Abstract
Purpose
Even though adipose tissue-derived stem cells (ADSCs) have been spotlighted as a possible alternative for liver transplantation in an experimental setting, the mechanism by which ADSCs improve liver dysfunction remains poorly characterized. The objective of this study was to evaluate the therapeutic ability of undifferentiated ADSCs, and find a few clues on how ADSCs alleviate liver damage by comparing the transplantation routes.
Methods
In vitro generated human ADSCs were checked for surface markers and stage-specific genes for characterization. Afterwards, they were transplanted into C57BL/6 mice with CCl4-induced liver injury. The transplantations were made via tail vein, portal vein, and direct liver parenchymal injection. At 1 and 3 post-transplantation days, serum biochemical parameters and/or liver specimens were evaluated.
Results
We have shown here that ADSCs have the characteristics of mesenchymal stem cells, and belong to endodermal and/or early hepatic differentiation stage. After transplantation into the mice with acute liver failure, markers of liver injury, such as alanineaminotransferase, aspartateaminotransferase, as well as ammonia, decreased. Of these transplantation routes, transplantation via tail vein rendered the most prominent reduction in the biochemical parameters.
At present, liver transplantation is considered as the best curative option for end-stage liver diseases. However, the limitations of liver transplantation, such as the shortage of organ donors, have made the process long and difficult for peri-transplantation courses. Therefore, the adverse effects caused by life-long immunosuppressive regimens have prompted the efforts to find the alternative. Hepatocyte transplantation was first considered, however, it also faced several difficulties, which include the unsolved problem of donor shortage, troublesome processes of cultivation, and requirement of immunosuppressive regimens. Under these circumstances, many investigators have researched stem cell transplantation with the hope of overcoming these limitations.
Of all the possible stem cell lines, we paid attention to the adult stem cells, especially mesenchymal stem cells (MSCs). MSCs were proven to have the ability to proliferate extensively, and to differentiate into multiple cell types [1,2]. In addition, MSCs are known to circumvent immunocompatibility problems because they have low expressions of human leukocyte antigen (HLA) I and no HLA II, as well as a low risk of allogenic rejection and graft-versus-host disease [3,4]. Various tissues, such as the bone marrow, amniotic fluid, placenta, umbilical cord blood, scalp tissue, placenta, and adipose tissue, were known to contain MSCs [5-9].
In our study, we intended to evaluate the therapeutic potential of the adipose tissue. Zuk et al. [9,10] first demonstrated the adipse tussue-derived stem cells' (ADSCs) potential for a multi-lineage differentiation, and Seo et al. [11] reported the first successful hepatocytic differentiation from ADSCs. Thereafter, numerous trials have applied ADSCs into hepatic failure animal models and demonstrated the therapeutic potentials of ADSCs transplantation [12-17]. However, the exact mechanism by which ADSCs improve the liver dysfunction in a hepatic injured animal model remains to be clarified. Most researchers have focused on the possibility that the transplanted ADSCs become engulfed in the liver, where they act as hepatocyte through transdifferentiation or fusion [11,12,14-17]. Therefore, they have attempted to establish differentiated ADSCs in vitro prior to a transplantation. However, it also has been proposed that MSCs act as trophic mediators, by which they secrete bioactive factors to ameliorate the liver damage [13,18]. To prove this ADSCs' hormonal effect, the therapeutic effect of undifferentiated ADSCs should first be demonstrated. Therefore, the purpose of this study was to evaluate the therapeutic ability of undifferentiated ADSCs, and find a clue on how ADSCs alleviate liver damage by comparing the transplantation routes.
Adipose tissues were obtained from 3 different donors (donor 1: female, 33 years old; donor 2: female, 17 years old; donor 3: male, 13 years old) undergoing appendectomy or cholecystectomy. The hospital's ethics committee approved this study and informed consents were obtained from all the patients. To isolate ADSCs, adipose tissues were washed with equal volumes of phosphate-buffered saline (PBS) and digested at 37℃ for 30 minutes with 0.075% collagenase type I (Sigma-Aldrich Co., St. Louis, MO, USA). Enzyme activity was neutralized with Dulbecco's modified Eagle's medium (DMEM)/F-12 (Invitrogen, Carlsbad, CA, USA) containing 10% fetal bovine serum (FBS; Invitrogen). Adipose tissue cells were filtered through a 100-µm nylon mesh to remove the cellular debris. Cell suspension was centrifuged for 10 minutes to obtain a pellet and cultured at 37℃/5% CO2 in culture media (DMEM/F-12, 10% FBS, and 100 U/mL penicillin/100 µg/mL streptomycin). Following the incubation, the plates were washed with PBS to remove the residual non-adherent red blood cells. The resulting cell population was considered as stromal vascular fraction, and banked in DMEM/F-12 containing 10% FBS. After thawing, cells were cultured at 37℃/5% CO2 in DMEM/F-12 containing 10% FBS culture media. Adherent cells were maintained in culture, and the media were changed every 2 days. Upon reaching an 80% confluence, the cells were digested with 0.25% trypsin/ethylenediaminetetraacetic acid at 37℃, centrifuged and resuspended in the media. The cell suspensions were plated in new flasks and remained in the culture. After 3 passages, the cells were used for analysis.
ADSCs were analyzed by flow cytometry for cell surface antigen expression. Passage-three cells were incubated with fluorescein isothiocyanate- or phycoerythrin-conjugated anti-CD44, anti-CD45, anti-CD29 or mouse immunoglobulin G isotype control antibodies (BD Biosciences, Heidelberg, Germany) for 30 minutes at 4℃ in PBS. Cells were washed and analyzed by a FACSCalibur kit (BD Biosciences).
To determine which developmental stage - endodermal stage, early hepatic differentiation stage, or mature hepatocyte stage - the ADSCs belong to, RT-PCR was performed. In order to make a proper comparison, we attempted an endodermal induction for ADSCs [12,19]. Thereafter, ADSCs without an endodermal induction were compared against ADSCs with an endodermal induction. For an endodermal induction, the cells were treated for 3 days with DMEM/F-12, (serum free) supplemented with 20 ng/mL Activin A (R&D Systems Inc., Minneapolis, MN, USA) and 10 ng/mL FGF4 (PeproTech EC Ltd., London, UK). Total RNA was extracted from ADSCs using a TRIzol kit (Invitrogen). RNA integrity was examined by agarose gel electrophoresis. cDNA was synthesized using 2 mg of total RNA and random primers. GAPDH was used as a reference gene. Specific PCR products (Bioneer, Daejeon, Korea) were generated using 5 pmol sense and antisense primers as indicated below: GATA4: sense, 5'-TTCCTC TTCCCTCCTCAA AT-3' and anti-sense, 5'-TCA GCG TGT AAA GGC ATC TG-3'; FOXA2: sense, 5'-GGGAGCGGTGAAGATGGAAG-3' and anti-sense, 5'-TGCCAGCGCCCACGTA-3'; α-Fetoprotein (AFP): sense, 5'-AACAAGTATGGATTCTCAGG-3' and anti-sense, 5'-ATTGATGCTCTCTTTGTCTG-3'; HNA-4α: sense, 5'-GAGCAGGAATGGGAAGGA TG-3' and anti-sense, 5'-GGCTGTCCTTTGGGATGA AG-3'; and GAPDH: sense, 5'-TCCATGACA ACTTTGGTATC-3' and anti-sense, 5'-TGTAGCCAAATTCGTTGTTA-3', based on human genome database (National Center for Biotechnology Information).
For the identification of ADSCs after the transplantation, ADSCs nuclei were stained using DAPI (Sigma-Aldrich). DAPI was added to the media to a final concentration of 50 mg/mL. The cells were incubated at 37℃ under 5% CO2 for 30 minutes, then washed six times in PBS. Cells were detached by digestion with 0.25% trypsin/EDTA at 37℃, centrifuged, and resuspended in PBS at 2 × 108 cells/mL.
Animal studies were carried out in compliance with the guidelines of the Institute for Laboratory Animal Research, Korea. Eight-week-old male C57BL/6 mice (Damool Science, Daejeon, Korea) were used. Experimental mice were largely divided into 2 groups: control group (saline infusion group; SL group = 5) and transplantation group (TR group, n = 15). TR group was further divided according to the transplantation routes; transplantation via tail vein (TV; n = 5), transplantation via portal vein (PV; n = 5), and transplantation via direct liver parenchyma infusion (LP; n = 5) groups (Fig. 1). Acute liver failure model was produced by giving an i.p. injection of 100 mL/20 g body-weight of olive oil containing 10 mL CCl4 at day 0. The control group received i.p. injection of 100 mL/20 g body-weight of olive oil without CCl4. At day 1, mice underwent a transplantation of ADSCs at a concentration of 1.5 × 106 cells per mouse. The transplantation routes were different according to each TR group. Specifically, in the PV group, the transplantation was performed after the midline laparotomy under i.v. ketamine anesthesia. Spleen was exposed using a wet cotton swab. A purse-string suture using a prolene 6-0 (Ethicon Inc., Somerville, NJ, USA) was made around a presumed infusion site. Then, ADSCs were infused slowly for 1 minute, and thereafter, the purse-string suturing was completed. In the LP group, the same laparotomy as the PV group was made. After putting a superior lobe aside, the infusion was solely performed into a medial lobe after the placement of purse-string suture. After the infusion, purse-string suturing was completed. Lastly, in TV the group, transplantation was made via tail vein without anesthesia.
Blood samples were obtained from each mouse at 1 day and 3 days after the transplantation, centrifuged for 20 minutes at 400 g, and its serum was collected. The concentration of biochemical parameters such as aspartate aminotransferase (AST), alanine aminotransferase (ALT), and ammonia were analyzed by using a IDEXX VetTest Chemistry Analyzer (IDEXX Laboratories Inc., Westbrook, ME, USA).
HE staining was performed to find the changes in histological architecture, and Ki67 stain was followed to evaluate the degree of proliferation. Liver specimens were prepared at Day 1 post-transplantation, and each specimen was stained into hematoxylin-esosin stain and Ki 67 stain, respectively. For Ki67 immunohistochemistry, paraffin-embedded tissue sections were deparaffinized in xylene and rehydrated in a graded series of alcohol. The antigen was retrieved with 0.01M citrate buffer (pH 6.0) by heating the sample in autoclave (CHS-ACCE-860, JW Pharmaceutical, Seoul, Korea) at a controlled final temperature of 121℃ for 25 minutes. The tissue sections were then placed in 3% hydrogen peroxide for 5 minutes to inactivate the endogenous peroxidase, and blocked for 10 minutes with normal horse serum (DakoCytomation LSAB2 System-HRP kit; DakoCytomation, Glostrup, Denmark). The primary antibody used for this study was Ki-67 mouse monoclonal antibody (Clon MIB-1, 1:100; DakoCytomation). All the pre-diluted primary antibodies were applied for 30 minutes at room temperature. The slides were then treated with biotinylated secondary antibody for 10 minutes at room temperature, followed by Streptavidin-HRP and 3,3'-diaminobenzidine solution for another 10 minutes at room temperature. Tissue sections were counter-stained with hematoxylin.
ADSCs were cultivated in DMEM/F-12 containing 10℃ FBS, and were replated when they reached an 80℃ confluence. ADSCs initially appeared as a spindle shape, reminding of fibroblasts (Fig. 2A). After the 3-day endodermal Activin A and FGF4 induction period, this fibroblast-like morphology was progressively changed into an oval shape (Fig. 2B).
To specify the antigenic properties of ADSCs, cell surface protein expression was determined by flow cytometry. Flow cytometric analysis confirmed the expressions of the MSC markers (CD29, CD105, and CD166) in all three donors, however not the hematopoietic stem cell marker (CD34) (Fig. 3).
Gene expression of undifferentiated ADSCs was compared with the gene expression of ADSCs which had passed an endodermal induction. In both groups, genes related with endodermal stage (GATA4) and early hepatic differentiation (FOXA2, AFP) were expressed. However, genes related with mature hepatocyte (Albumin, HNF-4α) were not (Fig. 4).
ADSCs labeled by DAPI had round or oval light blue spots when viewed under the fluorescence microscope, and most ADSCs appeared to be labeled. We transplanted 1.5 × 106 cells of DAPI-labeled ADSCs into the CCl4-injured mice by 3 different ways; via tail vein (TV group), portal vein (PV group), and directly liver parenchyma (LP group). Liver specimens were obtained 24 hours after the transplantation to certify the distribution of DAPI-positive cells in each group. In the TV group, DAPI-positive cells were found to be evenly distributed under the fluorescence microscope (Fig. 5A). In the PV group, DAPI-positive cells were observed in clusters, scattered throughout every lobe of the liver (Fig. 5B). In the LP group, DAPI-positive cells were mainly restricted in the injected lobe, forming a cluster nature (Fig. 5C). To estimate the amount of engulfed ADSCs according to each transplantation route, the number of DAPI-positive cells was counted in 5 randomized views of the liver sections. The mean numbers of DAPI-positive cells in the TV, PV, and LP group were 48.0 ± 5.7, 43.4 ± 3.1, and 38.2 ± 5.5, respectively (Fig. 5D). The number of DAPI-positive cells in the TV group was comparable to the number in the PV group. However, the LP group showed the least amount of prominent ADSCs engulfment among these groups (P = 0.026).
HE staining was performed to find the changes in histological architecture, and Ki67 stain was followed to evaluate the degree of proliferation. Normal mouse liver showed well-organized hepatic cords in a HE stain, and unstained nuclei in a Ki67 stain suggested a low proliferation status (control group). Twenty-four hours after the CCl4 injection, centrilobular necrosis was observed in a HE stain (HF group). Additionally, no nucleus was stained with Ki67 stain in the HF group. Histological stains were obtained 24 hours after the transplantation. In the TV group, reduction of the necrosis area was observed when it was compared to the SL group, and several nuclei stained with Ki67 emerged. Such an improvement - the reduction of the necrosis area, and the emergence of Ki67 stained nuclei- was also observed in the TV and LP group. The degree of improvement was most prominent in the TV group, followed by the PV group, the LP group, respectively (Fig. 6).
Twenty-four hours after the CCl4 i.p. injection, biochemical parameters such as AST, ALT, and ammonia were significantly increased in the HF group, when it was compared to the control group. Next, we evaluated the therapeutic potential according to the transplantation routes (Fig. 7). One day after the ADSCs transplantation, AST and ALT values of the TV group and PV group were significantly lower compared to the measurements in the SL group (in AST values, 760 IU/L [980 - 640], 1050 IU/L [1,320 - 980] versus 1,585 IU/L [1,890 - 1,110], P < 0.05; in ALT values, 620 IU/L [1,300 - 360], 1,000 IU/L [1,100 - 800] versus 1,200 [2,470 - 850], P < 0.05) (Fig. 7). And, 3 days after the transplantation, AST and ALT of the all TR groups (TV, PV, and LP group) were significantly lower than the values of SL group (in AST values, 260 IU/L [280 - 185], 370 IU/L [560 - 110], and 570 IU/L [740 - 320] versus 980 IU/L [1,230 - 450], P < 0.05). Of the TR groups, one day after transplantation, the TV group showed the most prominent reduction in the biochemical parameters than the PV and LP groups (in AST values, 760 IU/L [980 - 640] versus 1050 IU/L [1,320 - 980], 1,320 IU/L [1,530 - 1,120], P < 0.05; in ALT values, 620 IU/L [1,300 - 360] versus 1,000 [1,100 - 800], 1,020 [1,360 - 820], P < 0.05). Likewise, ammonia concentrations of the TR groups were lower than the concentrations of the SL group, though this difference did not reach a statistical significance.
The shortage of organ donors and other substantial limitations of liver transplantation have caused many researchers to find an alternative option. Ever since the pluripotency and reproducibility of the stem cells have been disclosed [20], many investigators have hoped to alleviate liver diseases by a stem cell transplantation. Therefore, many experiments have been carried out. However, there's still a long way to go since there is still no consensus on the stem cell source, the transplantation route, or even the appropriate amount of the stem cells. Of all the available stem cell sources, MSCs have been spotlighted, possibly due to its affluence and easy approachability [21]. We paid our attention to the adipose tissue as a possible MSC source for following reasons. First, extra procedure to attain the tissue is usually not required because adipose tissues can easily be harvested in the process of a common surgical procedure, such as appendectomy and cholecystectomy. Furthermore, we could also utilize the omental patch which is removed during the abdominal cancer operations. Next, the stem cell yield from the adipose tissue is known to be, maximally, about 40 times more than the yield from the bone marrow [22,23]. Lastly, in-vitro expansion of ADSC can be performed conveniently.
Over the past two decades after the discovery of stem cells in the adipose tissue [9], the therapeutic potential of ADSCs to the liver disease have been explored. However, numerous reports focused on the hepatic differentiation of ADSCs, because they thought that the ADSCs' therapeutic potential originated from the functionally differentiated stem cells. However, it was also known that stem cells can act as paracrine or endocrine cells to affect adjacent cells by secreting bioactive factors. Rehman et al. [18] reported that adipose stromal cells, which had been obtained from human subcutaneous adipose tissue, secreted considerable amount of vascular endothelial growth factor, hepatocyte growth factor, and transforming growth factor-β. Moreover, Banas et al. [13] later observed that ADSCs secreted a higher volume of interleukin 1 receptor α (IL-1Rα), IL-6, IL-8, granulocyte colony-stimulating factor (G-CSF), granulocyte-macrophage (GM-CSF), monocyte chemotactic protein 1, and nerve growth factor than MSCs originated from either the bone marrow or human dermal fibroblasts did. In our study, we demonstrated a definite decline in the liver enzyme levels after an undifferentiated ADSC transplantation. The changes were observed one day after the transplantation, and became prominent 3 days after the transplantation. Because a 24-hour period is not sufficient for ADSCs to differentiate into full-grown hepatocytes, it is natural to think that the systemic changes induced by ADSCs might have contributed to it. Our observation was also in line with other reports [13,16]. We think that our result provides a substantial meaning when it is applied to a clinical setting. Acute liver failure is a catastrophe which can lead to death. Moreover, there is a very limited time window to treat and save the patients. However, inducing a hepatic differentiation needs fixed time, ranging from 2 weeks to several months [11,12,14-17]. Therefore, it is our opinion that if the undifferentiated ADSCs' effectiveness can be proved in a clinical setting, a number of patients can receive a potentially life-saving treatment within that small window of time.
In this experiment, the therapeutic potentials according to the transplantation route were compared. Until now, in most studies, ADSC transplantations have been attempted by a peripheral vein [11-14,17], and uncommonly, via portal vein [15] and direct liver parenchyma [16]. We think that a study of the transplantation route can give essential information about how ADSCs influence the injured liver. After a liver injury, a systemic change occurs [24-29]; the SDF-1, G-CSF, and other mediator expressions increase in the peripheral blood, whereas the SDF-1 and CXCR4 expressions in the bone marrow decrease because of neutrophil protease. These changes lead to the recruitment of MSCs and hematopoietic stem cells into the peripheral blood, ending up reaching the liver. During this process, the hepatic damage is thought to be ameliorated by the ADSCs' endocrine and/or paracrine effects. In our study, whereas transplanted ADSCs via tail vein eventually reach the liver by way of the blood stream, transplantations via other routes - portal vein and direct liver parenchyma - bypass the systemic circulation. Therefore, bypassing the systemic circulation in PV group and LP group is thought to be the cause of lesser prominent liver repairing effects in these groups.
We found several differences according to the transplantation route. First, there was a noticeable difference in the liver's ADSCs distribution; whereas the TV group showed evenly scattered ADSCs in the liver, the PV group and the LP group demonstrated clustered ADSCs alongside the portal vein or the injected site, respectively. Next, we observed that the engulfed amount of tail-vein transplanted ADSCs was not lesser than the engulfed amount of portal vein transplanted ADSCs, even though tail-vein transplanted ADSCs reached the liver by way of the systemic circulation. One explanation could be that the chemotactic factors, which had been released from the injured liver, effectively attracted the ADSCs [27,30]. Lastly, of these three different transplantation route groups, the TV group showed the most prominent reduction in the biochemical parameters. Therefore, we think the bioactive factors in the TV group might have contributed to this phenomenon.
In conclusion, we demonstrated that undifferentiated ADSCs were able to improve the hepatic function in mice with acute liver injury within a short span of time. Since there was not a sufficient time period for the ADSCs to differentiate into full-grown hepatocytes, it is natural to think that the ADSCs induced hormonal changes favorable to the liver function. We also observed that the transplantation via peripheral vein resulted in a more prominent liver function than the transplantation via other routes. This finding also supports the ADSCs' hormonal effect because the ADSCs transplanted via peripheral vein had relatively longer time to stay in the systemic circulation than the ADSCs transplantated via other routes. However, for the clinical application, further and more extensive studies are necessary to confirm these findings as well as to clarify exactly how ADSCs improve the liver function.
Figures and Tables
Fig. 1
Study design. SL, saline infusion via tail vein; PV, transplantation via portal vein; TV, transplantation via tail vein; LP, transplantation via direct liver parenchyma infusion.
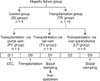
Fig. 2
Morphology of adipose tissue-derived stem cells (ADSCs) before (A) and after (B) endodermal induction (×100 magnification). ADSCs initially had spindle-like appearance progressively changing into an oval shape after endodermal induction.
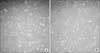
Fig. 3
Flow cytometry of adipose tissue-derived stem cells (ADSCs) with mesenchymal and hematopoietic stem cell markers. Mesenchymal stem cell markers (CD29, CD105, and CD166) were expressed whereas hematopoietic stem cell marker (CD34) was not.
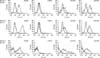
Fig. 4
Genes related to hepatic differentiation determined by reverse transcription-polymerase chain reaction. Adipose-tissue derived stem cells (ADSCs), both before and after the endodermal induction, expressed GATA4, FOXA2, and AFP genes, and did not express albumin and HNF-4α genes.
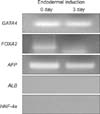
Fig. 5
Engulfment of 4, 6-diamidino-2-phenylindole (DAPI)-labeled adipose tissue-derived stem cells (ADSCs) in mouse liver according to different transplantation routes. Mice with acute liver injury were transplanted with DAPI-labeled ADSCs injected through tail vein (TV group) (A), portal vein (PV group) (B), and direct liver parenchyma (LP group) (C). Panels show fluorescent microscopic views of representative liver sections obtained one day after the transplantation (×100 magnification). The number of DAPI-positive cells of the TV group was comparable to the number of the PV group (D). However, the number of DAPI- positive cells of the TV group was significantly higher than that of the LP group (P = 0.026).a)
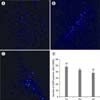
Fig. 6
Comparison of histological changes according to transplantation routes. The histological sections were stained into two ways; H&E stain (A-D) and Ki67 immunohistochemical stain (E-H). Panel (A-D) represents the changes in the centrilobular necrosis area (red ellipse) observed after the saline infusion via tail vein (A), the transplantion of ADSCs via tail vein (B), portal vein (C), and direct liver parenchyma infusion (D). Panel (E-H) represents the changes in the occurrence of Ki67-positive nuclei (arrow; brown spot) observed after the saline infusion via tail vein (A), the transplantion of ADSCs via tail vein (B), portal vein (C), and direct liver parenchyma infusion (D).
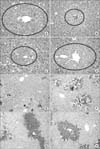
Fig. 7
Comparison of biochemical parameters including aspartate aminotransferase (AST) (A), alanine aminotransferase (ALT) (B), and ammonia (C) according to transplantation routes. The means and standard deviations were demonstrated. Of the 3 different transplantation route groups, the TV group (adipose tissue-derived stem cells transplantation via tail vein) showed the most prominent reduction in the AST and ALT values (P<0.05). SL, saline infusion group; PV, transplantation via portal vein; TV, transplantation via tail vein; LP, transplantation via liver parenchyma.
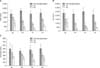
ACKNOWLEDGEMENTS
This work was supported by foundations donated by Astellas Pharma Korea Inc. (5-2006-D0237-00004), by Gangneung Dong-In Hospital, and by Clinical Research Institute, Daejeon St. Mary's Hospital, The Catholic University of Korea School of Medicine (CMCDJ-P-2011-001).
References
1. Thomson JA, Itskovitz-Eldor J, Shapiro SS, Waknitz MA, Swiergiel JJ, Marshall VS, et al. Embryonic stem cell lines derived from human blastocysts. Science. 1998. 282:1145–1147.
2. Pittenger MF, Mackay AM, Beck SC, Jaiswal RK, Douglas R, Mosca JD, et al. Multilineage potential of adult human mesenchymal stem cells. Science. 1999. 284:143–147.
3. McIntosh K, Zvonic S, Garrett S, Mitchell JB, Floyd ZE, Hammill L, et al. The immunogenicity of human adipose-derived cells: temporal changes in vitro. Stem Cells. 2006. 24:1246–1253.
4. Bartholomew A, Sturgeon C, Siatskas M, Ferrer K, McIntosh K, Patil S, et al. Mesenchymal stem cells suppress lymphocyte proliferation in vitro and prolong skin graft survival in vivo. Exp Hematol. 2002. 30:42–48.
5. Conget PA, Minguell JJ. Phenotypical and functional properties of human bone marrow mesenchymal progenitor cells. J Cell Physiol. 1999. 181:67–73.
6. De Coppi P, Bartsch G Jr, Siddiqui MM, Xu T, Santos CC, Perin L, et al. Isolation of amniotic stem cell lines with potential for therapy. Nat Biotechnol. 2007. 25:100–106.
7. Bieback K, Kern S, Klüter H, Eichler H. Critical parameters for the isolation of mesenchymal stem cells from umbilical cord blood. Stem Cells. 2004. 22:625–634.
8. Shih DT, Lee DC, Chen SC, Tsai RY, Huang CT, Tsai CC, et al. Isolation and characterization of neurogenic mesenchymal stem cells in human scalp tissue. Stem Cells. 2005. 23:1012–1020.
9. Zuk PA, Zhu M, Mizuno H, Huang J, Futrell JW, Katz AJ, et al. Multilineage cells from human adipose tissue: implications for cell-based therapies. Tissue Eng. 2001. 7:211–228.
10. Zuk PA, Zhu M, Ashjian P, De Ugarte DA, Huang JI, Mizuno H, et al. Human adipose tissue is a source of multipotent stem cells. Mol Biol Cell. 2002. 13:4279–4295.
11. Seo MJ, Suh SY, Bae YC, Jung JS. Differentiation of human adipose stromal cells into hepatic lineage in vitro and in vivo. Biochem Biophys Res Commun. 2005. 328:258–264.
12. Banas A, Teratani T, Yamamoto Y, Tokuhara M, Takeshita F, Osaki M, et al. Rapid hepatic fate specification of adipose-derived stem cells and their therapeutic potential for liver failure. J Gastroenterol Hepatol. 2009. 24:70–77.
13. Banas A, Teratani T, Yamamoto Y, Tokuhara M, Takeshita F, Osaki M, et al. IFATS collection: in vivo therapeutic potential of human adipose tissue mesenchymal stem cells after transplantation into mice with liver injury. Stem Cells. 2008. 26:2705–2712.
14. Banas A, Teratani T, Yamamoto Y, Tokuhara M, Takeshita F, Quinn G, et al. Adipose tissue-derived mesenchymal stem cells as a source of human hepatocytes. Hepatology. 2007. 46:219–228.
15. Liang L, Ma T, Chen W, Hu J, Bai X, Li J, et al. Therapeutic potential and related signal pathway of adipose-derived stem cell transplantation for rat liver injury. Hepatol Res. 2009. 39:822–832.
16. Sato Y, Araki H, Kato J, Nakamura K, Kawano Y, Kobune M, et al. Human mesenchymal stem cells xenografted directly to rat liver are differentiated into human hepatocytes without fusion. Blood. 2005. 106:756–763.
17. Sgodda M, Aurich H, Kleist S, Aurich I, König S, Dollinger MM, et al. Hepatocyte differentiation of mesenchymal stem cells from rat peritoneal adipose tissue in vitro and in vivo. Exp Cell Res. 2007. 313:2875–2886.
18. Rehman J, Traktuev D, Li J, Merfeld-Clauss S, Temm-Grove CJ, Bovenkerk JE, et al. Secretion of angiogenic and antiapoptotic factors by human adipose stromal cells. Circulation. 2004. 109:1292–1298.
19. Lee SW, Min SO, Kim SY, Choi SB, Kim HO, Kim KS. Mesenchymal stem cells: the promotion of endodermal-induction using activin A. Korean J Hepatobiliary Pancreat Surg. 2009. 13:205–214.
20. Petersen BE, Bowen WC, Patrene KD, Mars WM, Sullivan AK, Murase N, et al. Bone marrow as a potential source of hepatic oval cells. Science. 1999. 284:1168–1170.
21. Barry FP, Murphy JM. Mesenchymal stem cells: clinical applications and biological characterization. Int J Biochem Cell Biol. 2004. 36:568–584.
22. Strem BM, Hicok KC, Zhu M, Wulur I, Alfonso Z, Schreiber RE, et al. Multipotential differentiation of adipose tissue-derived stem cells. Keio J Med. 2005. 54:132–141.
23. Kern S, Eichler H, Stoeve J, Klüter H, Bieback K. Comparative analysis of mesenchymal stem cells from bone marrow, umbilical cord blood, or adipose tissue. Stem Cells. 2006. 24:1294–1301.
24. Aurich I, Mueller LP, Aurich H, Luetzkendorf J, Tisljar K, Dollinger MM, et al. Functional integration of hepatocytes derived from human mesenchymal stem cells into mouse livers. Gut. 2007. 56:405–415.
25. De Falco E, Porcelli D, Torella AR, Straino S, Iachininoto MG, Orlandi A, et al. SDF-1 involvement in endothelial phenotype and ischemia-induced recruitment of bone marrow progenitor cells. Blood. 2004. 104:3472–3482.
26. Gilchrist ES, Plevris JN. Bone marrow-derived stem cells in liver repair: 10 years down the line. Liver Transpl. 2010. 16:118–129.
27. Hattori K, Heissig B, Tashiro K, Honjo T, Tateno M, Shieh JH, et al. Plasma elevation of stromal cell-derived factor-1 induces mobilization of mature and immature hematopoietic progenitor and stem cells. Blood. 2001. 97:3354–3360.
28. Mavier P, Martin N, Couchie D, Préaux AM, Laperche Y, Zafrani ES. Expression of stromal cell-derived factor-1 and of its receptor CXCR4 in liver regeneration from oval cells in rat. Am J Pathol. 2004. 165:1969–1977.
29. Peled A, Petit I, Kollet O, Magid M, Ponomaryov T, Byk T, et al. Dependence of human stem cell engraftment and repopulation of NOD/SCID mice on CXCR4. Science. 1999. 283:845–848.
30. Aiuti A, Webb IJ, Bleul C, Springer T, Gutierrez-Ramos JC. The chemokine SDF-1 is a chemoattractant for human CD34+ hematopoietic progenitor cells and provides a new mechanism to explain the mobilization of CD34+ progenitors to peripheral blood. J Exp Med. 1997. 185:111–120.