on day 10 in both groups (Fig. 6).
In 1976, Matas et al. [8] reported that hepatocyte transplantation capable of continuous enzyme production could be an effective treatment for homozygous recessive Gunn rats lacking the enzyme uridine diphosphate glucuronyl transferase. The first hepatocyte transplantation in human subjects was attempted by Mito et al. [9] in 1992. Patients with chronic liver disease received infusion of autologous hepatocytes into the spleen. They confirmed the stability of hepatocyte transplantation. Subsequently, there have been many experimental studies of hepatocyte transplantation, and this procedure has been applied clinically in patients with acute liver failure, liver cirrhosis, enzymatic deficiency, and metabolic disease. Hepatocyte transplantation has been performed mainly in patients with enzymatic deficiency and metabolic disease because good results are expected in such cases, even when accounting for only <5% of the total hepatocyte mass [10].
Hepatocyte transplantation is under investigation as a possible therapeutic alternative for acute liver failure patients, but many problems remain to be resolved before clinical use [6]. The issues currently under investigation include isolation of hepatocytes, long-term survival of the hepatocytes, the conditions necessary to maintain hepatocyte metabolism, the quantity problem, storage, and the route of administration. This study was performed to determine the optimum conditions for isolation and culture of hepatocytes, and the ideal conditions for their long-term survival.
Methods of hepatocyte isolation can be divided into mechanical methods and those that use enzymes. Earlier methods were mostly mechanical, and cell suspension of liver tissue enabled hepatocyte isolation, but most cells were damaged and the survival rate was low. Howard et al. [11] reported the effects of incubation with collagenase, which breaks down liver tissue. Berry and Friend [12] developed the collagenase perfusion method, which enables a uniform distribution in vivo. This method allowed the isolation and culture of hepatocytes with a high survival rate. Seglen [7] studied the ideal conditions and the optimal enzyme concentration for the collagenase perfusion method, and developed the two-step collagenase-perfusion technique. This technique has been adopted widely and was used in the present study. For the clinical application of hepatocyte transplantation, hepatocytes should have viability >60% with an absence of microbial contamination [13]. The greatest obstacle to the clinical application of hepatocyte transplantation is currently the lack of good quality human hepatocytes. The viability of isolated hepatocytes in this study was >80%.
When hepatocytes are cultured in standard cell culture medium in a container with a plastic surface, the cells lose their gap junctions within 12-24 hours and adopt a flattened shape. The unique functions of the tissue are lost in 3-5 days, and the cells die within 1-2 weeks [14]. To improve this short-term survival and instability of function, conditions such as growth factors, nutrients, extracellular matrix, and cell-cell interaction are important. Methods to facilitate these conditions have been developed, such as adding growth factors or hormones to the culture medium [15], culturing cells with matrix-adhesion factors, coating the container surface with collagen, polystyrene, or laminin to act as an extracellular matrix [16], and inducing cell-cell interaction by culturing along with other cell types, such as endothelial cells [17].
The present study was performed to determine the optimal conditions for culturing hepatocytes while maintaining their function and enabling long-term survival. Here, the importance of the glucose concentration in the culture medium was investigated. Culture media are commercially available with a high or low glucose concentration (4.5-g/L and 1.0-g/L glucose, respectively). In the present study, the low-glucose group showed better cell proliferation than the high-glucose group in terms of morphological assessment, although the difference was not statistically significant in terms of functional assessment. The two groups did not differ significantly with respect to hepatocyte function. In both groups, the function of cultured hepatocytes decreased significantly over time. In addition, cell morphology and function were maintained for up to 3 days in culture. After 7 days, both groups show deterioration of cell morphology and reduced cell function. These changes were more prominent in the high glucose group.
Current clinical research uses a method involving isolation of hepatocytes from surgical liver biopsies of patients with benign liver disease or liver cancer, and using these hepatocytes fresh or after cryopreservation when necessary. Therefore, it is important to establish the optimal conditions for isolation and culturing of hepatocytes without adversely affecting their function. This study was limited in that the number of samples was small, and that a methodologically basic method was used. The parameters for investigation the function of cultured hepatocytes were also simple. However, further studies based on these findings may facilitate determination of the optimal conditions for hepatocyte culture. This study was considered to be an important first step in the clinical application of hepatocyte transplantation, although too basic.
In conclusion, the morphological assessment indicated that low-glucose culture medium is better than high-glucose culture medium for culturing of hepatocytes, although there was not significantly different in functional assessment. The cultured hepatocytes with low-glucose culture medium could be maintained for 7 days. Further studies of culture media supplemented with growth factors and hormones are necessary to determine the optimal culture conditions.
Figures and Tables
Fig. 1
Operative view of cannulation in the portal vein (A) and infusion of collagenase solution with whitish discoloration of the liver (B).
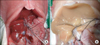
Fig. 2
Evaluation of cell viability by trypan blue exclusion test (×200). Viable cells with an intact plasma membrane exclude dyes, such as trypan blue, whereas damaged cells (arrows) become stained, with particularly strong staining in the nucleus. The viability of isolated hepatocytes in this photograph was about 85%.
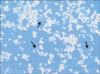
Fig. 3
The changes in total cell count (A) and viability (B) according to glucose concentration in the culture medium. Data are means ± standard error of the mean. Asterisks indicate significant differences between time points (*P < 0.05).
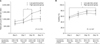
Fig. 4
Changes in liver function, including albumin (A), ammonia (B), and urea (C) levels, according to the glucose concentration in the culture medium. Data are means ± standard error of the mean. Asterisks indicate significant differences between time points (*P < 0.05).
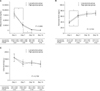
References
1. Gotthardt D, Riediger C, Weiss KH, Encke J, Schemmer P, Schmidt J, et al. Fulminant hepatic failure: etiology and indications for liver transplantation. Nephrol Dial Transplant. 2007; 22:Suppl 8. viii5–viii8.
2. Kjaergard LL, Liu J, Als-Nielsen B, Gluud C. Artificial and bioartificial support systems for acute and acute-on-chronic liver failure: a systematic review. JAMA. 2003; 289:217–222.
3. Demetriou AA, Brown RS Jr, Busuttil RW, Fair J, McGuire BM, Rosenthal P, et al. Prospective, randomized, multicenter, controlled trial of a bioartificial liver in treating acute liver failure. Ann Surg. 2004; 239:660–667.
4. Gill RQ, Sterling RK. Acute liver failure. J Clin Gastroenterol. 2001; 33:191–198.
5. Dhawan A, Puppi J, Hughes RD, Mitry RR. Human hepatocyte transplantation: current experience and future challenges. Nat Rev Gastroenterol Hepatol. 2010; 7:288–298.
6. Hughes RD, Mitry RR, Dhawan A. Current status of hepatocyte transplantation. Transplantation. 2012; 93:342–347.
7. Seglen PO. Preparation of isolated rat liver cells. Methods Cell Biol. 1976; 13:29–83.
8. Matas AJ, Sutherland DE, Steffes MW, Mauer SM, Sowe A, Simmons RL, et al. Hepatocellular transplantation for metabolic deficiencies: decrease of plasms bilirubin in Gunn rats. Science. 1976; 192:892–894.
9. Mito M, Kusano M, Kawaura Y. Hepatocyte transplantation in man. Transplant Proc. 1992; 24:3052–3053.
10. Fox IJ, Chowdhury JR, Kaufman SS, Goertzen TC, Chowdhury NR, Warkentin PI, et al. Treatment of the Crigler-Najjar syndrome type I with hepatocyte transplantation. N Engl J Med. 1998; 338:1422–1426.
11. Howard RB, Christensen AK, Gibbs FA, Pesch LA. The enzymatic preparation of isolated intact parenchymal cells from rat liver. J Cell Biol. 1967; 35:675–684.
12. Berry MN, Friend DS. High-yield preparation of isolated rat liver parenchymal cells: a biochemical and fine structural study. J Cell Biol. 1969; 43:506–520.
13. Hughes RD, Mitry RR, Dhawan A. Hepatocyte transplantation for metabolic liver disease: UK experience. J R Soc Med. 2005; 98:341–345.
14. Nyberg SL, Peshwa MV, Payne WD, Hu WS, Cerra FB. Evolution of the bioartificial liver: the need for randomized clinical trials. Am J Surg. 1993; 166:512–521.
15. Hayashi I, Sato GH. Replacement of serum by hormones permits growth of cells in a defined medium. Nature. 1976; 259:132–134.
16. Matsushita T, Ijima H, Koide N, Funatsu K. High albumin production by multicellular spheroids of adult rat hepatocytes formed in the pores of polyurethane foam. Appl Microbiol Biotechnol. 1991; 36:324–326.
17. Guillouzo A, Beaune P, Gascoin MN, Begue JM, Campion JP, Guengerich FP, et al. Maintenance of cytochrome P-450 in cultured adult human hepatocytes. Biochem Pharmacol. 1985; 34:2991–2995.