Abstract
Purpose
Macrophage migration inhibitory factor (MIF) may serve as a general marker for systemic inflammation in septic and nonseptic acute critical illness. Additionally, our previous experiment has demonstrated that immunosuppressant Prostaglandin E2 (PGE2) lowered MIF levels and inhibited T-cells proliferation when compared to control levels. The addition of hypertonic saline (HTS) increased MIF production as compared with PGE2-stimulated T-cells in concordance with restore PGE2-suppressed T-cells proliferation. Generally, HTS has been well known for its anti-inflammatory effect so far. Therefore, the experiments were conducted to evaluate MIF after stimulating lipopolysaccharide (LPS) either in the presence or absence of HTS in monocyte, in response to early phase injury.
Methods
Human acute monocytic leukemic cell line (THP-1) cells were cultured in RPMI media, to a final concentration of 1 × 106 cells/mL. The effect of HTS on LPS-induced MIF was evaluated in monocyte with 1 µg/mL LPS. HTS at 10, 20 or 40 mmol/L above isotonicity was added. MIF concentrations of the supernatant were determined by enzyme-linked immunosorbent assay, and cell lysates were used for Western blots analysis to determine the MIF expression.
In response to tissue injury, multifactorial networks of chemical signal initiate and maintain a host response designed to 'heal' the afflicted tissue. This involves activation and directed migration of leukocyte (neutrophils, monocytes and eosinophil) from the venous system to sites of damage. Inflammation is the first response of the immune system to infection or irritation. Cytokines are regulators of host responses to immune responses, inflammation and trauma. There are two types of cytokines: pro-inflammatory and anti-inflammatory. Thus, inhibitors of the pro-inflammatory cytokines have been considered as a candidate of anti-inflammatory drugs [1].
Recently, it has become apparent that Macrophage migration inhibitory factor (MIF) plays a central role in several immune responses including the modulation of several cytokines and monocyte, neutrophil and T-cell activation [2]. MIF may serve as a general marker for systemic inflammation in both septic and nonseptic acute critical illness [3,4]. This cytokine appears to be a critical regulator of the inflammatory pathways, leading to systemic inflammatory response syndrome (SIRS) and subsequent multiple organ dysfunction syndrome (MODS) [5,6]. Furthermore, MIF was rediscovered as a neuroendocrine peptide released in a hormone-like fashion by the anterior pituitary gland and the adrenal cortex after exposure to endotoxins (such as lipopolysaccharide [LPS]) of Gram-negative bacteria, corticotrophin-releasing hormone, or physiological stress [7]. However, immune-suppressant prostaglandin E2 (PGE2) lowered MIF levels and inhibited T-cells proliferation when compared to control levels [8,9].
Generally, hypertonic saline (HTS) has been known for its anti-inflammatory effect so far [10,11]. In addition, HTS is also known to have anti-immunoparalysis activity, demonstrated in our experiments by restoring PGE2-suppressant MIF on T-cells. Therefore we hypothesize that the homeostasis of humeral mediators by HTS occurs, at least in part, by an MIF mediated mechanism. Therefore, the experiments were conducted to evaluate MIF after stimulating LPS either in the presence or absence of HTS in monocyte, in response to early phase injury.
Human acute monocytic leukemic cell line (THP-1) cells (American Type Culture Collection, Manassas, VA, USA) were maintained in RPMI-1640 (Invitrogen, Carlsbad, CA, USA) supplemented with 10% fetal bovine serum, 2 mM glutamine, 10 mM HEPES, 100 U/mL penicillin/streptomycin at 37℃ in 5% carbon dioxide incubator. Cells were cultured to a density of 5 × 105 cells/mL. Cell viability, as determined by tyropan blue dye exclusion, was >99%. For the protein extracts, the cells were plated at a density of 1 × 106 cells/mL in 6-well flat-bottom culture plates and were stimulated with LPS (1 µg/mL) (Sigma-Aldrich Co., St. Louis, MO, USA) in the presence or absence of HTS at 10 mmol/L (HTS10), 20 mmol/L (HTS20) and 40 mmol/L (HTS40) above isotonicity, simultaneously. This resulted in sodium concentrations of 150 mmol/L, 160 mmol/L, and 180 mmol/L, which was measured by GEM Premier 3000 (Instrumentation Laboratory, Lexington, MA, USA), respectively.
The tetrazolium dye, MTT, is widely used to assess the viability or the metabolic state of the cells [12]. The MTT-colorimetric monocyte mediated cytotoxicity assay, is based on the ability of living cells to reduce MTT into formazan by mitochondrial succinate dehydrogenase in viable cells. THP-1 cells were plated in 96-well flat-bottom tissue culture plates to attain a final concentration of 1 × 106 cells/mL. The effect of HTS on THP-1 cell viability was evaluated in THP-1 cells with 1 µg/mL LPS, a concentration that induces THP-1 cell cytoxicity, in the presence or absence of HTS at 10 mmol/L, 20 mmol/L, and 40 mmol/L above isotonicity. After incubation for 24 hours at 37℃, the resultant THP-1 cell viability was determined by the MTT viability assay (ATCC, Manassas, VA, USA).
After incubation of THP-1 cells (1 × 105 cells/well; 100 µL) in 96-well culture plates for 2 hours or 20 hours, MIF concentration in culture supernatants was measured by sandwich ELISA. Briefly, 2 µg/mL of monoclonal capture antibody (R&D systems Inc., Minneapolis, MN, USA) was added to a 96-well plate and incubated overnight at room temperature. After incubation, the plates were incubated in a blocking solution comprising phosphate-buffered saline (PBS) containing 1% bovine serum albumin (BSA) and 0.05% Tween 20 for 2 hours at room temperature. The test samples and standard recombinant MIF (R&D systems Inc.) were added to the plates, and the plates were incubated for 2 hours or 20 hours at 4℃. The plates were washed four times with PBS containing Tween 20, and after the addition of 200 ng/mL of biotinylated detection monoclonal antibodies (R&D systems Inc.), plates were incubated for 2 hours at room temperature. The plates were washed, streptavidin-alkaline-phosphatase (1:2000; Sigma-Aldrich Co.) was added, and the reaction was allowed to proceed for 2 hours at room temperature. The plates were washed four times, and 1 mg/mL of p-nitrophenylphosphate dissolved in diethanolamine (Sigma-Aldrich Co.) was added to induce color reaction, which was stopped by adding 50 µL of 1 N NaOH. The optical density at 405 nm was measured on an automated microplate reader (Bio-Rad Laboratories Inc., Hercules, CA, USA). A standard curve was drawn by plotting optical density versus the log of the concentration of MIF.
After incubation for 24 hours at 37℃, the cells were washed 2 times in cold PBS and then centrifuged for 10 minutes. Cells pellet were resuspended in 10 µL per 1 × 106 cells/mL of superlysis buffer (protease Inhibitors, 1 M HEPES, 5 M NaCl, 0.5 M ethylenediaminetetraacetic acid, 1 mM NaOV4, 20% Triton X-100, 50 mM phenylmethylsulfonyl fluoride), incubated on ice for 7 minutes, and then centrifuged at 3,000 × g (12,000 rpm) for 15 minutes at 4℃. The supernatant was then transferred to an eppendorf tube and used for assay. The total protein concentration was determined by the Bradford method using a commercially available assay kit (Thermo Fisher Scientific, Rockford, IL, USA) [13]. The prepared protein lysates were aliquoted and used for Western blot analysis.
By using the protein extracts, expression of MIF protein was quantified by Western blot analysis. Proteins (20 µg/sample) were fractionated on 15% sodium dodecyl sulfate polyacrylamide gel (Bio-Rad Laboratories Inc.) and transferred onto a nitrocellulose membrane. Membranes were blocked for 1h in 5% BSA (Sigma-Aldrich Co.) as the blocking solution and then incubated with anti-human MIF (1:250; R&D systems Inc.). After washing, membranes were incubated with 1:2000 horseradish peroxidase-labeled goat anti-mouse antibody (R&D systems Inc.). The proteins were detected using Supersignal reagent (Pierce, Pockford, IL, USA) chemiluminescence kit.
One-way analysis of variance was performed to evaluate the significance of difference between the experimental groups. For a single comparison of two groups, Student's t-test was used with SPSS ver. 12.0 (SPSS Inc., Chicago, IL, USA). Data are expressed as mean ± SD. A value of P < 0.05 was considered significant. All experiments were performed in triplicate.
We performed the MTT test to evaluate the safety of HTS in THP-1 cells. Cells were exposed to increasing concentrations (10 to 40 mM of culture medium) of HTS for 24 hours. No sign of any negative effect was observed after treatment with HTS. However, THP-1 cell numbers were affected after 24 hours of treatment with LPS 1 µg/mL in the presence or absence of HTS at 10 mM (81.4 ± 0.14% vs. 78.5 ± 0.14%). HTS20 (88.4 ± 0.15%) and HTS40 (87.6 ± 0.13%) were found to protect against LPS-induced cytotoxicity in THP-1 cells (Fig. 1).
To find the relation between HTS and MIF on LPS-induced THP-1 cells, the MIF levels were measured in the cell supernatant. MIF levels increased by 0.69 ± 0.05 ng/mL in the supernatant of LPS-stimulated cells when compared to control levels (0.05 ± 0.01 ng/mL) at 2 hours. Also, MIF levels in LPS-stimulated cells (1.19 ± 0.65 ng/mL) were higher than control cells (0.43 ± 0.51 ng/mL) at 24 hours. However, HTS10 restored partially and decreased the MIF level (0.49 ± 0.05 ng/mL) in LPS-stimulated THP-1 cells (P < 0.05). LPS-stimulated THP-1 cells treated with HTS40 had a lower MIF level (0.27 ± 0.04 ng/mL) than HTS10-treated cells. However, there was no statistically significant difference of MIF levels in the supernatant of HTS10, HTS20 or HTS40 treated cells, respectively, and in the measured times (Fig. 2).
To determine the effect of HTS on the MIF expression, western blot analysis was performed. Correlating with the ELISA, levels of MIF protein expression were higher in LPS stimulating cells (25% decrease in band density) (P < 0.05). The addition of HTS to LPS stimulated cell decreased MIF protein expression regardless of HTS concentrations (Fig. 3). However, there was no statistically significant difference of MIF levels in the supernatant of HTS10 or HTS20 treated cells.
Severe damage on the human body, incurred by trauma, surgery, or prolonged shock may directly induce organ dysfunction. Possible pathogenesis follows: induction of ischemia, reperfusion injury, or immediate tissue destruction due to trauma (first hit). The first hit may not induce a primary multi-system organ failure, but a second hit, such as infection, could further activate the immune system already primed by the first hit. The pathophysiology of sepsis and shock emphasizes the importance of humeral mediator network. Mortality and morbidity will increase with the unusual response of these mediators. Specially, in the early stage after the first hit, increased tumor necrosis factor-α and interleukin (IL) 1 cause a hyperinflammation state, leading to early MODS [1,14,15]. Later on, immunosuppressive mediators such as PGE2, transforming growth factor-beta, IL-10 cause immuneparalysis, resulting in late MODS [1]. The mechanism through which hypertonic saline solutions reduce mortality in hypotensive patients is still unclear [16]. However, recent studies have demonstrated that hypertonicity substantially alters activation of inflammatory cells, consequently reducing subsequent organ injury from ischemia-reperfusion and decreasing nosocomial infection [17-19].
By controlling immune and inflammatory responses, MIF is thought to play an important role in the pathophysiology of septic shock and chronic inflammatory diseases. This implies that MIF may serve as a general marker for systemic inflammation in septic and nonseptic acute critical illness [3,4]. MIF is, specifically, a cytokine secreted by the anterior pituitary gland and immune cells in response to surgical stress, injury, and sepsis [7]. This cytokine appears to play a critical role in the regulation of the inflammatory pathways, contributing to SIRS and subsequent MODS [5,6]. MIF is also secreted by activated T-cells, and neutralizing anti-MIF antibodies inhibit T-cell proliferation and IL-2 production. T-cells also release MIF in response to glucocorticoid stimulation. MIF, in this case, acts to override glucocorticoid inhibition of T-cell proliferation, IL-2 and interferon γ production [8]. As for contribution of MIF to the regulation of cell survival, the inhibitory effect of MIF on apoptosis may be related to its reported stimulatory effects on cell proliferation, even though cellular apoptosis and cell proliferation are not simply the reverse of each other [6,20-22]. Our previous experiments have shown that PGE2 inhibited T-cells proliferation, leading to a decrease in MIF levels when compared to control levels. The addition of HTS increased MIF production as compared with PGE2-stimulated T-cells in concordance with restore PGE2-suppressed T-cells proliferation (not shown). The role of HTS in restoring T-cell proliferation suppressed by PGE2, at least in part, is believed to be mediated through an MIF pathway. Hyperosmolality may play an important role in HTS-enhancement of T-cell proliferation. Hypertonicity may contribute to increased IL-2 expression for T-cell proliferation [9,11,23].
LPS, a component of the cell wall of Gram-negative bacteria, is an endotoxin that induces septic shock syndrome. It stimulates the production of inflammatory mediators and is known to activate a number of cellular signals of human monocyte cells during inflammation and infection. Monocytes are key mediators of inflammation and widely distributed in the body [24,25]. THP-1 cells, which represent an appropriate model system to study immune responses, were utilized, therefore, to investigate the anti-inflammatory effects of HTS. Our present study demonstrated that HTS is an effective inhibitor of LPS-induced MIF generation and expression in THP-1 cells. In order words, LPS increased MIF levels in THP-1 cells. However, HTS restored the MIF levels in LPS-stimulated THP-1 cells and there was no statistically significance difference of MIF levels in HTS10, HTS20 or HTS40 treated cells. Besides, similar to ELISA, there was no statistically significance difference in MIF levels in HTS10 or HTS20 treated cells. So, it is considered that HTS10 would be sufficient. It is presumed that HTS controls LPS-induced MIF production or secretion. Therefore, we need to study evaluations between Toll-like receptor 4 or MIF receptors, which effect MIF production and effect, and HTS. Finally, this study indicated that HTS exhibited anti-inflammatory activities in THP-1 cells.
MIF exhibits proinflammatory activity and regulates cell proliferation and survival. In our previous and present experiment, MIF production was lower in PGE2-stimulated T-cells, and higher in LPS-stimulated THP-1 cells compared to control groups. HTS restored MIF production to control in PGE2-stimulated T-cells and LPS-stimulated THP-1 cells. We have demonstrated that HTS acts to maintain balance between both pro- and anti-inflammatory activities, which is critical for host defense against diseases. This implies that the role of HTS in maintaining homeostasis with humeral mediators, at least in part, should be mediated through an MIF pathway. This in vitro study, however, does not necessarily indicate respective in vivo effects, especially since the concentrations used are far higher than are achievable in vivo. Still, it may contribute to a better understanding of the possible biological actions of HTS.
Figures and Tables
Fig. 1
3-(4,5-dimethylthiazol-2-yl)-2,5-diphenyl-tetrazolium bromide (MTT) assay in THP-1 cells after 24 hours treatment with lipopolysaccharide (LPS) and varying concentrations of hypertonic saline (HTS). Absorbance of MTT formazan was determined at 570 nm using enzyme-linked immunosorbent assay reader. *LPS induced THP-1 cell viability by 19.3% (P < 0.05). However, HTS alone has no cytotoxic effects in THP-1 cells. Besides, †HTS partially protects against LPS-induced cytotoxicity in THP-1 cells. There was no statistical difference in protective effect by either HTS20 or HTS40.
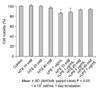
Fig. 2
Migration inhibitory factor (MIF) levels in cell supernatant were measured. THP-1 cells in presence of lipopolysaccharide (LPS) had increase in MIF level (0.69 ng/mL ± 0.05) when compared with THP-1 cells (0.05 ng/mL ± 0.01) at 2 hours. However, hypertonic saline (HTS) 10 restored partially and decreased MIF levels (0.49 ng/mL ± 0.05) when compared with LPS-stimulated THP-1 cells (P < 0.05). LPS-stimulated THP-1 cells treated with HTS40 had lower MIF levels (0.27 ng/mL ± 0.04) than HTS10-treated cells, however, there was no statistically significant difference on MIF levels according to HTS concentrations or measured times. ELISA, enzyme-linked immunosorbent assay.
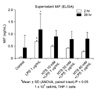
ACKNOWLEDGEMENTS
We are grateful to our colleagues for participation in the studies and their helpful discussion: Park JH, Han KS. This research was supported by Basic Science Research Program through the National Research Foundation of Korea (NRF) funded by the Ministry of Education, Science and Technology (R1009982) and was partially supported by a Korea University Grant.
References
1. Smith JW, Gamelli RL, Jones SB, Shankar R. Immunologic responses to critical injury and sepsis. J Intensive Care Med. 2006. 21:160–172.
2. Lehmann LE, Weber SU, Fuchs D, Klaschik S, Schewe JC, Book M, et al. Intracellular detection of macrophage migration inhibitory factor in peripheral blood leukocytes. Free Radic Biol Med. 2005. 38:1170–1179.
3. Bernhagen J, Calandra T, Bucala R. Regulation of the immune response by macrophage migration inhibitory factor: biological and structural features. J Mol Med (Berl). 1998. 76:151–161.
4. Lehmann LE, Novender U, Schroeder S, Pietsch T, von Spiegel T, Putensen C, et al. Plasma levels of macrophage migration inhibitory factor are elevated in patients with severe sepsis. Intensive Care Med. 2001. 27:1412–1415.
5. Larson DF, Horak K. Macrophage migration inhibitory factor: controller of systemic inflammation. Crit Care. 2006. 10:138.
6. Lue H, Kleemann R, Calandra T, Roger T, Bernhagen J. Macrophage migration inhibitory factor (MIF): mechanisms of action and role in disease. Microbes Infect. 2002. 4:449–460.
7. Schmidt-Supprian M, Murphy C, While B, Lawler M, Kapurniotu A, Voelter W, et al. Activated protein C inhibits tumor necrosis factor and macrophage migration inhibitory factor production in monocytes. Eur Cytokine Netw. 2000. 11:407–413.
8. Bacher M, Metz CN, Calandra T, Mayer K, Chesney J, Lohoff M, et al. An essential regulatory role for macrophage migration inhibitory factor in T-cell activation. Proc Natl Acad Sci U S A. 1996. 93:7849–7854.
9. Choi SH, Bansal V, Costantini T, Putnam J, Loomis W, Coimbra R. Arginine is essential in reversing prostaglandin E(2) T-cell suppression by hypertonic saline. J Surg Res. 2009. 156:83–89.
10. Coimbra R, Junger WG, Liu FC, Loomis WH, Hoyt DB. Hypertonic/hyperoncotic fluids reverse prostaglandin E2 (PGE2)-induced T-cell suppression. Shock. 1995. 4:45–49.
11. Junger WG, Liu FC, Loomis WH, Hoyt DB. Hypertonic saline enhances cellular immune function. Circ Shock. 1994. 42:190–196.
12. Saad B, Dakwar S, Said O, Abu-Hijleh G, Al Battah F, Kmeel A, et al. Evaluation of medicinal plant hepatotoxicity in co-cultures of hepatocytes and monocytes. Evid Based Complement Alternat Med. 2006. 3:93–98.
13. Bradford MM. A rapid and sensitive method for the quantitation of microgram quantities of protein utilizing the principle of protein-dye binding. Anal Biochem. 1976. 72:248–254.
14. Coussens LM, Werb Z. Inflammation and cancer. Nature. 2002. 420:860–867.
15. Delgado AV, McManus AT, Chambers JP. Production of tumor necrosis factor-alpha, interleukin 1-beta, interleukin 2, and interleukin 6 by rat leukocyte subpopulations after exposure to substance P. Neuropeptides. 2003. 37:355–361.
16. Bulger EM, May S, Kerby JD, Emerson S, Stiell IG, Schreiber MA, et al. Out-of-hospital hypertonic resuscitation after traumatic hypovolemic shock: a randomized, placebo controlled trial. Ann Surg. 2011. 253:431–441.
17. Costantini TW, Deree J, Martins JO, Putnam JG, de Campos T, Coimbra R. A novel fluid resuscitation strategy modulates pulmonary transcription factor activation in a murine model of hemorrhagic shock. Clinics (Sao Paulo). 2010. 65:621–628.
18. Choi SH, Lee SW, Hong YS, Jeun JM, Min BW. Selective inhibition of polymorphonuclear neutrophils by resuscitative concentration of hypertonic saline. Emerg Med J. 2006. 23:119–122.
19. Coimbra R, Hoyt DB, Junger WG, Angle N, Wolf P, Loomis W, et al. Hypertonic saline resuscitation decreases susceptibility to sepsis after hemorrhagic shock. J Trauma. 1997. 42:602–606.
20. Mitchell RA, Liao H, Chesney J, Fingerle-Rowson G, Baugh J, David J, et al. Macrophage migration inhibitory factor (MIF) sustains macrophage proinflammatory function by inhibiting p53: regulatory role in the innate immune response. Proc Natl Acad Sci U S A. 2002. 99:345–350.
21. Nguyen MT, Lue H, Kleemann R, Thiele M, Tolle G, Finkelmeier D, et al. The cytokine macrophage migration inhibitory factor reduces pro-oxidative stress-induced apoptosis. J Immunol. 2003. 170:3337–3347.
22. Park JK, Kim JK. Influence of MIP-1 Alpha on the CD4+ Th Lymphocytes. J Korean Surg Soc. 2004. 66:81–88.
23. Hoyt DB, Junger WG, Loomis WH, Liu FC. Effects of trauma on immune cell function: impairment of intracellular calcium signaling. Shock. 1994. 2:23–28.
24. Gilroy DW, Lawrence T, Perretti M, Rossi AG. Inflammatory resolution: new opportunities for drug discovery. Nat Rev Drug Discov. 2004. 3:401–416.
25. Chen CF, Cheng CH. Regulation of cellular metabolism and cytokines by the medicinal herb feverfew in the human monocytic THP-1 cells. Evid Based Complement Alternat Med. 2009. 6:91–98.