Abstract
Purpose
It has been suggested that constitutive up-regulation of cyclooxygenase (COX)-2 is associated with resistance to apoptosis, increased angiogenesis, and increased tumor invasiveness in various cancers including colon cancer. There are many factors involved in the resistance to 5-fluorouracil (5-FU) in colon cancer. However, little is known about the role of COX-2 in acquired resistance to 5-FU in colon cancer.
Methods
Hence we investigated whether COX-2 contribute to acquired resistance to 5-FU in colon cancer cells, using cytotoxicity assay for cell survival, reverse transcription-polymerase chain reaction (RT-PCR) for vascular endothelial growth factor (VEGF), quantitative RT-PCR for COX-1 and COX-2, and enzyme-linked immunosorbent assay for PGE2.
Results
The 5-FU resistant colon cancer cells, SNU-C5/5FUR, showed increased expression of COX-2, prostaglandin E2 (PGE2), and VEGF, compared to its parental cell (SNU-C5). By treatment with meloxicam, the expression of PGE2 and VEGF was reduced significantly in the resistant cells, but not in the parent cells.
5-Fluorouracil (5-FU) is a widely used chemotherapeutic agent that inhibits cancer cell growth and initiates apoptosis by targeting thymidylate synthase (TS), and by direct incorporation of 5-FU metabolites into DNA and RNA [1]. 5-FU-based chemotherapy improves the overall and disease-free survival of patients with solid cancers, including colorectal cancer. However, de novo and acquired chemoresistance are major obstacles to the success of 5-FU-based chemotherapy. The overexpression of TS has been shown to be a major 5-FU resistance-inducing factor [2]. However, the overexpression of TS does not account for all non-responding tumors in colorectal cancer patients treated with 5-FU [3]. High expression levels of dihydropyrimidine dehydrogenase (DPD), the genetic status of p53, NF-kB, DNA mismatch-repair genes, and cell cycle disturbance have also been reported to be associated with 5-FU resistance [3-7]. Thus, the mechanisms of resistance to 5-FU are believed to be multi-factorial.
The up-regulation of cyclooxygenase (COX)-2 expression is an early and key oncogenic event in human colon neoplasia, and occurs in 85% of colon cancers and in 50% of colon adenomas [8]. It has also been suggested that the overexpression of COX-2 in colorectal cancer is associated with tumor growth, angiogenesis, lymphatic invasion, and metastasis [9]. Nonsteroidal anti-inflammatory drugs (NSAIDs) targeting COX-2 have been shown to directly shrink colon adenomas in some patients and to mediate cancer cell apoptosis [10,11]. Similarly, in mice, the genetic inactivation of COX-2 blocks murine intestinal adenoma development [12,13]. Most actions of COX-2 are known to be mediated by prostaglandin E2 (PGE2). Furthermore, it has been reported that the anti-tumor effects of 5-FU are increased when it is co-treated with COX-2 inhibitors [14,15]. However, little is known about the role of COX-2 in acquired resistance to 5-FU in colon cancer cells.
In the present study, we investigated whether COX-2-derived PGE2 contributes to acquired resistance to 5-FU in colon cancer cells.
The SNU-C5 colon cancer cell line (KCLB no. 0000C5) was obtained from the Korean Cell Line Bank (Seoul, Korea) and cultured at 37℃ in a 5% CO2 atmosphere using Roswell Park Memorial Institute (RPMI) 1640 medium (Invitrogen, Gland Island, NY, USA) with 10% heat inactivated fetal bovine serum (FBS, Sigma-Aldrich Co., St. Louis, MO, USA). The 5-fluorouracil-resistant SNU-C5 subline, SNU-C5/5FUR, was selected from its parental cell line, SNU-C5, after chronic exposure to an intermittent dose schedule of 5-FU (Sigma-Aldrich Co.) in order to facilitate the expression of the resistant phenotype. 5-FU was initially added at 1 × IC50 (50% inhibitory concentration, 17.5 µM), and subsequently increased at a rate of 50%. Finally, the cells were cultured in a fixed 5-FU concentration (140 µM) [16]. When the sensitivities of SNU-C5 and SNU-C5/5FUR cells to 5-FU were determined using 3-(4, 5-dimethylthiazol-2-yl)-2,5-diphenyl tetrazolium bromide (MTT) assays, the IC50 values of SNU-C5 and SNU-C5/5FUR cells were 7.8 µM and 337.4 µM, respectively, i.e., SNU-C5/5FUR cells were 43.6 times more resistant to 5-FU than SNU-C5 cells.
The in vitro cytotoxicities of 5-FU and/or meloxicam (COX-2 inhibitor, Sigma-Aldrich Co.) were determined by using MTT assays [17]. Ninety-µL aliquots of cell suspensions, at 1 × 105 cells/mL in a RPMI 1640 medium containing 10% FBS, were seeded into a 96-well microplate containing 10 µL/well of a drug. Wells containing no drugs were used as cell viability control. A stock solution of 5 mg/mL of MTT (Sigma-Aldrich Co.) was prepared in normal saline and then stored at -20℃. After cells had been incubated at 37℃ for 3 days, an aliquot of 10 µL of MTT solution was added to each well, shaken for 1 minute, and incubated for 4 hours. Formazan crystals were dissolved with dimethylsulfoxide. The optical density of the wells was measured using a microplate reader at a wavelength of 540 nm. The IC50 of a drug was defined as the concentration that caused a 50% reduction in cell number versus the untreated control. IC50 values were directly determined from semi-logarithmic dose-response curves. All experiments were carried out at least in triplicate.
Extraction of mRNA from SNU-C5/WT and SNU-C5/5FUR was performed using the RNeasy protocol (Qiagen, Hilden, Germany). One microgram of total RNA was reverse transcribed into cDNA in a volume of 20 µL using avian myeloma leukemia virus reverse transcriptase and oligo dT primers (Promega, Madison, WI, USA), according to the manufacturer's instructions. Real-time PCR measures of COX-1, COX-2, and b-actin cDNA expression were obtained using a Light Cycler (Roche Diagnostics, Mannheim, Germany) with a Fast Start DNA Master SYBR Green I Kit (Roche Diagnostics). To verify amplification products, PCR products were analyzed on 2% agarose gel and stained with ethidium bromide. The sequences of the primers used were as follows: for β-actin, 5'-GACTATGACTTAGTTGCGTTA-3' and 5'-GTTGAACTCTCTACATACTTCCG-3'; for COX-2, 5'-ATGATCTACCCTCCTCAA-3' and 5'-GAACAACTGCTCATCAC-3'; for COX-1, 5'-CATCAAGATGTACGTGGT-3' and 5'-GGAGTAGACGAAGCCC-3'. Each reaction (20 µL) contained 4 µL cDNA (5-fold dilution), 2.5 mM MgCl2, 10 pmol of each primer and 2 µL of Fast Starter Mix (containing buffer, dNTPs, SYBR Green dye and Tag polymerase). Target genes were amplified using the following conditions: pre-denaturing at 95℃ for 10 minutes, 40 cycles of denaturing at 95℃ for 15 seconds, annealing for COX-2 at 59℃; COX-1 at 60℃; β-actin at 55℃ for 5 seconds, and extension at 72℃ for 7 seconds (β-actin for 21 seconds). Melting curve analysis was performed to confirm the production of single PCR products. Negative controls without template were produced for each run. Gene expression values (relative mRNA levels) are expressed as ratios (differences between cycle threshold values) between the gene of interest (COX-1, COX-2 mRNA) and β-actin (internal control). These provided a normalization factor for the amount of RNA isolated from a given specimen. Analysis of data was performed using Light Cycler software version 4.0.
RT-PCR was used to analyze the expression of VEGF and β-actin mRNAs. One microgram of total RNA was reverse transcribed into cDNA in a volume of 20 µL containing Taq polymerase and 10 pmole of each primer in a GeneAmp PCR2400 (Perkin-Elmer, Boston, MA, USA). To confirm amplification products, PCRs were analyzed on 2% agarose gel and stained with ethidium bromide. Gels were documented using a Kodak Imaging Station 4000 MM (Eastman Kodak, Rochester, NY, USA) and digitized using UN-SCAN-IT software (SilK Scientific, Orem, UT, USA). The sequences of the primers used were follows: for β-actin, 5'-GACTATGACTTAGTTGCGTTA-3' and 5'-GTTGAACTCTCTACATACTTCCG-3'; for VEGF, 5'-AYGCGGATCAAACCTCACCAAG-3' and 5'-ACAGCAGCGGGCACCAAC-3'. Each reaction (20 µL) contained 1.25 µL cDNA, 2.5 mM MgCl2, 10 pmol of each primer and Taq polymerase (Perkin-Elmer-Cetus, Norwalk, CT, USA). The amplification of target genes were as follows: pre-denaturing at 94℃ for 5 minutes, 35 cycles of denaturing at 94℃ for 30 seconds, annealing for VEGF at 62℃ (β-actin at 55℃) for 30 seconds, and extension at 72℃ for 1 minute (β-actin for 21 seconds).
PGE2 levels in culture media were measured by using a general radioimmunoassay procedure adapted from Cetta and Goetz [18]. Briefly, in preliminary studies, prostaglandin was recovered from culture media using extraction fluid (ethyl acetate:isopropanol:0.05 N HCl = 3: 3:1); the recovery rate was relatively constant (92 ± 3%, n = 9). When PGE2 was assayed in increasing aliquots of unextracted medium (25, 50 and 100 µL), potency estimates were parallel to a linearly transformed dose response curve. Accordingly, medium samples were assayed directly without extraction. Samples were quantified using a liquid scintillation analyzer. Duplicate hormone standards (5 to 1,000 pg) were included in each assay. The between- and within-assay variation coefficients for PGE2 were 7.3% and 6.5%, respectively.
By quantitative RT-PCR, the expression levels of COX-1 and COX-2 were significantly higher in SNU-C5/5FUR than in SNU-C5. Also the ratio of COX-2 to COX-1 was higher in the resistant cells than in the parental cells (Fig. 1).
PGE2 levels present in culture media supernatants after incubation for 12 hours were determined by ELISA. For untreated cells, PGE2 levels were significantly higher in SNU-C5/5FUR than in SNU-C5 (P < 0.01). After treatment with meloxicam, PGE2 levels were significantly and dose-dependently reduced in SNU-C5/5FUR cells (P < 0.01), but not in SNU-C5 cells (Fig. 2).
To investigate whether or not meloxicam can potentiate the cytotoxic effect of 5-FU in the two cell lines, MTT assays were conducted. Meloxicam did not have any potentiating effect on 5-FU sensitivity in either cell line (Fig. 3).
Despite the above-mentioned PGE2 level increases in SNU-C5/5FUR cells, meloxicam showed little effect on overcoming resistance to 5-FU in cell culture. Thus, we investigated VEGF expression, as VEGF is the most powerful stimulator for angiogenesis, and is required for tumor growth and metastasis. VEGF mRNA levels in untreated SNU-C5/5FUR cells were higher than that in SNU-C5 cells, Ten µM to 500 µM of meloxicam down-regulated these levels in SNU-C5/5FUR cells in a dose-dependent manner. However, paradoxically meloxicam dose-dependently increased VEGF mRNA levels in SNU-C5/WT cells (Fig. 4).
The present study demonstrates that the expressions of COX-2, PGE2, and VEGF were up-regulated in the 5-FU resistant colon cancer cells rather than in the parental cells. Although meloxicam, a COX-2 inhibitor, showed little antitumor effect on both cells, it was found to have an anti-angiogenic effect by down-regulating VEGF mRNA levels in the 5-FU resistant colon cancer cells, but not in the parental cells. The expressions of TS (thymidylate synthase) and DPD, which have been suggested to be involved in 5-FU resistance, were not found to be significantly changed by cDNA microarray analysis (data not shown). It has been suggested that wild type p53 significantly improves response to 5-FU in vitro and in vivo [19,20]. In the present study, we used the colon cancer cell line, SNU-C5, and its 5-FU resistant derivative, the SNU-C5/5FUR cell line. It is known that p53 is mutated and non-functional in SNU-C5 cells [21].
It is known that COX-2 is induced by a variety of stimuli, such as, oncogenes (HER-2/neu), growth factors (EGF), tumor promoters (phorbol esters and bile acid), and chemotherapy [12]. Moreover, microtubule- interfering agents (paclitaxel, vincristine, etc) are known to induce COX-2 in cancer cells [22,23]. However, it has not been determined as to whether COX-2 is induced by 5-FU in 5-FU-resistant cancer cells. It has been reported that COX-2 mRNA expression is increased by 5-FU alone or in combination in human solid tumors, e.g., esophageal, breast, ovarian, and colorectal tumors [24]. In addition, significant increases in COX-2 expression have been reported in rectal cancer after preoperative chemoradiotherapy [25]. The present study also demonstrates that COX-2 and PGE2 levels are up-regulated in colon cancer cells with acquired resistance to 5-FU (SNU-C5/5FUR cells), which suggests that COX-2 inhibitors play a beneficial role in overcoming the resistance of SNU-C5/5FUR cells to 5-FU.
Clinically, 5-FU is used as a primary, secondary or even tertiary chemotherapy in colorectal cancer (CRC). When used as a first line chemotherapy or adjuvant therapy, 5-FU is usually used in combination with folinic acid in CRC. On the other hand, in cases that experience recurrence after treatment with the regimen, 5-FU is used again in combination with oxaliplatin (FOLFOX regimen) or irinotecan (FOLFIRI regimen). Theoretically, in cases of recurrence the effects of 5-FU are likely to be limited, and thus, some means of overcoming acquired resistance to 5-FU is required.
Irie et al. [26] reported that celecoxib (a selective COX-2 inhibitor) synergistically potentiates the antitumor effect of 5-FU in an IFN-gamma dependent manner in an in vivo study of colon cancer cells. Other reports suggested that NSAIDs could be the modulators of fluorouracil-based chemotherapy, especially in high COX-2 expressing tumors, and that combined therapy with S-1 and a COX-2 inhibitor might hold promise for prophylaxis of liver metastasis of colorectal cancer [27,28]. A similar finding was reported for ultraviolet B-induced skin tumors in mice [15]. However, we did not find that meloxicam synergistically potentiates the antitumor effect of 5-FU in either a chemonaïve colon cancer cell line and in an acquired 5-FU resistant colon cancer cell line.
It is believed that neovascularization is required for a tumor to grow to >2 to 3 mm [29]. Chang et al. [30] reported that PGE2 stimulates the expressions of angiogenic regulatory genes in mammary tumor cells isolated from COX-2 transgenic mice, which demonstrates that COX-2-derived PGE2 is a potent inducer of angiogenic switch during mammary cancer progression. In the present study, VEGF up-regulation was observed in SNU-C5/5FUR cells, and this up-regulation was recovered adding meloxicam, a COX-2 inhibitor, which suggests that COX-2-derived PGE2 plays an important role in the angiogenic switching that occurs during the acquisition of 5-FU resistance in colon cancer. Furthermore, the present study demonstrates that meloxicam exerts an anti-angiogenic effect by inhibiting PGE2 synthesis at a dose of 10 µM, which is relatively compatible with therapeutic levels. However, we had one unexpected result, i.e., SNU-C5 cells (which are chemonaïve colon cancer cells) express little VEGF or COX-2 in the basal state, but these cells were found to express VEGF after meloxicam treatment. Similarly, Ueno et al. [31] reported that treatment with celecoxib (a COX-2 inhibitor) decreased VEGF serum levels immediately after treatment but later increased its levels in serum and plasma in breast cancer patients. Thus further studies are required to determine how COX-2 inhibitors participate in VEGF production in cancer cells and tissues that do not express COX-2.
In conclusion, the authors demonstrate that COX-2 derived PGE2 is up-regulated and COX-2 inhibitor may have anti-angiogenic effect in the colon cancer cells resistant to 5-FU.
Figures and Tables
Fig. 1
mRNA expression patterns of cyclooxygenase (COX)-2 in SNU-C5 and SNU-C5/5FUR determined by quantitative reverse transcription- polymerase chain reaction. (A) Significant difference in the ratio of COX-2/β-actin between SNU-C5 and SNU-C5/5FUR (P = 0.000727)a), (B) Significant difference in the ratio COX-2/COX-1 between SNU-C5 and SNU-C5/5FUR (P = 0.00117).a)
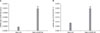
Fig. 2
Comparison of prostaglandin E2 (PGE2) levels between SNU-C5 and SNU-C5/5FUR. PGE2 levels present in the supernatant of the culture medium were determined by enzyme-linked immunosorbent assay using PGE2 monoclonal antibody. a)Compare PGE2 levels between SNU-C5 and SNU-C5/5FUR at basal state. b)Compare PGE2 levels after 12 hours treatment of meloxicam (10 µM) with control in SNU-C5/5FUR. c)Compare PGE2 levels after meloxicam (100 µM) with control in SNU-C5/5FUR, all of which have P-values less than 0.01.
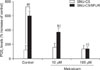
ACKNOWLEDGEMENTS
This work was supported by National Research Foundation of Korea (NRF) grant funded by the Ministry of Education, Science and Technology (MEST) through the Research Center for Resistant Cells (R13-2003-009).
References
1. Longley DB, Harkin DP, Johnston PG. 5-fluorouracil: mechanisms of action and clinical strategies. Nat Rev Cancer. 2003. 3:330–338.
2. Popat S, Matakidou A, Houlston RS. Thymidylate synthase expression and prognosis in colorectal cancer: a systematic review and meta-analysis. J Clin Oncol. 2004. 22:529–536.
3. Salonga D, Danenberg KD, Johnson M, Metzger R, Groshen S, Tsao-Wei DD, et al. Colorectal tumors responding to 5-fluorouracil have low gene expression levels of dihydropyrimidine dehydrogenase, thymidylate synthase, and thymidine phosphorylase. Clin Cancer Res. 2000. 6:1322–1327.
4. Boyer J, McLean EG, Aroori S, Wilson P, McCulla A, Carey PD, et al. Characterization of p53 wild-type and null isogenic colorectal cancer cell lines resistant to 5-fluorouracil, oxaliplatin, and irinotecan. Clin Cancer Res. 2004. 10:2158–2167.
5. Wang W, McLeod HL, Cassidy J. Disulfiram-mediated inhibition of NF-kappaB activity enhances cytotoxicity of 5-fluorouracil in human colorectal cancer cell lines. Int J Cancer. 2003. 104:504–511.
6. Meyers M, Wagner MW, Hwang HS, Kinsella TJ, Boothman DA. Role of the hMLH1 DNA mismatch repair protein in fluoropyrimidine-mediated cell death and cell cycle responses. Cancer Res. 2001. 61:5193–5201.
7. Mirjolet JF, Didelot C, Barberi-Heyob M, Merlin JL. G(1)/S but not G(0)/G(1)cell fraction is related to 5-fluorouracil cytotoxicity. Cytometry. 2002. 48:6–13.
8. Brown JR, DuBois RN. COX-2: a molecular target for colorectal cancer prevention. J Clin Oncol. 2005. 23:2840–2855.
9. Yamauchi T, Watanabe M, Kubota T, Hasegawa H, Ishii Y, Endo T, et al. Cyclooxygenase-2 expression as a new marker for patients with colorectal cancer. Dis Colon Rectum. 2002. 45:98–103.
10. Williams CS, Mann M, DuBois RN. The role of cyclooxygenases in inflammation, cancer, and development. Oncogene. 1999. 18:7908–7916.
11. Fosslien E. Biochemistry of cyclooxygenase (COX)-2 inhibitors and molecular pathology of COX-2 in neoplasia. Crit Rev Clin Lab Sci. 2000. 37:431–502.
12. Subbaramaiah K, Dannenberg AJ. Cyclooxygenase 2: a molecular target for cancer prevention and treatment. Trends Pharmacol Sci. 2003. 24:96–102.
13. Jacoby RF, Seibert K, Cole CE, Kelloff G, Lubet RA. The cyclooxygenase-2 inhibitor celecoxib is a potent preventive and therapeutic agent in the min mouse model of adenomatous polyposis. Cancer Res. 2000. 60:5040–5044.
14. Mizutani Y, Kamoi K, Ukimura O, Kawauchi A, Miki T. Synergistic cytotoxicity and apoptosis of JTE-522, a selective cyclooxygenase-2 inhibitor, and 5-fluorouracil against bladder cancer. J Urol. 2002. 168:2650–2654.
15. Wilgus TA, Breza TS Jr, Tober KL, Oberyszyn TM. Treatment with 5-fluorouracil and celecoxib displays synergistic regression of ultraviolet light B-induced skin tumors. J Invest Dermatol. 2004. 122:1488–1494.
16. Jung GR, Kim KJ, Choi CH, Lee TB, Han SI, Han HK, et al. Effect of betulinic acid on anticancer drug-resistant colon cancer cells. Basic Clin Pharmacol Toxicol. 2007. 101:277–285.
17. Pieters R, Huismans DR, Leyva A, Veerman AJ. Adaptation of the rapid automated tetrazolium dye based (MTT) assay for chemosensitivity testing in childhood leukemia. Cancer Lett. 1988. 41:323–332.
18. Cetta F, Goetz FW. Ovarian and plasma prostaglandin E and F levels in brook trout (Salvelinus fontinalis) during pituitary-induced ovulation. Biol Reprod. 1982. 27:1216–1221.
19. Arango D, Corner GA, Wadler S, Catalano PJ, Augenlicht LH. c-myc/p53 interaction determines sensitivity of human colon carcinoma cells to 5-fluorouracil in vitro and in vivo. Cancer Res. 2001. 61:4910–4915.
20. Kim HJ, Lee SC, Lee IK, Kang WK, Oh ST, Chang SK. The synergistic cell killing effects by the transduction of the w-p53 Gene and 5-FU administration in colon cancer cell lines. J Korean Surg Soc. 2007. 73:481–489.
21. Rand A, Glenn KS, Alvares CP, White MB, Thibodeau SM, Karnes WE Jr. p53 functional loss in a colon cancer cell line with two missense mutations (218leu and 248trp) on separate alleles. Cancer Lett. 1996. 98:183–191.
22. Subbaramaiah K, Hart JC, Norton L, Dannenberg AJ. Microtubule-interfering agents stimulate the transcription of cyclooxygenase-2. Evidence for involvement of ERK1/2 AND p38 mitogen-activated protein kinase pathways. J Biol Chem. 2000. 275:14838–14845.
23. Mathieu A, Remmelink M, D'Haene N, Penant S, Gaussin JF, Van Ginckel R, et al. Development of a chemoresistant orthotopichuman nonsmall cell lung carcinoma model in nude mice: analyses of tumor heterogenity in relation to the immunohistochemical levels of expression of cyclooxygenase-2, ornithine decarboxylase, lung-related resistance protein, prostaglandin E synthetase, and glutathione-S-transferase-alpha (GST)-alpha, GST-mu, and GST-pi. Cancer. 2004. 101:1908–1918.
24. Mercer SJ, Di Nicolantonio F, Knight LA, Gabriel FG, Whitehouse PA, Sharma S, et al. Rapid up-regulation of cyclooxygenase-2 by 5-fluorouracil in human solid tumors. Anticancer Drugs. 2005. 16:495–500.
25. Debucquoy A, Goethals L, Geboes K, Roels S, Mc Bride WH, Haustermans K. Molecular responses of rectal cancer to preoperative chemoradiation. Radiother Oncol. 2006. 80:172–177.
26. Irie T, Tsujii M, Tsuji S, Yoshio T, Ishii S, Shinzaki S, et al. Synergistic antitumor effects of celecoxib with 5-fluorouracil depend on IFN-gamma. Int J Cancer. 2007. 121:878–883.
27. Réti A, Pap E, Zalatnai A, Jeney A, Kralovánszky J, Budai B. Co-inhibition of cyclooxygenase-2 and dihydropyrimidine dehydrogenase by non-steroidal anti-inflammatory drugs in tumor cells and xenografts. Anticancer Res. 2009. 29:3095–3101.
28. Tachimori A, Yamada N, Amano R, Ohira M, Hirakawa K. Combination therapy of S-1 with selective cyclooxygenase-2 inhibitor for liver metastasis of colorectal carcinoma. Anticancer Res. 2008. 28(2A):629–638.
29. Wang D, DuBois RN. Cyclooxygenase 2-derived prostaglandin E2 regulates the angiogenic switch. Proc Natl Acad Sci U S A. 2004. 101:415–416.
30. Chang SH, Liu CH, Conway R, Han DK, Nithipatikom K, Trifan OC, et al. Role of prostaglandin E2-dependent angiogenic switch in cyclooxygenase 2-induced breast cancer progression. Proc Natl Acad Sci U S A. 2004. 101:591–596.
31. Ueno T, Chow LW, Toi M. Increases in circulating VEGF levels during COX-2 inhibitor treatment in breast cancer patients. Biomed Pharmacother. 2006. 60:277–279.