Abstract
Purpose
To elucidate the characteristic gene transcription profiles among various hepatic ischemia conditions, immediately transcribed genes and the degree of ischemic injury were compared among total ischemia (TI), intermittent clamping (IC), and ischemic preconditioning (IPC).
Methods
Sprague-Dawley rats were equally divided into control (C, sham-operated), TI (ischemia for 90 minutes), IC (ischemia for 15 minutes and reperfusion for 5 minutes, repeated six times), and IPC (ischemia for 15 minutes, reperfusion for 5 minutes, and ischemia again for 90 minutes) groups. A cDNA microarray analysis was performed using hepatic tissues obtained by partial hepatectomy after occluding hepatic inflow.
Results
The cDNA microarray revealed the following: interleukin (IL)-1β expression was 2-fold greater in the TI group than in the C group. In the IC group, IL-1α/β expression increased by 2.5-fold, and Na+/K+ ATPase β1 expression decreased by 2.4-fold. In the IPC group, interferon regulatory factor-1, osteoprotegerin, and retinoblastoma-1 expression increased by approximately 2-fold compared to that in the C group, but the expression of Na+/K+ ATPase β1 decreased 3-fold.
Major complications of hepatic resection are closely related to intraoperative hemorrhaging. Therefore, reducing intraoperative hemorrhaging is essential for promoting a favorable postoperative prognosis. Intermittent clamping (IC, Pringle maneuver) and ischemic preconditioning (IPC) have generally been used for this purpose. IC involves the repetition of long ischemic periods combined with short reperfusion periods. IPC consists of an ischemic period followed by a short reperfusion before a second, prolonged ischemic period.
Hepatic ischemia-reperfusion injury (IRI), which refers to damage occurring when the blood supply returns to the liver after a period of ischemia, is an inevitable problem after IC and IPC. Hepatic IRI consists of a series of complex pathophysiological processes [1,2]. However, the precise mechanism of hepatic IRI remains unclear. Temporal ischemia is not necessarily a cause of IRI, but it can protect against hepatic IRI [3]. For example, an endogenous protective effect of IPC against IRI after sublethal ischemia and reperfusion was reported for myocardial tissue [1]. Extensive studies have been performed to reveal the mechanism of IRI and the protective effect of temporal ischemic conditions [2,4-9]. Unfortunately, traditional biochemical approaches have been unsuccessful.
cDNA microarray is a novel genomic research method that permits simultaneous parallel expression analysis of multiple genes. In addition, it provides useful genetic information for several physiologic conditions [10,11]. Several reports have examined the association of genomic information with IRI and the protective effects of IC and IPC [10-12].
The focus of the current study was to compare 1) the expression of immediately transcribed genes using cDNA microarray and 2) the degree of ischemic injury by histopathologic examination among various rat hepatic ischemia models that mimic the conditions of clinical partial hepatectomy in humans.
Experimental animals were maintained in a pyogen-free (SPF) animal facility at the Clinical Research Center, Yonsei University College of Medicine, under constant room temperature (22℃) and humidity (55%), with a supply of sterile drinking water and sterile animal feed according to the standards of the Association of Assessment and Accreditation of Laboratory Animal Care International. In addition, animal experiments were conducted after approval (approval number; 04-086) of the experiment procedures by the ethical animal experiment guideline established by the Department of Experimental Animals, Clinical Medical Research Center, Yonsei University College of Medicine.
All experiments were performed using 5-week-old male Sprague-Dawley rats weighing 150 to 200 g. The rats were housed under constant environmental conditions with a 12-hour light-dark cycle and fed a laboratory diet of water and rat chow. Twelve rats were randomly and evenly divided into 4 groups as follows: control (C), total ischemia (TI), IC, and IPC.
The C group underwent a sham operation without vascular clamping. The TI group underwent continuous vascular clamping for 90 minutes. The IC group underwent six cycles of intermittent vascular clamping with 15 minutes of ischemia and 5 minutes of reperfusion. The IPC group underwent vascular clamping for 90 minutes after 15 minutes of clamping followed by 5 minutes of reperfusion (Fig. 1).
All surgical procedures were performed under general anesthesia using 30 mg/kg Zoletil (Virbac, Carros, France) via intraperitoneal injections. During anesthesia, body temperature was maintained between 36.5℃ and 37.5℃ using a heating pad. After a midline laparotomy, the falciform ligament was sectioned. For visualization of the hepatic hilum, traction of the duodenum and the middle lobe (ML)/left lateral lobe (LLL) was performed towards the left and cranial direction in that order. After dissection of the hepatic hilum, branches of the portal triad to the ML/LLL were identified and prepared for clamping. All structures in the portal triad to the ML/LLL were occluded by a microvascular clamp (Aesculap, San Francisco, CA, USA) according to specified protocols. Color changes of the ML/LLL were confirmed after clamping the triad to the ML/LLL. During the ischemic period, the abdomen was temporarily closed, and portal blood flow to the right and caudate lobes was maintained. Immediately after each round of ischemia, the ML/LLL were resected in all rats and prepared for histopathologic examination and microarray.
Blood samples were taken from caudal vessels for liver function tests after hepatic resections. Supernatant was sampled after an immediate centrifugation of blood samples at 4,500 rpm for 10 minutes. Using an automated biochemical analyzer (30FR, Toshiba, Tokyo, Japan), total serum protein and albumin levels were measured using biuret and bromocresol green methods, respectively. Bilirubin levels were evaluated using the Jendrassik-Grof method at 600 nm. Alkaline phosphatase levels were estimated using the p-nitrophenyl phosphate substrate method at 405 nm. Aspartate transaminase and alanine transaminase levels were measured using an ultraviolet-rate method at 340 nm.
The excised ischemic lobes were fixed using 10% formalin and embedded in paraffin. Tissues were cut into 5-µm-thick sections and stained using either hematoxylin-eosin (H-E) or terminal deoxyuridine triphosphate nick end labeling (TUNEL). All histopathologic assessments were performed by a pathologist who was blinded to the treatment protocols.
The degree of liver damage was analyzed using light microscopy under ×400 magnification by a point-counting method as follows: grade 0, minimal or no evidence of injury; grade 1, mild injury consisting of cytoplasmic vacuolation and focal nuclear pyknosis; grade 2, moderate to severe injury with extensive nuclear pyknosis, cytoplasmic hypereosinophilia, and loss of intercellular borders; grade 3, severe necrosis with the disintegration of hepatic cords, hemorrhage, and neutrophil infiltration [13].
Cleavage of genomic DNA during apoptosis results in DNA strand breaks that can be identified by labeling free 38-hydroxyl ends with modified nucleotides in an enzymatic reaction involving terminal deoxynucleotidyl transferase [14-16]. The prepared paraffin-embedded sections were stained using a commercially available kit (Apop Tag Peroxidase In Situ Apoptosis Detection Kit S7100; Chemicon International Inc., Billerica, MA, USA) according to the manufacturer's protocols. Hepatocytes were examined using light microscopy under ×400 magnification. Morphometric analysis of the fluorescent cells was performed to determine the number of TUNEL-positive (apoptotic) cells in 25 random fields.
Each fragment of rat liver was lysed and homogenized. RNA was extracted from each lysate by using RNeasy kits (Qiagen, Basel, Switzerland) according to the manufacturer's protocols. RNA quality was assessed using ultraviolet absorption at 260/280 nm by spectrophotometry and gel electrophoresis. After confirming that all samples had 18S and 28S rRNA peaks without degradation, prepared RNA from each rat was pooled [17].
cDNA microarray was performed using four CodeLink™ Bioarray UniSet Rat I Chips (Amersham Biosciences, Piscataway, NJ, USA) that were designed to report on 10,000 genes in the rat genome. Every procedure was performed according to the manufacturer's protocols as follows. Total RNA was reverse-transcribed into single-strand cDNA. Afterward, the second strand was used to synthesize double-stranded cDNA. Priming with anchored oligo(dT) directed the start of cDNA synthesis from the 5' end of the poly(A) tail. During reverse transcription, the cDNA strands of the C group and experimental groups were labeled by cyanine (Cy)-3 and Cy-5 fluorescent dyes, respectively. The labeled cDNA was hybridized to the prepared cDNA chip (CodeLink Bioarray UniSet Rat I) at 58℃ in water for 16 hours and then washed with hybridization wash buffer, followed by a subsequent wash with chemiluminescence rinse buffer.
Fluorescence intensities were captured and converted to numerical values using a ScanArray Lite Microarray Analysis System (Packard Biochip Technologies, Billerica, MA, USA). Signal intensities were imported into GenPlex ver. 3.0 (Istech, Goyang, Korea) and normalized to reduce interarray variation in hybridization intensity after filtering out probe sets with unreliable intensity values. Expression changes were presented as fold changes in comparison with the control group intensities. Then, the expression intensities were transformed into log2 values. Finally, the genes with greater than 2-fold changes in transcription in the cDNA microarray were identified.
All data were expressed as means ± standard deviation and analyzed using SPSS ver. 17.0 (SPSS Inc., Chicago, IL, USA). The Kruskal-Wallis test was used for comparing liver function tests and histopathologic findings. Tukey's post hoc test was used for analysis between groups. A value of P < 0.05 (two-sided tests) was considered statistically significant.
There were no significant differences in liver function among the groups for all parameters tested (P > 0.05) (Table 1).
The C group had minimal or no evidence of injury (grade 0), whereas the experimental groups exhibited grade 1 injury (Fig. 2).
The number of TUNEL-positive cells was significantly higher in the experimental groups than in the C group (2.0 ± 1.4, 57.0 ± 23.9, 7.7 ± 9.4, and 21.0 ± 22.3 in C, TI, IC, and IPC groups, respectively; P < 0.05 for all three groups [Figs. 3, 4]). Among the experimental groups, the TI group had significantly more positive hepatocytes than the other two groups.
Interleukin (IL)-1β expression was 2-fold higher in the TI groups than in the C group (log2 TI/C = 1.05). Compared to the expression in the C group, IL-1α/IL-1β expression was 2-fold higher in the IC group (log2 IC/C = 1.31 and 1.05), but Na+/K+ ATPase β1 expression was 2-fold lower (log2 IC/C = -1.26).
In the IPC group, the expression of interferon regulatory factor 1 (IRF-1) was 2-fold higher than that in the C group (log2 IPC/C = 1.21; Table 2, Fig. 5); conversely, Na+/K+ ATPase β1 expression was decreased by approximately 50% (log2 IPC/C = -1.58). The expression of both tumor necrosis factor receptor superfamily (member 11b; osteoprotegerin (OPG); log2 IPC/C = 1.03) and retinoblastoma (Rb)1 expression (log2 IPC/C = 1.16) was approximately 2-fold higher in the IPC group than in the C group.
This study was performed to identify the degree of ischemic injury and changes in gene transcription in various rat hepatic ischemia models. These models were chosen because they reflect the ischemic conditions of human hepatectomy including IC and IPC. In liver function tests and histopathologic examinations in the current study, there were no significant differences among the different ischemic conditions. We believe it is reasonable outcome because every sample was obtained just after the end of stimuli. However, even though there was no significant difference between groups, higher liver enzyme level was expected in TI group. Moreover the liver enzyme level of control C group was slightly higher than reference value of Sprague-Dawley Rats. Even though it is not considerable difference, we cannot completely rule out the bias related with hepatotoxicity caused by hypoxemia or anesthetics. However, TUNEL staining may distinguish differences in the degree of ischemia. It implies IC and IPC model may militate in favor of hepatocyte survival in comparison to TI model.
In previous studies, liver function tests and histopathologic examinations were useful for comparing the effects of IPC and TI [18]. Likewise, TUNEL staining has been considered a feasible method to assess the degree of apoptosis in hepatic ischemia [19]. However, those studies had reperfusion periods of 1 to 6 hours before sampling specimens after the ischemic periods, and that period may allow for more cellular changes than observed in the current study. This type of study design uses another reperfusion period before sampling specimens, which is different from the procedures used clinically in humans. Therefore, the appropriateness of biochemical and histopathologic examinations and TUNEL staining to assess immediate differences between IC and IPC through 90 minutes of hepatic ischemia remains to be evaluated in future studies.
The cDNA microarray used in the current study is a powerful method for revealing altered gene transcription in early and delayed IPC [20]. Early IPC protects the tissue within minutes of reperfusion, but the protection is only maintained for 1 to 2 hours. It is considered a process independent of protein synthesis and relies on preexisting substances as effectors [20]. The delayed preconditioning exerts its effect 24 hours after reperfusion and this effect may last for several days. It is thought that the delayed IPC relies on altered gene expression, resulting in the production of new proteins. A few reports have investigated gene transcription in IPC using cDNA microarrays [10,11,21,22]. Chen et al. [21] compared gene transcription between C and IPC groups using rats. Similarly as the current study, they only analyzed differences of 2-fold or greater in gene expression. Forty-three genes with significantly altered expression patterns were discovered in their report. The current study revealed the following alterations in gene expression: IL-1β in the TI group, IL-1α/β and Na+/K+ ATPase β1 in the IC group, and IRF-1, OPG, Rb1, and Na+/K+ ATPase β1 in the IPC group. There were no common alterations of gene transcription between our study and Chen's study [21]. Chen et al. [21] used 150 minutes of reoxygenation time following IPC. Conversely, in the current study, the liver lobes were resected immediately after the stimulus was discontinued. The time difference between the two studies should be considered when comparing the results. The resulting changes in gene expression in both studies may contribute to delayed IPC, but the genes with altered expression in the current study are more likely to act as triggers rather than mediators of IPC. IRI is believed to result from the activation of the proinflammatory cascade. Although no common alteration in gene expression was observed in the two studies, IL-10, a well-known anti-inflammatory cytokine, was significantly overexpressed in Chen's report [21]. In the current study, IL-1α and IL-1β, two proinflammatory cytokines, were overexpressed in the TI and IC groups, which is consistent with Chen's results. The transcription of IL-1α in the TI group was 1.7-fold greater than that in the C group in the current study.
In the current study, the β subunit of Na+/K+ ATPase was underexpressed. Na+/K+ ATPase was also underexpressed in a previous study with a design similar to that of the current study. Navarro-Sabate et al. [11] profiled the gene expression patterns associated with long, cold ischemia and IPC. In that study, a number of genes were involved in cellular physiologic processes that were downregulated. Decreased Na+/K+ ATPase activity may contribute to apoptosis in ischemic hepatocytes, preserving ATP [23]. ATP preservation prevents cell necrosis but appears to provide the necessary substrate for cells to undergo apoptosis instead [24]. This switch from necrosis to apoptosis may be beneficial for cell survival. However, this hypotheses requires further investigation.
Originally, apoptosis was believed to be completely different from necrosis. However, they were recently identified to have a shared intracellular pathway called necroapoptosis [15,25]. Therefore, proapoptotic gene transcription is not necessarily protective against IRI and is now thought to be a potential risk. In fact, increased expression of B cell lymphoma (Bcl)-2, a well-known anti-apoptotic gene, was observed after IPC in the previous study [10]. Conversely, the expression of Rb1 was prominent in the current study. Rb1 was known as a proapoptotic, tumor suppressor gene [26]. However, the recent evidences have demonstrated the existence of additional, cell type specific Rb1-encoded protein functions in cellular differentiation and survival [26]. Therefore the effect of over-expression of Rb1 can vary depending on the cellular circumstances. Especially, under stress such as hypoxia, apoptosis decreased caused by Rb1 loss [26].
The results of the current study, including overexpression of IL-1α and IL-1β in the TI and IC groups, appear consistent with those of Barrier's study [10]. Barrier et al. [10] used cDNA microarrays to compare global gene expression in liver biopsies from living human liver donors who did or did not undergo IPC just before liver devascularization. The IPC group exhibited significant overexpression of an IL-1 receptor antagonist (IL-1Ra) and Rb1. IL-1R is known to inhibit the effects of IL-1α and IL-1β by competing for type I and type II IL-1 receptors, resulting in reduced inflammation [27].
OPG is a member of the tumor necrosis factor receptor superfamily [28]. High OPG level is believed to be an important factor of atherosclerosis associated with vascular calcification [29]. However, OPG could promote cell survival by inhibiting tumor necrosis factor-related apoptosis [30]. The over-expression of OPG in current study is consistent with the previous studies.
The results of the current study were different from previous studies with regard to methodology. However, the characteristics of altered gene expression were consistent with those of other studies. In both the current and previous studies, genes that were overexpressed in the IPC group commonly exerted antiapoptotic and anti-inflammatory effects.
It is not simple to determine whether IPC or IC is the more beneficial procedure for liver surgery. Each method has benefits and drawbacks. IPC has advantages in terms of less intraoperative blood loss and a shorter operation time than IC. However, for longer periods of operation, IC was superior in several studies [3,19]. In addition, the protective effect of IC was superior to that of IPC in steatotic patients [2]. One cannot determine the superiority of these procedures based on the current study because it was not designed for that purpose. Nevertheless, the overexpression of IL-1α and IL-1β in the IC group is considered a result of IRI. Conversely, the OPG, Rb1, and Na+/K+ ATPase β1 genes, which had altered expression in the IPC group, were related to the mechanism protecting against IRI. It can be postulated that the IC group underwent IRI due to repeated reperfusion, but this requires further evaluation.
The current study has several limitations. The cDNA microarray analysis was performed only once because of the relatively small number of rats. Repeated microarrays are needed for reliability. Moreover, clustering analysis was not performed due to lack of repetitive microarrays. However, we consider this study as a pilot study, and we expect better outcomes in additional study based on this study. In addition, we used 2-fold gene expression change as a cutoff. We think outcomes come from the higher cutoff value are potentially more important genes. Moreover we used pooled method [17]. We believe that may be a factor to improve the limitation from the small number of specimens.
In conclusion, the current study provided valuable information about the clinical temporal hepatic occlusion method for studying immediate hepatic changes in various rat ischemia models, including 90 minutes of ischemia, because of the similarity of the protocols.
Figures and Tables
Fig. 1
Experimental design. The white area represents reperfusion time, and the black area represents ischemia time. C, control; TI, total ischemia; IC, intermittent clamping; IPC, ischemic preconditioning. The C group underwent sham operations (laparotomy with exposure of the liver but without vascular occlusion), the TI group underwent continuous clamping with 90 minutes of ischemia, the IC group underwent six cycles of intermittent clamping with 15 minutes of ischemia and 5 minutes of reperfusion, and the IPC group underwent clamping with 90 minutes of ischemia after IPC (15 minutes of clamping followed by 5 minutes reperfusion).
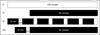
Fig. 2
Hematoxylin-eosin staining of resected livers (×40 or ×400 magnification). The degree of liver damage was determined at ×400 magnification by using a point-counting method as follows: grade 0, minimal or no evidence of injury; grade 1, mild injury consisting of cytoplasm vacuolation and focal nuclear pyknosis; grade 2, moderate to severe injury with extensive nuclear pyknosis, cytoplasmichypereosinophilia, and loss of intercellular borders; grade 3, severe necrosis with disintegration of hepatic cords, hemorrhage, and neutrophil infiltration. The C group had minimal or no evidence of injury (grade 0). All three treatment groups exhibited grade 1 liver damage. C, control; TI, total ischemia; IC, intermittent clamping; IPC, ischemic preconditioning.
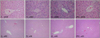
Fig. 3
Terminal deoxyuridine triphosphate nick end labeling (TUNEL) staining (×40 or ×400 magnification). The TI group had significantly more TUNEL-positive hepatocytes than the C and IC groups. There were no significant differences in TUNEL-positive hepatocytes among the C, IC, and IPC groups. C, control; TI, total ischemia; IC, intermittent clamping; IPC, ischemic preconditioning.
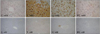
Fig. 4
Number of terminal deoxyuridine triphosphate nick end labeling (TUNEL)-positive cells. The number of TUNEL-positive cells was significantly higher in the experimental groups than in the C group. Among the experimental groups, the total ischemia (TI) group had significantly more positive hepatocytes than the other two groups. IC, intermittent clamping; IPC, ischemic preconditioning. *Statistical significance was tested using Kruskal-Wallis test of variance among groups. The comparison between the groups was performed using Tukey's post hoc test.
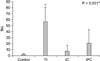
Fig. 5
Hierarchical clustering of the intermittent clamping (IC), ischemic preconditioning (IPC), and total ischemia (TI) groups. The hierarchical clustering of the IC, IPC, and TI group reveals different genetic expression patterns among the groups regarding immediate gene transcription in the livers of rats.
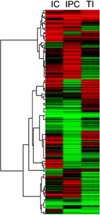
References
1. Murry CE, Jennings RB, Reimer KA. Preconditioning with ischemia: a delay of lethal cell injury in ischemic myocardium. Circulation. 1986. 74:1124–1136.
2. Petrowsky H, McCormack L, Trujillo M, Selzner M, Jochum W, Clavien PA. A prospective, randomized, controlled trial comparing intermittent portal triad clamping versus ischemic preconditioning with continuous clamping for major liver resection. Ann Surg. 2006. 244:921–928.
3. Clavien PA, Selzner M, Rudiger HA, Graf R, Kadry Z, Rousson V, et al. A prospective randomized study in 100 consecutive patients undergoing major liver resection with versus without ischemic preconditioning. Ann Surg. 2003. 238:843–850.
4. Fernandez L, Carrasco-Chaumel E, Serafín A, Xaus C, Grande L, Rimola A, et al. Is ischemic preconditioning a useful strategy in steatotic liver transplantation? Am J Transplant. 2004. 4:888–899.
5. Hong F, Radaeva S, Pan HN, Tian Z, Veech R, Gao B. Interleukin 6 alleviates hepatic steatosis and ischemia/reperfusion injury in mice with fatty liver disease. Hepatology. 2004. 40:933–941.
6. Koti RS, Yang W, Glantzounis G, Quaglia A, Davidson BR, Seifalian AM. Effect of ischaemic preconditioning on hepatic oxygenation, microcirculation and function in a rat model of moderate hepatic steatosis. Clin Sci (Lond). 2005. 108:55–63.
7. Selzner N, Selzner M, Jochum W, Clavien PA. Ischemic preconditioning protects the steatotic mouse liver against reperfusion injury: an ATP dependent mechanism. J Hepatol. 2003. 39:55–61.
8. Serafín A, Rosello-Catafau J, Prats N, Xaus C, Gelpi E, Peralta C. Ischemic preconditioning increases the tolerance of Fatty liver to hepatic ischemia-reperfusion injury in the rat. Am J Pathol. 2002. 161:587–601.
9. Vendemiale G, Grattagliano I, Caraceni P, Caraccio G, Domenicali M, Dall'Agata M, et al. Mitochondrial oxidative injury and energy metabolism alteration in rat fatty liver: effect of the nutritional status. Hepatology. 2001. 33:808–815.
10. Barrier A, Olaya N, Chiappini F, Roser F, Scatton O, Artus C, et al. Ischemic preconditioning modulates the expression of several genes, leading to the overproduction of IL-1Ra, iNOS, and Bcl-2 in a human model of liver ischemia-reperfusion. FASEB J. 2005. 19:1617–1626.
11. Navarro-Sabate A, Peralta C, Calvo MN, Manzano A, Massip-Salcedo M, Rosello-Catafau J, et al. Mediators of rat ischemic hepatic preconditioning after cold preservation identified by microarray analysis. Liver Transpl. 2006. 12:1615–1625.
12. Butte A. The use and analysis of microarray data. Nat Rev Drug Discov. 2002. 1:951–960.
13. Bilbao G, Contreras JL, Eckhoff DE, Mikheeva G, Krasnykh V, Douglas JT, et al. Reduction of ischemia-reperfusion injury of the liver by in vivo adenovirus-mediated gene transfer of the antiapoptotic Bcl-2 gene. Ann Surg. 1999. 230:185–193.
14. Gao W, Bentley RC, Madden JF, Clavien PA. Apoptosis of sinusoidal endothelial cells is a critical mechanism of preservation injury in rat liver transplantation. Hepatology. 1998. 27:1652–1660.
15. Gujral JS, Bucci TJ, Farhood A, Jaeschke H. Mechanism of cell death during warm hepatic ischemia-reperfusion in rats: apoptosis or necrosis? Hepatology. 2001. 33:397–405.
16. Jin LM, Liu YX, Zhou L, Xie HY, Feng XW, Li H, et al. Ischemic preconditioning attenuates morphological and biochemical changes in hepatic ischemia/reperfusion in rats. Pathobiology. 2010. 77:136–146.
17. Pan W, Lin J, Le CT. How many replicates of arrays are required to detect gene expression changes in microarray experiments? A mixture model approach. Genome Biol. 2002. 3:research0022.
18. Ishii S, Abe T, Saito T, Tsuchiya T, Kanno H, Miyazawa M, et al. Effects of preconditioning on ischemia/reperfusion injury of hepatocytes determined by immediate early gene transcription. J Hepatobiliary Pancreat Surg. 2001. 8:461–468.
19. Jang JH, Kang KJ, Kang Y, Lee IS, Graf R, Clavien PA. Ischemic preconditioning and intermittent clamping confer protection against ischemic injury in the cirrhotic mouse liver. Liver Transpl. 2008. 14:980–988.
20. Banga NR, Homer-Vanniasinkam S, Graham A, Al-Mukhtar A, White SA, Prasad KR. Ischaemic preconditioning in transplantation and major resection of the liver. Br J Surg. 2005. 92:528–538.
21. Chen W, Qiu JF, Zhang ZQ, Luo HF, Rosello-Catafau J, Wu ZY. Gene expression changes after hypoxic preconditioning in rat hepatocytes. Hepatobiliary Pancreat Dis Int. 2006. 5:416–421.
22. Raza A, Dikdan G, Desai KK, Shareef A, Fernandes H, Aris V, et al. Global gene expression profiles of ischemic preconditioning in deceased donor liver transplantation. Liver Transpl. 2010. 16:588–599.
23. Peralta C, Bartrons R, Riera L, Manzano A, Xaus C, Gelpí E, et al. Hepatic preconditioning preserves energy metabolism during sustained ischemia. Am J Physiol Gastrointest Liver Physiol. 2000. 279:G163–G171.
24. Kim JS, Ohshima S, Pediaditakis P, Lemasters JJ. Nitric oxide protects rat hepatocytes against reperfusion injury mediated by the mitochondrial permeability transition. Hepatology. 2004. 39:1533–1543.
25. Jaeschke H. Reperfusion injury after warm ischemia or cold storage of the liver: role of apoptotic cell death. Transplant Proc. 2002. 34:2656–2658.
26. Goodrich DW. The retinoblastoma tumor-suppressor gene, the exception that proves the rule. Oncogene. 2006. 25:5233–5243.
27. Gabay C, Smith MF, Eidlen D, Arend WP. Interleukin 1 receptor antagonist (IL-1Ra) is an acute-phase protein. J Clin Invest. 1997. 99:2930–2940.
28. Fili S, Karalaki M, Schaller B. Therapeutic implications of osteoprotegerin. Cancer Cell Int. 2009. 9:26.
29. Venuraju SM, Yerramasu A, Corder R, Lahiri A. Osteoprotegerin as a predictor of coronary artery disease and cardiovascular mortality and morbidity. J Am Coll Cardiol. 2010. 55:2049–2061.
30. Jensen JK, Ueland T, Atar D, Gullestad L, Mickley H, Aukrust P, et al. Osteoprotegerin concentrations and prognosis in acute ischaemic stroke. J Intern Med. 2010. 267:410–417.