Abstract
Objectives
To examine the effect of diazoxide on hypoxia-induced soluble fms-like tyrosin kinase-1 (sFlt-1) release in JEG-3 choriocarcinoma cells.
Methods
Cells were cultured under normoxia (20% O2) or hypoxia (1% O2), and expression of sFlt-1 mRNA and protein release was determined by quantitative real-time reverse-transcriptase polymerase chain reaction (qRT-PCR) assays and enzyme-linked immunosorbent assay (ELISA).
Results
Tumor necrosis factor-alpha (TNF-α) as well as hypoxia stimulated sFlt-1 release and diazoxide inhibited both of them. The selective inhibitor of mitochondrial adenosine triphosphat (ATP)-sensitive K+ channel opener (KATP) 5-hydroxydecanoate (5-HD) completely reversed the diazoxide-induced inhibition of hypoxia-stimulated sFlt-1 release. qRT-PCR and Western blot analyses showed that diazoxide up-regulated the heme oxygenase-1 (HO-1) expression. In addition, the HO-1 inducer cobalt protoporphyrin (CoPP) and the metabolic product of HO-1 bilirubin mimicked diazoxide to inhibit sFlt-1 release and reactive oxygen species (ROS) production under hypoxia, whereas the HO-1 inhibitor zinc protoporphyrin IX (ZnPP IX) antagonized the effect of diazoxide. In cells transfected with the HO-1 siRNA, diazoxide did not exert any effect on sFlt-1 release and ROS production under hypoxia.
Vascular endothelial growth factor (VEGF) is a key angiogenic factor in both physiological and pathological conditions. VEGF exists in five isoforms, generated by alternate splicing of the mRNA to give proteins of 121, 145, 165, 189 and 206 amino acids in length.1 VEGF exerts its effects by binding with high affinity to two tyrosine kinase receptors VEGFR1/fms-like tyrosine kinase 1 (Flt-1)2 and VEGFR2/kinase domain receptor (KDR).3 The two tyrosine kinase receptors VEGFR1/Flt-1 and VEGFR2/KDR were originally thought to be endothelial cell specific. However, in the placenta both endothelial and non-endothelial cells express the VEGF receptors. Both Flt-1 and KDR are expressed by human placental villous endothelium4,5,6 and Flt-1 mRNA has also been demonstrated to be highly expressed in the cytotrophoblast shell and columns7 while the protein localizes to the trophoblast bilayer and extravillous trophoblast.8
In contrast to the VEGFR2, the VEGFR1 can also be expressed as soluble protein. Soluble Flt-1 (sFlt-1) which comprises the first six immunoglobulin (Ig)-like domains of the protein and is a result of differential splicing of the Flt-1 gene.9 The existence of naturally occurring sFlt-1 was first demonstrated in cultured human umbilical vein endothelial cells.9,10 The trophoblasts also produce large amounts of sFlt11 and a rise in VEGF binding protein was reported in pregnancy.12
Levels of sFlt-1 are elevated in pregnancies complicated with preeclampsia13,14,15 and elevated sFlt-1 generated by preeclamptic placenta is responsible for suppressing angiogenesis.16 Adenoviral delivery of sFlt-1 to pregnant rats mimics the clinical manifestations of preeclampsia15 and introduction of sFlt-1 into pregnant mice caused hypertension and proteinuria, which could be reversed by VEGF.17 The latter study demonstrated that below a critical threshold level, sFlt-1 failed to elicit preeclamptic-like symptoms.17 Thus, a therapy that can reduce the circulating levels of free sFlt-1 in women with preeclampsia might alleviate the clinical signs and symptoms.
Pretreatment with mitochondrial adenosine triphosphat (ATP)-sensitive K+ channel opener (KATP) openers has recently emerged as a promising approach in preserving cellular function in various types of oxidative injuries. The prototype mitochondrial KATP opener diazoxide has been found to reduce infarct size in the whole heart, and survival of cardiomyocytes.18,19 Mitochondrial KATP opener is responsible for effective oxidative phosphorylation20 and regulation of reactive oxygen species (ROS) gene ration21,22 in ischemic preconditioning. Xu et al.23 showed that diazoxide inhibits the production of the proinflammatory cytokines tumor necrosis factor-alpha (TNF-α) and interleukine-6 (IL-6) by preeclamptic placentas and peripheral blood mononuclear cells in normal pregnancy. Recently, Zeng et al.24 have revealed that heme oxygenase-1 (HO-1) can be induced by diazoxide to stimulate protective process that attenuates ischemia/reperfusion injury. ROS and proinflammatory cytokines, TNF-α and IL-6 have been implicated as strong inducers of sFlt-1 release, whereas HO-1 has been reported to attenuate hypoxia-induced sFlt-1 release and oxidative stress.25 This understanding led us to a hypothesis that diazoxide might attenuate hypoxia-induced sFlt-1 release and oxidative stress, and exert a beneficial effect to alleviate preeclamptic signs and symptoms. To this end, we examined the effect of diazoxide on hypoxia-induced sFlt-1 release in JEG-3 human choriocarcinoma cells and the underlying mechanism was deciphered in relation with up-regulated HO-1 expression.
JEG-3 choriocarcinoma cells obtained from American Type Culture Collection were routinely maintained on plastic culture flasks in Dulbecco's minimum essential medium (DMEM)/F12 supplemented with 10% fetal bovine serum (FBS), 50 IU/mL penicillin G and 50 µg/mL streptomycin. Cells were trypsinized when cells became confluent (approximately every 4-5 days) using 0.05% trypsin/0.53 mM ethylenediaminetetraacetic acid (EDTA) solution and reseeded at one-sixth the original density. Between 18 and 24 h before undergoing hypoxia, cells were plated at a density of 1.25 × 105 cells per T-flask (12.5 cm2). The medium was changed 1 h before hypoxic or normoxic treatment to ensure an adequate amount of nutrient and growth factor. Hypoxic treatment was carried out in an incubator supplied with 1% O2, 5% CO2 and 94% N2. Normoxic experiments were performed under 20% O2, 5% CO2 and 75% N2.
Total RNA was extracted using Trizol (Invitrogen, Carlsbad, CA, USA). In order to remove contaminating genomic DNA, 1 mg of RNA was treated with DNase I Amplification Grade (Invitrogen, Carlsbad, CA, USA) at room temperature for 15 min and the reaction was terminated with 25 mM EDTA for 10 min at 65℃. RNA was quantified by spectrometry and used for qRT-PCR. qRT-PCR assays were carried out to quantify sFlt-1 mRNA. Real-time quantitation was based on the LightCycler assay, using a fluorogenic SYBR Green I reaction mixture for PCR with the LightCycler Instrument (Roche Diagnostics, Mannheim, Germany). The PCR reactions were carried out in a total volume of 20 µL, which included SYBR Green I with a Taq DNA polymerase reaction buffer, deoxynucleotide triphosphate mixture, 10 mM magnesium chloride (MgCl2), PCR-grade water, 2 µL of template DNA, and primers. The amplification program consisted of one cycle of 95℃ with 60 s hold (hot start) followed by 45 cycles of 95℃ with 0 s hold, specified annealing temperature with 5 s hold, 72℃ with 12 s hold, and specified acquisition temperature with 2 s hold. All experiments were conducted three times, and negative and positive controls were included. While no template negative control (H2O control) was run with every gene specific primer. Samples were analyzed in duplicate in three independent runs. β-actin mRNA was amplified as an internal control. The primer sequences were as follows:
sFlt-1 protein in cell-conditioned medium was measured by ELISA by using Quantikine Human ELISA kit (R&D Systems, Minneapolis, MN, USA).
Human umbilical vein endothelial cells (HUVECs) were cultured as confluent monolayers in permeable membrane filters. Transendothelial resistance (TER) was measured using transepithelial volt-ohmmeter (EVOM, World Precision Instruments, Sarasota, FL, USA) and chopstick electrodes.
Synthesis of NO was determined by assay of culture supernatants for nitrite, a stable reaction product of NO and molecular oxygen. Briefly, 400 µL of culture supernatant was allowed to react with 200 µL of Griess reagent26 and incubated at room temperature for 15 min. The optical density of the assay samples was measured spectrophotometrically at 570 nm. Fresh culture media served as the blank in all experiments. Nitrite concentrations were calculated from a standard curve derived from the reaction of NaNO2 in the assay mixture.
HO-1 protein was determined by Western blot analysis. Total intracellular protein was extracted by 5 times repeated freeze-thaw (F-T) lysis in F-T buffer (600 mM KCl, 20 mM Tris-Cl, pH 7.8, 20% glycerol, 0.4 mg/mL Pefabloc, 10 µg/mL leupeptin, 10 µg/mL pepstatin, and 5 µg/mL aprotinin). Protein concentration was determined by Bradford assay (Bio-Rad). For western blots, 30 µg of protein was subjected to sodium dodecyl sulphate (SDS)-polyacrylamide gel electrophoresis (PAGE) on 4% SDS-polyacrylamide gels (Bio-Rad). Membranes were blocked with Odyssey blocking buffer (LI-COR, Lincoln, NE, USA) for 2 hr at room temperature. Primary incubation was undertaken overnight at 4℃ with a rabbit anti-HO-1 polyclonal antibody (StressGen, Vancouver, CA, USA) at 1 : 2000 and a mouse anti-β-actin antibody at 1 : 5000. Secondary antibody incubation was done with Alexa Fluor 680 goat anti-rabbit and IRDye 800 goat anti mouse IgG for 1 hr at room temperature. Fluorescence was detected on an Odyssey infrared imager for simultaneous detection of both species. Blot analysis was performed with the supplied Odyssey software, and HO-1 was normalized to β-actin.
The intracellular generation of ROS was measured using 2",7"-dichloro-dihydro-fluorescein diacetate (DCFH-DA). The non fluorescent ester penetrates into the cells and is hydrolyzed to DCFH by the cellular esterases. The probe DCFH is rapidly oxidized to the highly fluorescent compound dichlorofluorescein (DCF) in the presence of cellular peroxidase and ROS such as hydrogen peroxide or fatty acid peroxides. Cells cultured in 24-well plate were preincubated in the culture medium with 10 µM DCFH-DA for 1 hr at 37℃. After the preincubation, cells were exposed to experimental protocols for various durations. Changes in DCF fluorescence was assayed using FACSort Becton Dickinson Flow Cytometer (Becton-Dickinson Bioscience, San Jose, CA, USA) and data were analyzed with CELLQuest Software.
siRNA and scrambled siRNA against human HO-1 were purchased from Santa Cruz Biotechnology (Santa Cruz, CA, USA), and transient transfections were performed using the Superfect® transfection reagent from QIAGEN (Hilden, Germany). The cells were incubated with the siRNA and scrambled siRNA at a concentration of 100 nM for 16 h in serum free media.
Hypoxia and/or inflammatory cytokines contribute as triggers to stimulate sFlt-1 release in preeclampsia placenta.25 We first examined whether the JEG-3 choriocarcinoma cells conserve the basal expression and release of sFlt-1, and responses to hypoxia and the inflammatory cytokine TNF-α.
Quantitative real time PCR and ELISA analyses showed that the JEG-3 cells conserve baseline sFlt-1 mRNA expression and protein release. Cells cultured under hypoxia (1% O2) for 24 h showed 2.1 fold increase in sFlt-1 mRNA expression and 2.7 fold increase in protein release (Fig. 1). Incubation of cells with TNF-α also resulted in elevated sFlt-1 mRNA expression (2.4 fold) and protein release (2.6 fold).
We examined whether hypoxic JEG-3 cell-derived sFlt-1 could lead to endothelial dysfunction by observing the effect of conditioned media on HUVEC functions. HUVEC were grown as confluent monolayers on permeable polycarbonate membrane filters and then switched into conditioned media from normoxic and hypoxic cells. HUVECs incubated in hypoxia-conditioned media showed significantly more rapid drop in TER and carbachol-stimulated NO production than HUVECs incubated in normoxia-conditioned media (Fig. 2).
To determine whether diazoxide affects sFlt-1 release, cells were incubated with diazoxide under normoxia and hypoxia for 24 h and sFlt-1 was assayed in the conditioned media. Diazoxide did not affect the baseline sFlt-1 release, whereas it significantly inhibited the hypoxia-stimulated sFlt-1 release. Likewise, TNF-α-induced sFlt release was significantly reduced upon treatment with diazoxide (Fig. 3).
Diazoxide is a well known selective activator of mitochondrial KATP.27 To determine the involvement of mitochondrial KATP in the action mechanism of diazoxide, we examined the effect of diazoxide in the presence of the selective mitochondrial KATP inhibitor 5-hydroxydecanoate (5-HD).27 As shown in Figure 4, 5-HD completely reversed the diazoxide-induced inhibition of hypoxia-stimulated sFlt-1 release. Interestingly, the selective opener of mitochondrial big conductance Ca2+ activated K+ channel (BKCa), NS 161927 showed similar effect to inhibit the hypoxia-stimulated sFlt-1 release as diazoxide. NS 1619-induced effect was completely reversed in the presence of the mitochondrial BKCa inhibitor paxilline (Fig. 4).27
Several investigators showed that increased expression of HO-1 might inhibit sFlt-1 release and placental damage. Hence, we determined whether diazoxide affects the HO-1 expression. qRT-PCR and Western blot analyses showed that hypoxia exhibited reduction in the HO-1 expression, whereas diazoxide not only reversed the hypoxia-induced reduction but up-regulated further the HO-1 expression (Fig. 5).
In order to determine whether the effect of diazoxide to inhibit sFlt-1 release under hypoxia is related with the promoted expression of HO-1, we determined the effects of HO-1 related molecules on the sFlt-release. The HO-1 inducer cobalt protoporphyrin (CoPP)28 and the metabolic product of HO-1, bilirubin28 mimicked diazoxide to inhibit sFlt-1 release under hypoxia, whereas the HO-1 inhibitor zinc protoporphyrin IX (ZnPP IX)28 attenuated the effect of diazoxide (Fig. 6). Similar results were observed in the experiments to determine the effects of diazoxide and the HO-1 related molecules on hypoxia-stimulated production of ROS (Fig. 7).
Small interfering RNA approaches were used to confirm further the role of HO-1 in the diazoxide-induced inhibition of sFlt-1 release and ROS production. qRT-PCR and Western blot analysis showed that the basal expression of the HO-1 mRNA and protein was significantly diminished by transfection with the HO-1 siRNA. In these cells, CoPP and diazoxide failed to increase the expression of HO-1 mRNA and protein (Fig. 8). In analogy, in cells transfected with the HO-1 siRNA, diazoxide did not exert any effect on sFlt release and ROS production under hypoxia (Fig. 9).
The World Health Organization reports 60,000 maternal deaths worldwide annually as a consequence of preeclampsia and near 12% of their babies fail to thrive within the first month. Preeclampsia is responsible for 30% of all premature deliveries and is associated with approximately 4 million intrauterine growth-restricted babies.29 Moreover, preeclampsia also impacts long term heath. Systematic re view and meta analysis showed that women with preeclampsia are at a two-fold increased risk of developing cardiovascular disease later in life.30 For several decades there has been an active search for novel agents which might have a therapeutic potential for treating preeclampsia. However, still the only effective therapy is delivery of the baby and placenta.
The present study raised the possibility that diazoxide may have the strong potential to be investigated as a the rapeutic agent for preeclampsia. Aside from the findings in the present study, considering that diazoxide has benefits against other pathogenic process that are present in preeclampsia, it might not be absurd to expect beneficial effects from diazoxide to alleviate preeclamptic signs and symptoms. Diazoxide, together with other KATP, has long been used as effective antihypertensive drugs and it may provide beneficial effect for women with preeclampsia to control blood pressure. In addition, diazoxide has been reported to inhibit the production of ROS and proinflammatory cytokines such as TNF-α and IL-6 by preeclamptic placentas and peripheral blood mononuclear cells.23 Those proinflammatory cytokines have been implicated as important mediators to initiate or aggravate preeclampsia.25
In the present study, qRT-PCR and ELISA analyses showed that exposure to hypoxia or proinflammatory cytokine TNF-α stimulated sFlt-1 mRNA expression and protein release (Fig. 1). Diazoxide did not affect the baseline sFlt-1 release, whereas it significantly inhibited both the hypoxiaand TNF-α-stimulated sFlt-1 mRNA expression and protein release (Fig. 3). In preeclampsia, poor placentation causes both oxidative and endoplasmic reticulum stress of the placenta. It is believed placental hypoxia stimulates excessive production of sFlt-1, which binds and deactivates circulating VEGF. When maternal endothelium is deprived of VEGF it becomes dysfunctional hence leading to the clinical syndrome of the mother. Although hypoxia is one trigger for release of sFlt-1 from the preeclamptic placenta, inflammatory mechanisms may contribute or even predominate.25 Stimulation of sFlt-1 expression and release by TNF-α which is comparable with hypoxia-induced stimulation in the present study is consistent with this argument (Fig. 3). The ability of diazoxide to inhibit both the hypoxia- and TNF-α-induced sFlt-1 release reminded us of the fact that it had already been known as a promising approach to preserve cellular function in various types of oxidative injuries.
Though diazoxide is a selective activator of mitochondrial KATP, several mitochondrial K+ channel-independent effects of diazoxide should be considered. Diazoxide inhibits succinate oxidation31 and succinate dehydrogenase32 or inhibit opening of the mitochondrial membrane pore33 and these may be responsible for its effects. However, in the present study, the selective inhibitor of mitochondrial KATP 5-HD27 completely reversed the diazoxide-induced inhibition of hypoxia-stimulated sFlt-1 release. Furthermore, the selective opener of mitochondrial BKCa NS 161927 showed similar effect to inhibit the hypoxia-stimulated sFlt-1 release as diazoxide. NS 1619-induced effect was completely reversed in the presence of the mitochondrial BKCa in hibitor paxilline.27 These results suggest that opening of mitochondrial K+ channels is a crucial process in diazoxide-induced inhibition of sFlt release (Fig. 4).
One of the potential therapy which has been proven effective in numerous experimental forms of hypertension is the manipulation of the HO-1 pathway.34,35,36,37 HO-1 is hypothesized to attenuate hypertension through multiple pathways. HO-1 produces two bioactive compounds as products of heme metabolism: carbon monoxide (CO) and bilirubin. Bilirubin has been shown in numerous systems to function as a powerful antioxidant.38,39 As it is recognized that production of ROS is a major component of varied forms of hypertension, moderate increases in bilirubin could act to decrease overall oxidative stress. CO acts as a potent vasodilator, functioning in a manner similar to endothelium-derived relaxing factor.40
Of particular interest to preeclampsia, HO-1 and CO have been shown to directly inhibit production of endoglin and interferon-γ-induced secretion of sFlt-1.41 Additionally, it has been reported that CO derived from HO-1 in vascular smooth muscle could inhibit production of endothelin-1,42 a protein shown to be a final common pathological factor in several experimental models of preeclampsia.
Several lines of evidence suggested the involvement of HO-1 in diazoxide-induced inhibition of sFlt-1 release and ROS formation. qRT-PCR and Western blot analyses showed that hypoxia exhibited reduction in the HO-1 expression, whereas diazoxide not only reversed the hypoxia-induced reduction but further up-regulated HO-1 expression (Fig. 5). In addition, the HO-1 inducer CoPP28 and the metabolic product of HO-1 bilirubin28 mimicked diazoxide to inhibit sFlt-1 release and ROS production under hypoxia, whereas the HO-1 inhibitor ZnPP IX28 antagonized the effect of diazoxide (Fig. 6, 7). Finally, a small interfering RNA app roach was used to confirm further the role of HO-1 in the diazoxide-induced inhibition of sFlt-1 release and ROS production. In cells transfected with the HO-1 siRNA, diazoxide did not exert any effect on sFlt-1 release and ROS production under hypoxia (Fig. 8,9). These results, taken together, strongly suggest that up-regulation of the HO-1 expression is the crucial mechanism responsible for the diazoxide-induced inhibition of the sFlt-1 release and ROS production under hypoxia.
Figures and Tables
Fig. 1
Upregulated expression of soluble fms-like tyrosine kinase 1 (sFlt-1) in response to hypoxia and tumor necrosis factor alpha (TNF-α) in JEG-3 cells. Cells were cultured under normoxia (20% O2), under hypoxia (1% O2), or under normoxia with TNF-α (50 ng/mL) for 24 h. (A) sFlt-1 mRNA expression was determined by quantitative real-time reverse-transcriptase polymerase chain reaction (qRT-PCR). (B) sFlt-1 protein release was determined by enzyme-linked immunosorbent assay (ELISA) analysis of the culture supernatants. Mean ± SE of six independent duplicate experiments. *P < 0.01 vs. normoxic control. (sFlt-1: soluble fms-like tyrosine kinase 1, TNF-α: tumor necrosis factor alpha)

Fig. 2
Deterioration of functional integrity of human umbilical vein endothelial cell (HUVEC) by conditioned media from hypoxic JEG-3 cells. HUVECs were grown as confluent monolayers on permeable polycarbonate membrane filters and then exposed to conditioned media from JEG-3 cells cultured for 24 h under normoxia (20% O2, open circle in A, normoxia control [NC] in B) or hypoxia (1% O2, solid circle in A, hyperoxia control [HC] group in B). After indicated time periods (in A) or 24 h further incubation (in B), changes in transendothelial resistance (TER) (A) and nitrite production (B) were determined. Mean ± SE of four independent duplicate experiments. *P < 0.01 vs. normoxic conditioned media. (TER: transendothelial resistance, NC: normoxia control, HC: hyperoxia control)
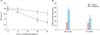
Fig. 3
Diazoxide-induced inhibition of hyoxia- and tumor necrosis factor alpha (TNF-α)-stimulated soluble fms-like tyrosine kinase 1 (sFlt-1) release. Cells were cultured under normoxia (20% O2), hypoxia (1% O2), or under normoxia with TNF-α (50 ng/mL) in the presence and absence of diazoxide (10 µM) for 24 h. (A) sFlt-1 mRNA expression was determined by quantitative real-time reverse-transcriptase polymerase chain reaction (qRT-PCR). (B) sFlt-1 protein release was determined by enzyme-linked immunosorbent assay (ELISA) analysis of the culture supernatants. Mean ± SE of four independent duplicate experiments. *P < 0.01 vs. normoxic control. #P < 0.05 vs without diazoxide. (TER: transendothelial resistance, NC: normoxia control, HC: hyperoxia control)

Fig. 4
Effects of mitochondrial K+ channel openers and their inhibitors on soluble fms-like tyrosine kinase 1 (sFlt-1) release. Cells were cultured under normoxia (20% O2) or hypoxia (1% O2) with each 10 µM of diazoxide or NS 1619 in the presence and absence of their selective inhibitors, 5-hydroxydecanoate (5-HD) and paxilline (each 10 µM). sFlt-1 protein release was determined by enzyme-linked immunosorbent assay (ELISA) analysis of the culture supernatants and presented as % of normoxic control. Mean ± SE of four independent duplicate experiments. *P < 0.01 vs. the respective control. #P < 0.05 vs. vehicle (without inhibitors). (sFlt-1: soluble fms-like tyrosine kinase 1, 5-HD: 5-hydroxydecanoate)

Fig. 5
Up-regulation of heme oxygenase-1 (HO-1) expression by diazoxide. Cells were cultured under normoxia (20% O2) or hypoxia (1% O2) with or without diazoxide (10 µM) and cobalt protoporphyrin (CoPP; 10 µM) as a positive control for 24 h. (A) HO-1 mRNA expression was determined by quantitative real-time reverse-transcriptase polymerase chain reaction (qRT-PCR). (B) HO-1 protein expression was determined by Western blot analysis of the cell extracts. Mean ± SE of four independent duplicate experiments. *P < 0.01 vs. normoxia. #P < 0.01 vs. the respective control. (HO-1: heme oxygenase-1, NO : nitric oxide, CoPP: cobalt protoporphyrin)

Fig. 6
Effects of heme oxygenase-1 (HO-1) related agents on soluble fms-like tyrosine kinase 1 (sFlt-1) release. Cells were cultured under normoxia (20% O2) or hypoxia (1% O2) with or without 10 µM of each agent. sFlt-1 protein release was determined by enzyme-linked immunosorbent assay (ELISA) analysis of the culture supernatants and presented as % of normoxic control. Mean ± SE of three independent duplicate experiments. *P < 0.01 vs. control. #P < 0.05 vs. diazoxide alone. (sFlt-1: soluble fmslike tyrosine kinase 1, CoPP: cobalt protopor phyrin, ZnPP: zinc protoporphyrin)

Fig. 7
Effects of heme oxygenase-1 (HO-1) related agents on reactive oxygen species (ROS) production. Cells were cultured under normoxia (20% O2) or hypoxia (1% O2) with or without 10 µM of each agents. ROS formation was determined by fluorospectrometric analysis of dichlorofluorescein (DCF) fluorescence and presented as % of normoxic control. Mean ± SE of three independent duplicate experiments. *P < 0.01 vs. control. #P < 0.05 vs diazoxide alone. (ROS: reactive oxygen species, CoPP: cobalt protoporphyrin, ZnPP: zinc protoporphyrin)
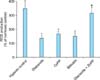
Fig. 8
Down-regulation of heme oxygenase-1 (HO-1) mRNA and protein expression by siRNA transfection. Cells were transfected with HO-1 siRNA or scrambled siRNA (siRNA control). (A) HO-1 mRNA expression was determined by quantitative real-time reverse-transcriptase polymerase chain reaction (qRT-PCR). (B) HO-a protein expression was determined by Western blot analysis of the cell extracts. *P < 0.01 vs. siRNA control. #P < 0.05 vs. the respective control (without drug). (HO-1: heme oxygenase-1, CoPP: cobalt protoporphyrin, Cont: Control, Diaz: Diazoxide)
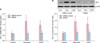
Fig. 9
Suppression of the diazoxide- and cobalt protoporphyrin (CoPP)-induced effects on soluble fms-like tyrosine kinase 1 (sFlt) release and reactive oxygen species (ROS) formation in heme oxygenase-1 (HO-1) siRNA-transfected cells. Cells were transfected with 30 pi comoles of HO-1 siRNA and incubated for 24 h normoxia (20% O2) or hypoxia (1% O2) with or without each 10 µM of with each 10 µM of diazoxide or CoPP. (A) sFlt-1 protein release was determined by enzyme-linked immunosorbent assay (ELISA) analysis of the culture supernatants. (B) ROS formation was determined by a fluorospectrometric analysis of dichlorofluorescein (DCF) fluorescence. Data were presented as % of normoxic control. Mean ± SE of four independent duplicate experiments. (sFlt-1: soluble fms-like tyrosine kinase 1, CoPP: cobalt protoporphyrin, ROS: reactive oxygen species)
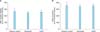
References
1. Dvorak HF, Brown LF, Detmar M, Dvorak AM. Vascular permeability factor/vascular endothelial growth factor, microvascular hyperpermeability, and angiogenesis. Am J Pathol. 1995; 146:1029–1039.
2. de Vries C, Escobedo JA, Ueno H, Houck K, Ferrara N, Williams LT. The fms-like tyrosine kinase, a receptor for vascular endothelial growth factor. Science. 1992; 255:989–991.
3. Terman BI, Dougher-Vermazen M, Carrion ME, Dimitrov D, Armellino DC, Gospodarowicz D, et al. Identification of the KDR tyrosine kinase as a receptor for vascular endothelial cell growth factor. Biochem Biophys Res Commun. 1992; 187:1579–1586.
4. Crescimanno C, Marzioni D, Persico MG, Vuckovic M, Mühlhauser J, Castellucci M. Expression of bFGF, PIGF and their receptors in the human placenta. Placenta. 1995; 16:A13.
5. Clark DE, Smith SK, Sharkey AM, Charnock-Jones DS. Localization of VEGF and expression of its receptors flt and KDR in human placenta throughout pregnancy. Hum Reprod. 1996; 11:1090–1098.
6. Vuckovic M, Ponting J, Terman BI, Niketic V, Seif MW, Kumar S. Expression of the vascular endothelial growth factor receptor, KDR, in human placenta. J Anat. 1996; 188(Pt 2):361–366.
7. Charnock-Jones DS, Sharkey AM, Boocock CA, Ahmed A, Plevin R, Ferrara N, et al. Vascular endothelial growth factor receptor localization and activation in human trophoblast and choriocarcinoma cells. Biol Reprod. 1994; 51:524–530.
8. Ahmed A, Li XF, Dunk C, Whittle MJ, Rushton DI, Rollason T. Colocalisation of vascular endothelial growth factor and its Flt-1 receptor in human placenta. Growth Factors. 1995; 12:235–243.
9. Villars DS. The equilibrium constants of reactions involving hydroxyl. Proc Natl Acad Sci U S A. 1929; 15:705–709.
10. Kendall RL, Wang G, Thomas KA. Identification of a natural soluble form of the vascular endothelial growth factor receptor, FLT-1, and its heterodimerization with KDR. Biochem Biophys Res Commun. 1996; 226:324–328.
11. Clark DE, Smith SK, He Y, Day KA, Licence DR, Corps AN, et al. A vascular endothelial growth factor antagonist is produced by the human placenta and released into the maternal circulation. Biol Reprod. 1998; 59:1540–1548.
12. Anthony FW, Evans PW, Wheeler T, Wood PJ. Variation in detection of VEGF in maternal serum by immunoassay and the possible influence of binding proteins. Ann Clin Biochem. 1997; 34(Pt 3):276–280.
13. Ahmad S, Ahmed A. Regulation of soluble VEGFR-1 by VEGF and oxygen and its elevation in pre-eclampsia and fetal growth restriction. Placenta. 2001; 22:A7.
14. Vuorela P, Helske S, Hornig C, Alitalo K, Weich H, Halmesmäki E. Amniotic fluid--soluble vascular endothelial growth factor receptor-1 in preeclampsia. Obstet Gynecol. 2000; 95:353–357.
15. Maynard SE, Min JY, Merchan J, Lim KH, Li J, Mondal S, et al. Excess placental soluble fms-like tyrosine kinase 1 (sFlt1) may contribute to endothelial dysfunction, hypertension, and proteinuria in preeclampsia. J Clin Invest. 2003; 111:649–658.
16. Ahmad S, Ahmed A. Elevated placental soluble vascular endothelial growth factor receptor-1 inhibits angiogenesis in preeclampsia. Circ Res. 2004; 95:884–891.
17. Bergmann A, Ahmad S, Cudmore M, Gruber AD, Wittschen P, Lindenmaier W, et al. Reduction of circulating soluble Flt-1 alleviates preeclampsia-like symptoms in a mouse model. J Cell Mol Med. 2010; 14:1857–1867.
18. Kersten JR, Gross GJ, Pagel PS, Warltier DC. Activation of adenosine triphosphate-regulated potassium channels: mediation of cellular and organ protection. Anesthesiology. 1998; 88:495–513.
19. Szewczyk A, Marbán E. Mitochondria: a new target for K channel openers? Trends Pharmacol Sci. 1999; 20:157–161.
20. Dos Santos P, Kowaltowski AJ, Laclau MN, Seetharaman S, Paucek P, Boudina S, et al. Mechanisms by which opening the mitochondrial ATP-sensitive K(+) channel protects the ischemic heart. Am J Physiol Heart Circ Physiol. 2002; 283:H284–H295.
21. Krenz M, Oldenburg O, Wimpee H, Cohen MV, Garlid KD, Critz SD, et al. Opening of ATP-sensitive potassium channels causes generation of free radicals in vascular smooth muscle cells. Basic Res Cardiol. 2002; 97:365–373.
22. Ferranti R, da Silva MM, Kowaltowski AJ. Mitochondrial ATP-sensitive K+ channel opening decreases reactive oxygen species generation. FEBS Lett. 2003; 536:51–55.
23. Xu B, Makris A, Thornton C, Ogle R, Horvath JS, Hennessy A. Antihypertensive drugs clonidine, diazoxide, hydralazine and furosemide regulate the production of cytokines by placentas and peripheral blood mononuclear cells in normal pregnancy. J Hypertens. 2006; 24:915–922.
24. Zeng Z, Huang HF, He F, Wu LX, Lin J, Chen MQ. Diazoxide attenuates ischemia/reperfusion injury via upregulation of heme oxygenase-1 after liver transplantation in rats. World J Gastroenterol. 2012; 18:1765–1772.
25. Redman CW, Sargent IL. Placental stress and preeclampsia: a revised view. Placenta. 2009; 30:Suppl A. S38–S42.
26. Schmidt HHHW, Kelm M. Determination of nitrite and nitrate by the Griess reaction. In : Feelisch M, Stamler JS, editors. Methods in nitric oxide research. Chichester: John Wiley & Sons;1996. p. 491–497.
27. Robertson DW, Steinberg MI. Potassium channel modulators: scientific applications and therapeutic promise. J Med Chem. 1990; 33:1529–1541.
28. Marks GS. Heme oxygenase: the physiological role of one of its metabolites, carbon monoxide and interactions with zinc protoporphyrin, cobalt protoporphyrin and other metalloporphyrins. Cell Mol Biol (Noisy-le-grand). 1994; 40:863–870.
29. Cudmore MJ, Ramma W, Cai M, Fujisawa T, Ahmad S, Al-Ani B, et al. Resveratrol inhibits the release of soluble fmslike tyrosine kinase (sFlt-1) from human placenta. Am J Obstet Gynecol. 2012; 206:253.e10–253.e15.
30. Bellamy L, Casas JP, Hingorani AD, Williams DJ. Preeclampsia and risk of cardiovascular disease and cancer in later life: systematic review and meta-analysis. BMJ. 2007; 335:974.
31. Drose S, Brandt U, Hanley PJ. K+-independent actions of diazoxide question the role of inner membrane KATP channels in mitochondrial cytoprotective signaling. J Biol Chem. 2006; 281:23733–23739.
32. Dzeja PP, Bast P, Ozcan C, Valverde A, Holmuhamedov EL, Van Wylen DG, et al. Targeting nucleotide-requiring enzymes: implications for diazoxide-induced cardioprotection. Am J Physiol Heart Circ Physiol. 2003; 284:H1048–H1056.
33. Hausenloy D, Wynne A, Duchen M, Yellon D. Transient mitochondrial permeability transition pore opening mediates preconditioning-induced protection. Circulation. 2004; 109:1714–1717.
34. Cao J, Inoue K, Li X, Drummond G, Abraham NG. Physiological significance of heme oxygenase in hypertension. Int J Biochem Cell Biol. 2009; 41:1025–1033.
35. Botros FT, Schwartzman ML, Stier CT Jr, Goodman AI, Abraham NG. Increase in heme oxygenase-1 levels ameliorates renovascular hypertension. Kidney Int. 2005; 68:2745–2755.
36. Sabaawy HE, Zhang F, Nguyen X, ElHosseiny A, Nasjletti A, Schwartzman M, et al. Human heme oxygenase-1 gene transfer lowers blood pressure and promotes growth in spontaneously hypertensive rats. Hypertension. 2001; 38:210–215.
37. Yang L, Quan S, Nasjletti A, Laniado-Schwartzman M, Abraham NG. Heme oxygenase-1 gene expression modulates angiotensin II-induced increase in blood pressure. Hypertension. 2004; 43:1221–1226.
38. Neuzil J, Stocker R. Bilirubin attenuates radical-mediated damage to serum albumin. FEBS Lett. 1993; 331:281–284.
39. Neuzil J, Stocker R. Free and albumin-bound bilirubin are efficient co-antioxidants for alpha-tocopherol, inhibiting plasma and low density lipoprotein lipid peroxidation. J Biol Chem. 1994; 269:16712–16719.
40. Wu L, Wang R. Carbon monoxide: endogenous production, physiological functions, and pharmacological applications. Pharmacol Rev. 2005; 57:585–630.
41. Cudmore M, Ahmad S, Al-Ani B, Fujisawa T, Coxall H, Chudasama K, et al. Negative regulation of soluble Flt-1 and soluble endoglin release by heme oxygenase-1. Circulation. 2007; 115:1789–1797.
42. Morita T, Kourembanas S. Endothelial cell expression of vasoconstrictors and growth factors is regulated by smooth muscle cell-derived carbon monoxide. J Clin Invest. 1995; 96:2676–2682.