Abstract
Objectives
We examined the effect of sildenafil citrate on advanced glycation end products (AGEs)-induced soluble fms-like tyrosine kinase 1 (sFlt-1) release in JEG-3 choriocarcinoma cells.
Methods
Cells were incubated with control bovine serum albumin (BSA) or AGEs-BSA, and expression of sFlt-1 mRNA and protein release was determined by quantitative reverse transcription-polymerase chain reaction (qRT-PCR) and enzyme-linked immunosorbent assay (ELISA), respectively. AGEs-BSA increased sFlt-1 mRNA expression and protein release in a dose-dependent manner.
Results
Sildenafil citrate suppressed sFlt-1 mRNA expression and protein release in cells treated with AGEs-BSA in a dose-dependent manner. Likewise, it inhibited the increase of reactive oxygen species (ROS) production and NF-κB activity in these cells. Cobalt protoporphyrin (CoPP) and bilirubin also inhibited sFlt-1 release and ROS production in cells treated with AGEs-BSA, whereas zinc protoporphyrin IX (ZnPP IX) antagonized the effect of sildenafil citrate. In cells transfected with the heme oxygenase-1 (HO-1) siRNA, sildenafil citrate failed to inhibit the sFlt-1 release and ROS production.
Preeclampsia is one of the most common obstetrical complications affecting up to 8% of all gestations1 and remains major causes of maternal and neonatal mortality and morbidity in Korea and worldwide. It typically presents after 20th week of gestation and is characterized by new-onset hypertension with proteinuria and/or edema during pregnancy.2 It is accepted that abnormal implantation of the blastocyst and abnormal placental bed remodeling or other causes of reduced placental perfusion are prerequisites for preeclampsia,1 but the mechanistic links between this step and the development of preeclampsia are still largely unknown.
A number of molecular pathways have been suggested to be involved in the cascade from placental events to maternal dysfunction. One of them which has been the subject of intense research is the production of the soluble form of the vascular endothelial growth factor (VEGF) receptor-1. The soluble VEGF receptor-1 is also denoted as soluble fms-like tyrosine kinase-1 (sFlt-1) as it belongs to fms-related tyrosine kinase family.3 The proto-oncogene fms encodes the tyrosine kinase transmembrane receptor. It has been shown that sFlt-1 is produced in the placenta during preeclampsia and is found to be elevated in the circulation of preeclamptic women, often before the onset of maternal symptoms.3,4 In addition, numerous experimental models have demonstrated the implication of sFlt-1 overexpression in the development of preeclampsia.5,6,7,8 In maternal circulation, sFlt-1 binds to free VEGF and placental growth factor (PlGF), sequestering and making them unavailable for proper signaling.3
Advanced glycation end-products (AGEs) represent the molecular complexes generated as a result of non-enzymatic reactions of carbohydrates and oxidized lipid with proteins. These reactions leads to irreversible cross-linking of proteins, and as a consequence, loss of protein structure and function.9 Accumulation of AGEs contribute to diverse metabolic disorders such as diabetes,10 obesity,11 metabolic syndromes,12 and polycystic ovary syndrome.13 The women with such disorders, when in pregnancy, have a higher risk of preeclampsia.1 Previous studies demonstrated that serum AGEs in preeclamptic women were significantly higher than in healthy women.14,15 Accumulation of AGEs may induce oxidative injury and vascular perturbation in placental bed, leading to preeclampsia.16 A recent study demonstrated that AGEs might contribute to the pathogenesis of preeclampsia by promoting sFlt-1 production in extravillous trophoblasts, implicating AGEs in a novel bridge between metabolic disorders and preeclampsia.17
Sildenafil, a specific inhibitor of cyclic guanosine monophosphate (cGMP)-specific phosphodiesterase type 5 (PDE-5), has evolved from a potential anti-angina drug into an oral treatment for erectile dysfunction (Viagra®; Pfizer, New York, NY, USA), and more recently, into a new orally active treatment for pulmonary arterial hypertension (Revatio®; Pfizer, New York, NY, USA).18 Sildenafil prolongs the relaxation and vasodilatation actions of cGMP on corpus cavernosal smooth muscle cells by inhibiting the breakdown of nitric oxide (NO)-driven cGMP.18
Theoretically, a therapeutic approach that could cause dilatation of the uterine or systemic vessels could restore placental perfusion, vascular tones of systemic arteries and consequently ameliorate the preeclamptic symptoms. Considering the well recognized action of sildenafil as a vasodilating agent, it would not be absurd to expect beneficial effects from sildenafil to alleviate preeclamptic signs and symptoms. Although trials in human patients did not provide confirmative results, these studies have left open the possibility that PDE-5 inhibitors could be beneficial to the preeclamptic patient.19,20,21 Animal studies with rat models of preeclampsia induced by NG-nitro-L-arginine methyl ester (L-NAME) treatment22 and placental ischemia23 showed that sildenafil treatment significantly reduced the preeclamptic signs and symptoms, such as hypertension, proteinuria and fetal mortality. Furthermore, sildenafil was shown to suppress elevation of the plasma levels of anti-angiogenic factors including sFlt-1.24 These results raised hope for the therapeutic potential of sildenafil as an effective option for the management of preeclampsia.
JEG-3, a choriocarcinoma cell line, may serve as an appropriate in vitro trophoblastic model to study preventive and therapeutic agents in the field of preeclampsia.25 This could help to understand of the association between sFlt-1 and other gynecologic conditions such as endometriosis and menopause.26 These cells have been shown to preserve responses to hypoxia to increase sFlt-1 mRNA expression and protein release.27 In this study, we examined the effect of sildenafil on sFlt-1 release in the AGEs-treated JEG-3 cells, and evaluated the role of the heme oxygenase-1 (HO-1) as a key molecule to mediate the action of sildenafil.
JEG-3 choriocarcinoma cells obtained from American Type Culture Collection were routinely maintained on plastic culture flasks in Dulbecco's Modified Eagle's Medium/Nutrient Mixture F-12 Ham (DMEM/F12) supplemented with 10% fetal bovine serum (FBS), 50 IU/mL penicillin G and 50 µg/mL streptomycin. Cells were trypsinized when cells became confluent (approximately every 4-5 days) using 0.05% trypsin/0.53 mM ethylenediaminetetraacetic acid (EDTA) solution and re-seeded at one-sixth the original density. Before 1 h to exposure to experimental protocols, cells were switched to serum-free media.
AGEs-bovine serum albumin (AGEs-BSA) was prepared as previously described.28 Endotoxin-free BSA (Calbiochem, La Jolla, CA, USA) of 5 g was incubated with 9 g D-glucose in 100 mL phosphate buffer (0.2 M, pH 7.4). The mixed solution was filtered through 0.22 µm microporous membrane filter and then maintained in sterile conditions in the dark at 37℃ for 3 months. At the end of incubation, unincorporated glucoses and low molecular reactants were removed by dialysis overnight against 0.1 M phosphate buffer. For BSA control BSA was incubated under the same conditions without glucose. Every preparation was tested for endotoxin by the limulus amebocytelysate assay (E-Toxate kit; Sigma-Aldrich, St Louis, MO, USA), and stored at 4℃ for use when no endotoxin was detected.
The efficiency of AGE-BSA formation was assessed by fluorescence spectrophotometric analysis (excitation 360 nm, emission 450 nm) and measurement of Nε-carboxymethyl-lysine (CML) by enzyme-linked immunosorbent assay (ELISA). AGEs-BSA was characterized by a 16 fold increase in glycation fluorescence and a 86-fold higher CML concentration compared to the unmodified BSA (17.2 nmoL/mg protein in AGEs-BSA vs. 0.2 nmoL/mg protein in unmodified BSA).
Total RNA was extracted using Trizol (Invitrogen, Carlsbad, CA, USA). In order to remove contaminating genomic DNA, 1 mg of RNA was treated with DNase (DNase I Amplification Grade; Invitrogen, Carlsbad, CA, USA) at room temperature for 15 min and the reaction was terminated with 25 mM EDTA for 10 min at 65℃. RNA was quantified by spectrometry and used for quantitative RT-PCR (qRT-PCR). qRT-PCR assays were carried out to quantify mRNAs for sFlt-1, receptors for AGEs (RAGE), HO-1 and β-actin. Real-time quantitation was based on the LightCycler assay, using a fluorogenic SYBR Green I re action mixture for PCR with the LightCycler Instrument (Roche Diagnostics, Mannheim, Germany). The PCR reactions were carried out in a total volume of 20 µL, which included SYBR Green I with a Taq DNA polymerase reaction buffer, deoxynucleotide triphosphate mixture, 10 mM MgCl2, PCR-grade water, 2 µL of template DNA, and primers. The sequences of the chosen primers were given in Table 1.
sFlt-1 protein was measured by ELISA by using Quantikine Human ELISA kit (R&D Systems, Minneapolis, MN, USA).
The intracellular generation of ROS was measured using 2',7'-dichlorodihydrofluorescein diacetate (DCFH-DA). The nonfluorescent ester penetrates into the cells and is hydrolyzed to DCFH by the cellular esterases. The probe (DCFH) is rapidly oxidized to the highly fluorescent compound 2',7'-dichlorofluorescein (DCF) in the presence of cellular peroxidase and ROS such as hydrogen peroxide or fatty acid peroxides. Cells cultured in 24-well plate were preincubated in the culture medium with 10 M DCFH-DA for 1 h at 37℃. After the preincubation, cells were exposed to experimental protocols for various durations. Changes in DCF fluorescence was assayed using FACSort Becton Dickinson Flow Cytometer (Becton-Dickinson Bioscience, San Jose, CA, USA) and data were analyzed with CELLQuest Software.
Luciferase reporter gene assay was performed to measure activity. Transient transfections were performed with Lipofectamine Plus Reagent (Invitrogen, Carlsbad, Ca, USA) using pNF-κB-Luc and pCMV-β-Gal plasmids (Clontech Laboratories Inc., Palo Alto, CA, USA) The reporter gene assay was performed according to the manufacturer-provided method.
HO-1 protein was determined by Western blot analysis. Total intracellular protein was extracted by 5X repeated freeze-thaw lysis in FT buffer (600 mM KCl, 20 mM Tris-Cl, pH 7.8, 20% glycerol, 0.4 mg/mL Pefabloc, 10 µg/mL leupeptin, 10 µg/mL pepstatin, and 5 µg/mL aprotinin). Protein concentration was determined by Bradford assay (Bio-Rad). For Western blots, 30 µg of protein was subjected to sodium dodecyl sulfate-polyacrylamide gel electrophoresis (SDS-PAGE) on 4-0% gradient SDS-PAGE (Bio-Rad). Membranes were blocked with Odyssey blocking buffer (LI-COR, Lincoln, NE, USA) for 2 h at room temperature. Primary incubation was undertaken overnight at 4℃ with a rabbit anti-HO-1 polyclonal antibody (StressGen, San Diego, Ca, USA) at 1 : 2000 and a mouse anti-β-actin antibody (Gentest) at 1 : 5000. Secondary antibody incubation was done with Alexa Fluor 680 goat anti-rabbit (Molecular Probes) and IRDye 800 goat antimouse IgG (Rockland) for 1 h at room temperature. Fluorescence was detected on an Odyssey infrared imager (LI-COR) for simultaneous detection of both species. Blot analysis was performed with the supplied Odyssey software, and HO-1 was normalized to β-actin.
siRNA and scrambled siRNA against human HO-1 were purchased from Santa Cruz Biotechnology (Santa Cruz, Ca, USA), and transient transfections were performed using the Superfect® transfection reagent from QIAGEN (Hilden, Germany). The cells were incubated with the siRNA and scrambled siRNA at a concentration of 100 nM for 16 h in serum free media.
We examined first the JEG-3 choriocarcinoma cells used in this study preserve the basal expression and release of sFlt-1. qRT-PCR and ELISA analyses showed that the JEG-3 cells preserve baseline sFlt-1 mRNA expression and protein release. Control BSA did not affect the baseline sFlt-1 mRNA expression and protein release, whereas AGEs-BSA stimulated both the sFlt-1 mRNA expression and protein release in a concentration-dependent manner. When cells were treated with 200 µg/mL AGEs-BSA for 24 h, there were 3.5 fold and 2.7 fold increases, respectively, in mRNA expression and protein release (Fig. 1).
To examine whether the effect of AGEs-BSA in this study resulted from interaction with RAGE, the effect of anti-RAGE antibody was determined. In the presence of anti-RAGE antibody, AGEs-BSA failed to increase the sFlt-1 mRNA expression and protein release, indicating that interaction with RAGE is essential for the process of cellular actions of AGEs-BSA (Fig. 2).
Increased ROS production and NF-κB activation has been shown to be implicated in the AGE-related pathophysiological processes.29,30 In significantly increased ROS production and NF-κB activity. Anti-RAGE antibody inhibited the AGEs-BSA-induced stimulation of ROS production and NF-κB activity (Fig. 3).
To determine the effect of sildenafil citrate on AGEs-BSA-induced cellular effects, cells were incubated with AGEs-BSA for 24 h in the presence of sildenafil citrate (indicated concentrations or 20 µM, unless otherwise indicated). Sildenafil citrate did not affect the baseline sFlt-1 mRNA expression and protein release (data not shown), whereas it inhibited the AGEs-BSA-stimulated sFlt-1 mRNA expression (Fig. 4A) and protein release (Fig. 4B) in a concentration-dependent manner. Sildenafil citrate also suppressed the AGEs-BSA-induced stimulation of ROS production (Fig. 5A) and NFkB activity (Fig. 5B). AGEs-induced cellular effects have been known to be closely related with increased expression of RAGE. In the present study, in cells treated with AGEs-BSA a significant increase in the expression of RAGE mRNA was observed. In these cells, as well as in BSA control cells, sildenafil citrate did not show any significant effect on the RAGE mRNA expression.
Several investigators showed that increased expression of HO-1 might inhibit sFlt-1 release and preeclamptic complications.31,32,33 Hence, we determined whether sildenafil citrate affects the HO-1 expression. qRT-PCR and Western blot analyses showed that sildenafil citrate significantly stimulated expression of HO-1 mRNA and protein in the cells treated with BSA control and AGEs-BSA. Treatment with AGEs-BSA alone did not affect the HO-1 mRNA and protein expression (Fig. 6).
In order to determine whether the effect of sildenafil citrate to inhibit sFlt-1 release and ROS production in cells treated with AGEs-BSA is related with the promoted expression of HO-1, we determined the effects of HO-1 related molecules. The HO-1 inducer, cobalt protoporphyrin (CoPP),34 and the metabolic product of HO-1, bilirubin,34 inhibited the AGEs-BSA-induced stimulation of sFlt-1 release. These effects were comparable to the sildenafil citrate-mediated inhibition of sFlt-1 release. On the contrary, the HO-1 inhibitor, zinc protoporphyrin IX (ZnPP IX)34 attenuated the effect of sildenafil citrate (Fig. 7A). Similar results were observed in the experiments to examine the effects on ROS production (Fig. 7B). These results strongly suggest that sildenafil citrate-induced inhibition of sFlt-1 release and ROS production in cells treated with AGEs-BSA is closely related with upregulated expression of HO-1.
Small interfering RNA approaches were used to confirm further the role of HO-1 in the sildenafil citrate-induced inhibition of sFlt-1 release and ROS production in cells treated with AGEs-BSA. Western blot analysis showed that the basal expression of the HO-1 was sinificantly diminished by transfection with the HO-1 siRNA. In these cells, CoPP and sildenafil citrate failed to increase the expression of HO-1 (Fig. 8A). Likewise, in cells transfected with the HO-1 siRNA, sildenafil citrate failed to inhibit the AGEs-BSA-induced stimulation of sFlt-1 release and ROS production (Fig. 8B, 8C). These results confirm our hypothesis that upregulation of the HO-1 expression is the crucial mechanism to mediate the sildenafil citrate-induced inhibition of the sFlt-1 release and ROS production in cells treated with AGEs-BSA.
Preeclampsia remains a major complication of pregnancy, affecting at least one out of twenty pregnancies.1 Besides the risk for mother and fetus during pregnancy and delivery, preeclampsia also impacts long-term health. Systemic review and meta-analysis reported that women with preeclampsia are at two-fold increased risk of developing cardiovascular disorders in later life.35 The later impact of preeclampsia is not confined to maternal health. It confers increased risk for cardiovascular complications to the offspring as well.36 Though the etiological cause of preeclampsia is not fully understood yet, diverse metabolic disorders have been recognized as predisposing causes toward the disease.1 AGEs have been implicated in the molecular bridge between the metabolic disorder and preeclampsia. Previous studies demonstrated that elevation of serum AGEs is closely related to the development of preeclampsia.14,15 A recent study demonstrated that AGEs might contribute to the pathogenesis of preeclampsia by promoting sFlt-1 production in extravillous trophoblasts, suggesting that AGEs might be an upstream molecule that promotes sFLt-1 expression in trophoblasts and contributes to the pathogenesis of preeclampsia.17 In the present study we also observed that AGEs-BSA increased sFlt-1 mRNA expression and protein release (Fig. 1). Although JEG-3 cells used in this study is a cell line established from choriocarcinoma, it has been shown to preserve trophoblastic cell-like characteristics, and might serve as an appropriate in vitro trophoblastic model for studies in various fields including preeclampsia.25 Thus, the results in this study can give us a valuable insight into trophoblastic mechanism responsible for the pathophysiology of preeclampsia.
AGEs exert their cellular effects through the interaction with RAGE, AGE-R3 (galectin-3), and maybe other AGE-binding proteins. Among them, RAGE is the best characterized AGE receptor and responsible for most of the deleterious effects of AGEs, whereas AGE-R3 is probably implicated in the removal of AGEs.37 In the presence of anti-RAGE antibody, AGEs-BSA did not affect the sFlt-1 mRNA expression and protein release, indicating that AGEs-RAGE interaction is essential for the process of cellular actions of AGEs-BSA (Fig. 2). AGEs-BSA-induced increases in ROS production and NF-κB activity are in accord with the previous understanding that increased ROS production and NF-κB activation is implicated in the AGE-related pathophysiological processes.29,30 In the presence of anti-RAGE antibody, AGEs-BSA failed to stimulate ROS production and NF-κB activity suggesting that these processes are also mediated through AGEs-RAGE interaction (Fig. 3).
Management of preeclampsia is still restricted by a lack of effective therapeutic agents, and there has been interest in using PDE-5 inhibitors in this patient population. Studies with several animal models of preeclampsia have demonstrated beneficial improvements in fetal outcome when treated with sildenafil.22,23,38,39 Herraiz et al.38 and Ramesar et al.22,24 demonstrated that the deleterious effect of NO synthas (NOS) inhibition during pregnancy could be significantly attenuated by sildenafil administration. Decrease in the bioavailability of NO is one of the most commonly recognized mechanisms of vascular dysfunction in preeclampsia. Normal pregnancy is associated with an increase in endothelium-derived NO, which is concomitant with overall increases in vasodilation and vascular compliance, which seems to be regulated by increased NOS activity.40,41,42 Indeed, pregnancy induces a significant increase in circulating levels of cGMP in the mother, suggesting increased NOS activity. Then, it would be logical to assume that a decrease in NO could be a major contributor to the endothelial dysfunction, and likewise, a restoration of NO bioavailability or cGMP with PDE-5 inhibitors could have a palliative effect in the preeclampsia patient. Further understanding of preeclampsia could improve general conditions of postpartum women and possibly connected to general welfare of menopausal women.
In the present study sildenafil citrate suppressed sFlt-1 mRNA expression and protein release in cells treated with AGEs-BSA in a dose-dependent manner (Fig. 4). Likewise, it inhibited the increase of ROS production and NFkB activity in these cells (Fig. 5A, 5B). These observations could provide us with a new insight into pharmacological action of sildenafil citrate. In addition to its well-recognized vasodilatory effect, the therapeutic targets of sildenafil citrate for preeclampsia may be widened to include the mechanisms within trophoblastic cell per se. In this regard, a previous report is notable, which has shown that systemic administration of sildenafil to L-NAME treated preeclampsia model rats decreased the plasma levels of anti-angiogenic factors including sFlt-1.24
Expression of RAGE proteins is known to be regulated by AGEs. In this study, increased expression of RAGE mRNA was observed in cells treated with AGEs-BSA indicating that AGEs-BSA upregulated the expression of RAGE. Sildenafil citrate did not affect the basal or AGEs-BSA stimulated expression of RAGE, indicating that action of sildenafil citrate does not seem to be related with regulation of RAGE expression.
One of the potential tools which has been proven effective in numerous experimental forms of hypertension is the manipulation of the HO-1 pathway.43,44,45,46 HO-1 produces two bioactive compounds as products of heme metabolism: carbon monoxide (CO) and bilirubin. Bilirubin functions as a powerful antioxidant.47,48 As production of ROS has been known to be a major component of varied forms of hypertension, moderate increase in bilirubin could act to decrease overall oxidative stress. CO acts as a potent vasodilator, functioning in a manner similar to NO.49 Of particular interest to preeclampsia, HO-1 and CO have been shown to directly inhibit production of VEGF and interferon-γ-induced secretion of sFlt-1.31
The present study strongly suggests the involvement of HO-1 in sildenafil citrate-induced inhibition of sFlt-1 release and ROS formation. qRT-PCR and Western blot analyses showed that sildenafil citrate upregulated the HO-1 expression (Fig. 6). Furthermore, the HO-1 inducer CoPP29 and metabolic product of HO-1 bilirubin29 also inhibited sFlt-1 release and ROS production in cells treated with AGEs-BSA, whereas the HO-1 inhibitor ZnPP IX29 antagonized the effect of sildenafil citrate (Fig. 7). Finally, small interfering RNA approaches were used to confirm further the role of HO-1 in the action of sildenafil citrate. In cells transfected with the HO-1 siRNA, sildenafil citrate failed to inhibit the sFlt-1 release and ROS production (Fig. 8). These results, taken together, strongly suggest that upregulation of the HO-1 expression is the crucial mechanism responsible for the sildenafil citrate-induced inhibition of the sFlt-1 release and ROS production in cells treated with AGEs-BSA.
Figures and Tables
Fig. 1
Upregulated expression and release of soluble fms-like tyrosine kinase-1 (sFlt-1) in JEG-3 cells treated with advanced glycation end-products-vine serum albumin (AGEs-BSA). Cells were incubated in the absence of BSA (Con), in the presence of unmodified BSA (BSA, 200 g/mL) or indicated concentrations of AGEs-BSA (AGE) for 24 h. (A) sFlt-1 mRNA expression was determined by quantitative reverse transcription-polymerase chain reaction (qRT-PCR). (B) sFlt-1 protein release was determined by enzyme-linked immunosorbent assay (ELISA) analysis of the culture supernatants. Mean ± S.E. of 4 independent duplicate experiments. *P < 0.01 vs. BSA control. sFlt-1: soluble fms-like tyrosine kinase-1, AGE: advanced glycation end-products.
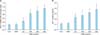
Fig. 2
Effect of anti-receptors for advanced glycation end-product (RAGE) antibody on advanced glycation end-products-bovine serum albumin (AGEs-BSA)-induced stimulation of soluble fms-like tyrosine kinase-1 (sFlt-1) expression and release in JEG-3 cells. Cells were incubated in the presence of each 200 µg/mL of unmodified BSA or AGEs-BSA (AGE) for 24 h. (A) sFlt-1 mRNA expression was determined by quantitative reverse transcription-polymerase chain reaction (qRT-PCR). (B) sFlt-1 protein release was determined by enzyme-linked immunosorbent assay (ELISA) analysis of the culture supernatants. Mean ± S.E. of 4 independent duplicate experiments. *P < 0.01 vs. BSA control, #P < 0.01 vs. without anti-RAGE. sFlt-1: soluble fms-like tyrosine kinase-1, BSA: bovine serum albumin, AGE: advanced glycation end-products.
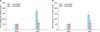
Fig. 3
Effect of anti-receptors for advanced glycation end-product (RAGE) antibody on advanced glycation end-products-bovine serum albumin (AGEs-BSA)-induced stimulation of reactive oxygen species (ROS) production and NF-κB activity in JEG-3 cells. Cells were incubated in the presence of each 200 µg/mL of unmodified BSA or AGEs-BSA (AGE) for 24 h. (A) ROS production was determined by 2',7'-dichlorodihydrofluorescein (DCFH) fluorescence assay. (B) NF-κB activity was determined by reporter gene assay. Mean ± S.E. of 4 independent duplicate experiments. *P < 0.01 vs. BSA control, #P < 0.01 vs. without anti-RAGE. ROS: reactive oxygen species, BSA: bovine serum albumin, AGE: advanced glycation end-products.
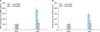
Fig. 4
Effect of sildenafil citrate on advanced glycation end-products-bovine serum albumin (AGEs-BSA)-induced stimulation of soluble fms-like tyrosine kinase-1 (sFlt-1) expression and release in JEG-3 cells. Cells were incubated in the presence of 200 µg/mL AGEs-BSA alone or with indicated concentrations of sildenafil citrate (SC) for 24 h. (A) sFlt-1 mRNA expression was determined by quantitative reverse transcription-polymerase chain reaction (qRT-PCR) and presented as % of BSA control. (B) sFlt-1 protein release was determined by enzyme-linked immunosorbent assay (ELISA) analysis of the culture supernatants and presented as % of BSA control. Mean ± S.E. of 4 independent duplicate experiments. *P < 0.05, #P < 0.01 vs. BSA control. sFlt-1: soluble fms-like tyrosine kinase-1, BSA: bovine serum albumin, SC: sildenafil citrate.
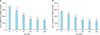
Fig. 5
Effect of sildenafil citrate on advanced glycation end-products-bovine serum albumin (AGEs-BSA)-induced stimulation of reactive oxygen species (ROS) production, NF-κB activity and receptors for advanced glycation end-product (RAGE) mRNA expression in JEG-3 cells. Cells were incubated in the presence of each 200 µg/mL of unmodified BSA or AGEs-BSA (AGE) with or without 20 µM sildenafil citrate (SC) for 24 h. (A) ROS production was determined by 2',7'-dichlorodihydrofluorescein (DCFH) fluorescence assay. (B) NF-κB activity was determined by reporter gene assay. (C) RAGE mRNA expression was determined by quantitative reverse transcription-polymerase chain reaction (qRT-PCR). Mean ± S.E. of 4 independent duplicate experiments. *P < 0.01 vs. BSA control, #P < 0.01 vs. without anti-RAGE. ROS: reactive oxygen species, BSA: bovine serum albumin, AGE: advanced glycation end-products, RAGE: receptors for advanced glycation end-product.
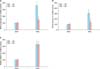
Fig. 6
Upregulation of heme oxygenase-1 (HO-1) expression by sildenafil citrate. Cells were incubated for 24 h in the presence of each 200 µg/mL of unmodified bovine serum albumin (BSA) or advanced glycation end products (AGEs)-BSA (AGE) with vehicle (DMSO, Veh), 20 µM sildenafil citrate (SC) and 20 µM cobalt protoporphyrin (CoPP). (A) HO-1 mRNA expression was determined by quantitative reverse transcription-polymerase chain reaction (qRT-PCR). (B) HO-1 protein expression was determined by Western blot analysis of the cell extracts. Mean ± S.E. of 4 independent duplicate experiments. *P < 0.01 vs. Veh. HO-1: heme oxygenase-1, BSA: bovine serum albumin, AGE: advanced glycation end-product, Veh: vehicle, SC: sildenafil citrate, CoPP: Cobalt protoporphyrin.

Fig. 7
Effects of heme oxygenase-1 (HO-1) related agents on advanced glycation end-products-bovine serum albumin (AGEs-BSA)-stimulated soluble fms-like tyrosine kinase-1 (sFlt-1) release and reactive oxygen species (ROS) production. Cells were incubated for 24 h in the presence of each 200 µg/mL of unmodified BSA or AGEs-BSA (AGE) with or without each 20 µM of sildenafil citrate (SC), cobalt protoporphyrin (CoPP), bilirubin (Bili), and zinc protoporphyrin IX (ZnPP). (A) sFlt-1 protein release was determined by enzyme-linked immunosorbent assay (ELISA) analysis of the culture supernatants and presented as % of BSA control. (B) Reactive oxygen species (ROS) production was determined by 2',7'-dichlorodihydrofluorescein (DCFH) fluorescence assay and presented as % of BSA control. Mean ± S.E. of 3 independent duplicate experiments. *P < 0.01 vs. AGE alone, #P < 0.05 vs. AGE+SC without ZnPP. sFlt-1: soluble fms-like tyrosine kinase-1, BSA: bovine serum albumin, AGE: advanced glycation end-product, SC: sildenafil citrate, CoPP: cobalt protoporphyrin, Bili: bilirubin, ZnPP: zinc protoporphyrin, ROS: reactive oxygen species.
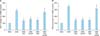
Fig. 8
Effect of heme oxygenase-1 (HO-1) downregulation on advanced glycation end-products-bovine serum albumin (AGEs-BSA)-stimulated soluble fms-like tyrosine kinase-1 (sFlt-1) release and reactive oxygen species (ROS) production. (A) Down-regulation of HO-1 protein expression by siRNA transfection. Cells transfected with 30 picomoles of HO-1 siRNA or scrambled siRNA (siRNA control) were incubated 24 h with vehicle (Veh) or each 20 µM of sildenafil citrate (SC) and cobalt protoporphyrin (CoPP). HO-1 protein expression was determined by Western blot analysis of the cell extracts. (B) Cells were incubated in the presence of each 200 µg/mL of unmodified BSA or AGEs-BSA (AGE) with or without each 20 µM of sildenafil citrate (SC). sFlt-1 protein release was determined by enzyme-linked immunosorbent assay (ELISA) analysis of the culture supernatants. (C) ROS formation was determined by 2',7'-dichlorodihydrofluorescein (DCFH) fluorescence assay. Data were presented as % of BSA control. Mean ± S.E. of 4 independent duplicate experiments. *P < 0.01 vs. BSA control, #P < 0.01 vs. AGE alone, @P < 0.01 vs. siRNA control. HO-1: heme oxygenase-1, Veh: vehicle, SC: sildenafil citrate, CoPP: Cobalt protoporphyrin, sFlt-1: soluble fms-like tyrosine kinase-1, BSA: bovine serum albumin, AGE: advanced glycation end-product, ROS: reactive oxygen species.
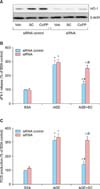
References
1. Roberts JM, Pearson G, Cutler J, Lindheimer M. Summary of the NHLBI working group on research on hypertension during pregnancy. Hypertension. 2003; 41:437–445.
2. Turner JA. Diagnosis and management of pre-eclampsia: an update. Int J Womens Health. 2010; 2:327–337.
3. Wu FT, Stefanini MO, Mac Gabhann F, Kontos CD, Annex BH, Popel AS. A systems biology perspective on sVEGFR1: its biological function, pathogenic role and therapeutic use. J Cell Mol Med. 2010; 14:528–552.
4. Vuorela P, Helske S, Hornig C, Alitalo K, Weich H, Halmesmaki E. Amniotic fluid--soluble vascular endothelial growth factor receptor-1 in preeclampsia. Obstet Gynecol. 2000; 95:353–357.
5. Maynard SE, Min JY, Merchan J, Lim KH, Li J, Mondal S, et al. Excess placental soluble fms-like tyrosine kinase 1 (sFlt1) may contribute to endothelial dysfunction, hypertension, and proteinuria in preeclampsia. J Clin Invest. 2003; 111:649–658.
6. Ahmad S, Ahmed A. Elevated placental soluble vascular endothelial growth factor receptor-1 inhibits angiogenesis in preeclampsia. Circ Res. 2004; 95:884–891.
7. Bergmann A, Ahmad S, Cudmore M, Gruber AD, Wittschen P, Lindenmaier W, et al. Reduction of circulating soluble Flt-1 alleviates preeclampsia-like symptoms in a mouse model. J Cell Mol Med. 2010; 14:1857–1867.
8. Leaños-Miranda A, Campos-Galicia I, Isordia-Salas I, Rivera-Leaños R, Romero-Arauz JF, Ayala-Méndez JA, et al. Changes in circulating concentrations of soluble fms-like tyrosine kinase-1 and placental growth factor measured by automated electrochemiluminescence immunoassays methods are predictors of preeclampsia. J Hypertens. 2012; 30:2173–2181.
9. Brownlee M. Biochemistry and molecular cell biology of diabetic complications. Nature. 2001; 414:813–820.
10. Vlassara H, Striker GE. AGE restriction in diabetes mellitus: a paradigm shift. Nat Rev Endocrinol. 2011; 7:526–539.
11. Gaens KH, Stehouwer CD, Schalkwijk CG. Advanced glycation endproducts and its receptor for advanced glycation endproducts in obesity. Curr Opin Lipidol. 2013; 24:4–11.
12. Piperi C, Adamopoulos C, Dalagiorgou G, Diamanti-Kandarakis E, Papavassiliou AG. Crosstalk between advanced glycation and endoplasmic reticulum stress: emerging therapeutic targeting for metabolic diseases. J Clin Endocrinol Metab. 2012; 97:2231–2242.
13. Toulis KA, Goulis DG, Mintziori G, Kintiraki E, Eukarpidis E, Mouratoglou SA, et al. Meta-analysis of cardiovascular disease risk markers in women with polycystic ovary syndrome. Hum Reprod Update. 2011; 17:741–760.
14. Chekir C, Nakatsuka M, Noguchi S, Konishi H, Kamada Y, Sasaki A, et al. Accumulation of advanced glycation end products in women with preeclampsia: possible involvement of placental oxidative and nitrative stress. Placenta. 2006; 27:225–233.
15. Oliver EA, Buhimschi CS, Dulay AT, Baumbusch MA, Abdel-Razeq SS, Lee SY, et al. Activation of the receptor for advanced glycation end products system in women with severe preeclampsia. J Clin Endocrinol Metab. 2011; 96:689–698.
16. Guedes-Martins L, Matos L, Soares A, Silva E, Almeida H. AGEs, contributors to placental bed vascular changes leading to preeclampsia. Free Radic Res. 2013; 47:Suppl 1. 70–80.
17. Huang QT, Zhang M, Zhong M, Yu YH, Liang WZ, Hang LL, et al. Advanced glycation end products as an upstream molecule triggers ROS-induced sFlt-1 production in extravillous trophoblasts: a novel bridge between oxidative stress and preeclampsia. Placenta. 2013; 34:1177–1182.
18. Ghofrani HA, Osterloh IH, Grimminger F. Sildenafil: from angina to erectile dysfunction to pulmonary hypertension and beyond. Nat Rev Drug Discov. 2006; 5:689–702.
19. Downing J. Sildenafil for the treatment of preeclampsia. Hypertens Pregnancy. 2010; 29:248–250. author reply 51-2.
20. Samangaya RA, Mires G, Shennan A, Skillern L, Howe D, McLeod A, et al. A randomised, double-blinded, placebo-controlled study of the phosphodiesterase type 5 inhibitor sildenafil for the treatment of preeclampsia. Hypertens Pregnancy. 2009; 28:369–382.
21. Kim TH, Lee HH, Kwak JJ. Conservative management of abnormally invasive placenta: choriocarcinoma with uterine arteriovenous fistula from remnant invasive placenta. Acta Obstet Gynecol Scand. 2013; 92:989–990.
22. Ramesar SV, Mackraj I, Gathiram P, Moodley J. Sildenafil citrate improves fetal outcomes in pregnant, L-NAME treated, Sprague-Dawley rats. Eur J Obstet Gynecol Reprod Biol. 2010; 149:22–26.
23. George EM, Palei AC, Dent EA, Granger JP. Sildenafil attenuates placental ischemia-induced hypertension. Am J Physiol Regul Integr Comp Physiol. 2013; 305:R397–R403.
24. Ramesar SV, Mackraj I, Gathiram P, Moodley J. Sildenafil citrate decreases sFlt-1 and sEng in pregnant l-NAME treated Sprague-Dawley rats. Eur J Obstet Gynecol Reprod Biol. 2011; 157:136–140.
25. Orendi K, Kivity V, Sammar M, Grimpel Y, Gonen R, Meiri H, et al. Placental and trophoblastic in vitro models to study preventive and therapeutic agents for preeclampsia. Placenta. 2011; 32:Suppl. S49–S54.
26. Mun MJ, Kim JH, Kim TH, Hwang JY, Jang WC. Associations between estrogen receptor gene polymorphisms and endometriosis. J Korean Soc Menopause. 2013; 19:64–73.
27. Groesch KA, Torry RJ, Wilber AC, Abrams R, Bieniarz A, Guilbert LJ, et al. Nitric oxide generation affects pro- and anti-angiogenic growth factor expression in primary human trophoblast. Placenta. 2011; 32:926–931.
28. Franke S, Siggelkow H, Wolf G, Hein G. Advanced glycation endproducts influence the mRNA expression of RAGE, RANKL and various osteoblastic genes in human osteoblasts. Arch Physiol Biochem. 2007; 113:154–161.
29. Giacco F, Brownlee M. Oxidative stress and diabetic complications. Circ Res. 2010; 107:1058–1070.
30. Yamagishi S, Maeda S, Matsui T, Ueda S, Fukami K, Okuda S. Role of advanced glycation end products (AGEs) and oxidative stress in vascular complications in diabetes. Biochim Biophys Acta. 2012; 1820:663–671.
31. Cudmore M, Ahmad S, Al-Ani B, Fujisawa T, Coxall H, Chudasama K, et al. Negative regulation of soluble Flt-1 and soluble endoglin release by heme oxygenase-1. Circulation. 2007; 115:1789–1797.
32. George EM, Cockrell K, Aranay M, Csongradi E, Stec DE, Granger JP. Induction of heme oxygenase 1 attenuates placental ischemia-induced hypertension. Hypertension. 2011; 57:941–948.
33. George EM, Colson D, Dixon J, Palei AC, Granger JP. Heme oxygenase-1 attenuates hypoxia-induced sFlt-1 and oxidative stress in placental villi through its metabolic products CO and bilirubin. Int J Hypertens. 2012; 2012:486053.
34. Marks GS. Heme oxygenase: the physiological role of one of its metabolites, carbon monoxide and interactions with zinc protoporphyrin, cobalt protoporphyrin and other metalloporphyrins. Cell Mol Biol (Noisy-le-grand). 1994; 40:863–870.
35. Bellamy L, Casas JP, Hingorani AD, Williams DJ. Pre-eclampsia and risk of cardiovascular disease and cancer in later life: systematic review and meta-analysis. BMJ. 2007; 335:974.
36. Zandi-Nejad K, Luyckx VA, Brenner BM. Adult hypertension and kidney disease: the role of fetal programming. Hypertension. 2006; 47:502–508.
37. Schmidt AM, Yan SD, Yan SF, Stern DM. The biology of the receptor for advanced glycation end products and its ligands. Biochim Biophys Acta. 2000; 1498:99–111.
38. Herraiz S, Pellicer B, Serra V, Cauli O, Cortijo J, Felipo V, et al. Sildenafil citrate improves perinatal outcome in fetuses from pre-eclamptic rats. BJOG. 2012; 119:1394–1402.
39. Stanley JL, Andersson IJ, Poudel R, Rueda-Clausen CF, Sibley CP, Davidge ST, et al. Sildenafil citrate rescues fetal growth in the catechol-O-methyl transferase knockout mouse model. Hypertension. 2012; 59:1021–1028.
40. Abram SR, Alexander BT, Bennett WA, Granger JP. Role of neuronal nitric oxide synthase in mediating renal hemodynamic changes during pregnancy. Am J Physiol Regul Integr Comp Physiol. 2001; 281:R1390–R1393.
41. Anumba DO, Robson SC, Boys RJ, Ford GA. Nitric oxide activity in the peripheral vasculature during normotensive and preeclamptic pregnancy. Am J Physiol. 1999; 277:H848–H854.
42. Conrad KP, Joffe GM, Kruszyna H, Kruszyna R, Rochelle LG, Smith RP, et al. Identification of increased nitric oxide biosynthesis during pregnancy in rats. FASEB J. 1993; 7:566–571.
43. Cao J, Inoue K, Li X, Drummond G, Abraham NG. Physiological significance of heme oxygenase in hypertension. Int J Biochem Cell Biol. 2009; 41:1025–1033.
44. Botros FT, Schwartzman ML, Stier CT Jr, Goodman AI, Abraham NG. Increase in heme oxygenase-1 levels ameliorates renovascular hypertension. Kidney Int. 2005; 68:2745–2755.
45. Sabaawy HE, Zhang F, Nguyen X, ElHosseiny A, Nasjletti A, Schwartzman M, et al. Human heme oxygenase-1 gene transfer lowers blood pressure and promotes growth in spontaneously hypertensive rats. Hypertension. 2001; 38:210–215.
46. Yang L, Quan S, Nasjletti A, Laniado-Schwartzman M, Abraham NG. Heme oxygenase-1 gene expression modulates angiotensin II-induced increase in blood pressure. Hypertension. 2004; 43:1221–1226.
47. Neuzil J, Stocker R. Bilirubin attenuates radical-mediated damage to serum albumin. FEBS Lett. 1993; 331:281–284.
48. Neuzil J, Stocker R. Free and albumin-bound bilirubin are efficient co-antioxidants for alpha-tocopherol, inhibiting plasma and low density lipoprotein lipid peroxidation. J Biol Chem. 1994; 269:16712–16719.
49. Wu L, Wang R. Carbon monoxide: endogenous production, physiological functions, and pharmacological applications. Pharmacol Rev. 2005; 57:585–630.