Abstract
Background
Hereditary spherocytosis (HS) is a chronic hemolytic anemia characterized by microspherocytes in the peripheral blood and increased erythrocyte osmotic fragility (EOF). This study evaluated the cryohemolysis test (CHT); initial hemolysis (IH); immediate and incubated hemolysis percentage in 5.5 g/L NaCl (H5.5); mean corpuscular hemoglobin concentration (MCHC); red blood cell distribution width (RDW); and Hb/MCHC, Hb/RDW, and MCHC/RDW ratios for the diagnosis of HS.
Methods
Data from 13 patients with HS were evaluated at the Instituto de Bioquímica Aplicada and compared with data from 14 unaffected individuals and 11 patients with anemia due to another etiology. Total blood and reticulocyte counts, CHT, and immediate and incubated EOF were performed in all subjects; sensitivity, specificity, efficiency, and Youden index (YI) were calculated.
Results
Eight patients with HS had MCHC ≥345 g/L, 10 had RDW ≥14.5%, 12 had IH >5.0 g/L, 11 had immediate H5.5 ≥5%, and 13 had incubated H5.5 ≥50% (the cut-off value to consider HS). The efficiency and YI were: immediate H5.5 (0.94–0.85), incubated H5.5 (0.89–0.82), IH (0.89–0.78), MCHC (0.87–0.62), CHT (0.84–0.54), and Hb/MCHC (0.71–0.56), respectively. The calculated ratios could distinguish subjects with HS from unaffected individuals (P<0.05), but not those with anemia of another etiology (P>0.05).
Conclusion
Although the CHT and supplementary hematimetric indexes were useful to differentiate individuals with SH from healthy controls, they cannot distinguish from anemias of other etiology. CHT and MCHC, in addition to EOF, are recommended for diagnosing HS patients because of their low cost and efficiency.
Hereditary spherocytosis (HS) is a heterogeneous disorder in which abnormalities of the red blood cell membrane structural proteins lead to the loss of membrane surface area, resulting in hyperdense, spherically-shaped erythrocytes with reduced deformability and reduced shorter half-life. The loss of surface area results from increased membrane fragility due to primary and secondary abnormalities in erythrocyte membrane proteins involved in the vertical joints between the cytoskeleton and the lipid bilayer, particularly ankyrin, α- and β-spectrin, band 3, and protein 4.2 [12].
HS is inherited in an autosomal dominant manner in around two-thirds of patients, associated with mutations in the ankyrin, β-spectrin, or band 3 genes. In the remaining patients, inheritance is non-dominant due to autosomal recessive inheritance or a de novo mutation [3].
The osmotically fragile spherocytes are selectively trapped in the spleen and destroyed. Increased red blood cell destruction causes the main clinical features of typical HS: evidence of hemolysis with anemia, reticulocytosis, splenomegaly, jaundice, gallstone formation, spherocytes in a peripheral blood smear, and increased osmotic fragility of the erythrocytes. The degree of hemolysis varies from asymptomatic patients who are incidentally diagnosed, to patients with severe disease, who are transfusion-dependent [4].
When investigating a patient suspected to have a membrane-associated hemolytic anemia, a range of information is required, including family and clinical history, red cell indices and peripheral blood smear examination, reticulocyte count and negative direct Coombs test (DCT) findings, and the results of laboratory tests for hemolytic processes and membrane defects [5].
The principle for the traditional laboratory diagnostic tests of hereditary red cell membrane defects exploits the reduced surface area-to-volume ratio, as found in spherocytes. These tests measure the rate of red cell lysis in different incubation media. The erythrocyte osmotic fragility (EOF) test determines the concentration of sodium chloride at which 50% of the fresh and incubated red cells are lysed. The glycerol lysis, acidified glycerol lysis time test (AGLT), and pink tests determine the extent or rate of the lysis of red cells suspended in buffered glycerol solutions. However, these tests do not differentiate HS from secondary spherocytosis associated with other conditions, mainly autoimmune hemolytic anemias [6].
Streichman et al. [7] proposed in 1990 the cryohemolysis test (CHT) to identify HS, based on the observation that red blood cells of HS patients are particularly susceptible to cold (0℃) in hypertonic conditions. Later, King et al. [8] presented a rapid flow cytometric test for the diagnosis of HS in routine hematology. The flow cytometric test measures the fluorescence intensity of intact red cells labeled with eosin-5-maleimide (EMA) dye, which reacts covalently with Lys-430 on the first extracellular loop of band 3 protein. Finally, Won and Suh [9] developed the flow cytometry based OF (FCM OF) test to measure the residual red cell counts after the cells are exposed to hypotonic solutions.
British experts in 2011 recommended the implementation of cryohemolysis and EMA when the HS diagnosis was equivocal because of its high predictive value. However, in patients with family history, clinical and typical laboratory (spherocytes, increased mean corpuscular hemoglobin concentration [MCHC], and reticulocytosis) findings indicated that it was not necessary to perform additional diagnostic tests. Finally, gel electrophoresis analysis of erythrocyte membranes was the method of choice for the diagnosis of atypical cases [10].
The International Council for Standardization in Haematology reported that all screening tests; i.e., the EOF test, AGLT test, osmotic gradient ektacytometry, and EMA binding test, can detect typical HS. In the absence of a family history, DCT and one screening test for HS should both be performed. If in doubt, they have advised the use of two of these screening tests [11].
Some authors have explored the discriminant capacity of hematimetric indices such as MCHC and red blood cell distribution width (RDW) [12], as well as ratios calculated from them (Hb/MCHC, Hb/RDW, and MCHC/RDW) [13]. These authors concluded that these indices were valuable complementary tools in the diagnosis and classification of disease severity.
The sensitivity and specificity of these tests vary according to different authors. The objective of this study was to determine the efficiency in HS diagnosis of the CHT; EOF parameters (immediate and post-incubation hemolysis % in 5.5 g/L NaCl and initial hemolysis); and the MCHC and RDW hematimetric indexes and their calculated ratios (Hb/MCHC, Hb/RDW, and MCHC/RDW).
A cross-sectional descriptive study was performed between November 2012 and March 2017 at the Instituto de Bioquímica Aplicada, Universidad Nacional de Tucumán (Argentina). The sample consisted of 38 individuals. Of these, 14 were healthy controls, 13 had HS (4 adults and 9 children ≤12 yr of age), and 11 had anemias due to other causes (4β-thalassemia trait carriers, 2 HbS heterozygous, 2 iron deficiency anemia [IDA], 2 non-spherocytic hemolytic anemia, and 1 microcytic hypochromic anemia with normal iron level). The diagnosis of HS was based on signs and symptoms of hemolytic anemia plus osmotic fragility measurements in fresh and incubated blood, as well as the presence of spherocytes in peripheral blood smears. Thalassemia patients were diagnosed on the basis of cell indices, cellulose acetate electrophoresis (CAE) at alkaline pH, and Hb A2 level by microcolumn chromatography (BioSystem S.A., Barcelona, Spain). Sickle cell hemoglobinopathies were also diagnosed on the basis of CAE and sickling test results. IDA was diagnosed by determining serum iron concentration, total iron binding capacity (TIBC), and transferrin saturation (SAT) through colorimetric methods (Wiener Lab, Rosario, Argentina). Subjects with non-spherocytic hemolytic anemia, according reticulocytes, bilirubin and lactate dehydrogenase (LDH) levels had negative direct Coombs test (DCT) findings.
Blood samples were collected in K2-EDTA. The total blood count was measured by hematologic counter (Sysmex KX-21N, Kobe, Japan), reticulocytes were counted manually with brilliant cresyl blue dye, and DCT and cryohemolysis test (CHT) were also performed. Using the Hb measurement and hematimetric indexes, the Hb/MCHC, Hb/RDW, and MCHC/RDW ratios were calculated. MCHC ≥345 g/L and RDW ≥14.5% were considered indicative of HS.
Bilirubin and LDH levels were measured using an autoanalyzer (Roche Cobas c311, Indianapolis, USA) in patients with a presumptive HS diagnosis. The reference values for LDH are 200–480 U/L, for total bilirubin (TBil) are up to 1.2 mg/dL and indirect bilirubin (IBil) to 0.8 mg/dL.
The method described by Streichman et al. [71415] was used to perform the CHT. The reagent occurs in a 0.7 M buffered sucrose solution. The sample (500 µL) was placed into plastic tubes and washed 3 times with cold saline. A 60% cell suspension in saline was prepared and kept in an ice bath until testing. Two milliliters of the buffered sucrose solution were incubated at 37℃ for 10 minutes to equilibrate the temperature. Fifty µL of cell suspension was placed in the preheated sucrose solution, mixed manually for several seconds, and incubated at 37℃ for exactly 10 minutes. Thereafter, the tubes were transferred to an ice bath, incubated for exactly 10 minutes, and centrifuged at 1500 rpm for another 10 minutes. The supernatant was read at 540 nm (Metrolab 1600 plus, Rosario, Argentina). The test was performed twice in each subject and processed in parallel with a normal control. A hemolysate solution at 100% was prepared by pipetting 50 µL of the original sample in 2 mL of distilled water. It was centrifuged and 200 µL of supernatant was placed in 4 mL of distilled water. The solution was mixed and read at 540 nm. To calculate the percentage of cryohemolysis, the optical density (OD) of the sample and 100% hemolysate was used according to the following formula:
The normal range suggested in the literature is 3–15%, with CHT >20% suggestive of HS [716]. Previously, in order to corroborate the normal range, our team studied 30 healthy volunteers, finding a range of 4.0–12.5% [17]. As these results were similar to those in the literature, we adopted the recommended reference values.
Anticoagulated whole blood with lithium heparin was used for the EOF test, as described by Dacie and Lewis [16]. The samples were processed in parallel with a normal control. EOF was expressed as the concentration of NaCl required to produce 50% hemolysis (H50). The same procedure was performed after the samples were kept at 37℃ for 24 hours (incubated test). The reference values for immediate and incubated EOF were 4.0–4.5 g/L and 4.6–5.9 g/L, respectively. It was considered indicative of HS, when 5.5 g/L NaCl hemolysis tube showed a value ≥5% and ≥50% in the immediate and incubated test, respectively. Another index for HS was immediate initial hemolysis ≥5.0 g/L NaCl in the EOF test.
This study was approved by Comité de Bioética de la Facultad de Medicina (Universidad Nacional de Tucumán), Tucumán, Argentina. Informed consent was obtained from all patients before inclusion in the study. In the case of children, the consent was provided by their parents.
IBM SPSS Statistics for Windows, version 20.0 was used to perform out all statistical analyses. All data except for age were presented as mean±standard deviation. Student's-T tests were used for pairwise comparisons between groups. P<0.05 was defined as the level of significance. The sensitivity (Se) and specificity (Sp) of the tests for HS were calculated; receiver operating characteristic (ROC) curves were drawn and areas under the curve (AUC) were calculated. Efficiency and Youden index (YI) were calculated using the following formula:
Table 1 shows the results for the three groups under study: normal, HS and other anemia. The subjects with HS were children under 12 years of age in 9 cases; eight had a family history of hemolytic anemia, seven had been transfused at least once, and one had been splenectomized. Spherocytes were observed in all individuals with HS but also were seen in two patients with iron deficiency anemia and in one with β-thalassemia trait. Significant differences (P<0.05) in almost all parameters were observed between healthy controls and HS individuals. The same was observed when comparing the normal group to those with other anemia, except for CHT, reticulocytes, initial hemolysis, and incubated 5.5 g/L NaCl hemolysis % (P>0.05).
CHT in the healthy control group showed a mean of 7.0±3.9%; only one subject had a value of 18.9%, above the reference range (3–15%) but lower for considering HS (>20%). Two children, one with heterozygous sickle cell anemia and one with β-thalassemia trait, showed CHT values of 35.7% and 32.0%, respectively (Fig. 1). For β-thalassemia carriers, the CHT levels were 15.3±11.6%. According to the ROC curve analysis, the optimum cutoff value to separate normal subjects from patients with HS was 15%. In these conditions, the computed test sensitivity and specificity were 83% and 88%, respectively (Fig. 2).
Comparison between the HS and other anemia groups revealed significant differences (P<0.05) in mean corpuscular Hb (MCH), MCHC, reticulocytes, CHT, immediate and incubated H50, initial hemolysis, and immediate and incubated 5.5 g/L NaCl hemolysis % (Table 1).
The HS group had LDH, total bilirubin (TBil) and indirect bilirubin (IBil) levels above the reference values (LDH=606±173 U/L; TBil=2.63±1.10 mg/dL; IBil=2.02±1.08 mg/dL). Eight HS patients had MCHC ≥345 g/L, and 10 had RDW ≥14.5%. One child with heterozygous sickle cell anemia exhibited a very high RDW (Fig. 1). Twelve HS subjects presented with an initial hemolysis ≥5.0 g/L; 11 showed immediate hemolysis at NaCl 5.5 g/L ≥5%; and the incubated hemolysis at NaCl 5.5 g/L was ≥50% in the HS group. Reticulocyte counts also revealed the presence of Heinz bodies in three individuals with HS and in one with sickle cell hemoglobinopathy.
The ROC curves for MCHC and RDW, with AUC of 0.91 and 0.84, respectively, demonstrated that the best cutoff values were used in the actual study (Fig. 2). The combination of MCHC and RDW as a screening tool increased the Youden index values (Table 2).
The Hb/MCHC, Hb/RDW, and MCHC/RDW ratios discriminated HS patients from healthy controls (Fig. 1). However, they could not differentiate HS subjects from those with anemia due to other causes and no significant differences (P>0.05) were observed between them. The Hb/MCHC ratio was much higher in two HS male patients because they had greater Hb values (134 and 154 g/L, respectively). In contrast, two children with very low Hb, one with HS and the other with S hemoglobinopathy, had lower Hb/MCHC ratios (Fig. 1). Regarding Hb/RDW ratio, two male subjects (HS and normal) showed higher values due to higher Hb levels (154 and 161 g/L, respectively) (Fig. 1). Among healthy controls, the ranges and cutoff value for HS ratios, according ROC curves, were Hb/MCHC=0.37–0.46 and <0.37; Hb/RDW=9.7–12.0 and <9.7; MCHC/RDW=24.3–27.9 and <24.3, respectively (Fig. 2).
According to the sensitivity, specificity, efficiency, and Youden index analyses, the best test to discriminate HS was hemolysis percentage in 5.5 g/L NaCl in the immediate and incubated EOF tests (Table 2). According to the efficiency analysis, the best tests were initial hemolysis in EOF test, MCHC, CHT, and Hb/MCHC ratio, in that order. The Youden index for RDW, Hb/RDW, and MCHC/RDW reached the lowest values.
The correct diagnosis of HS and its differentiation from other causes of increased hemolysis is of critical importance for therapeutic decisions. Although British experts recommend no further testing when family history and laboratory findings match the diagnosis of HS, specific tests are still necessary in order to assess the fragility of red blood cells to confirm the diagnosis in geographical areas where the prevalence of the disease is low. However, there are also cases of very mild expression and other atypical cases in which several tests are required to confirm the diagnosis. It is, therefore, very important to consider the specificity, sensitivity, and efficiency of the methods used in the detection of HS.
The survey by the European Network for Rare Red Cell Anemias showed that the most frequently used tests for HS diagnosis included EMA binding (60% of centers) and immediate EOF and acidified glycerol lysis time test (around 50% of centers). Less than 20% of the centers used the CHT. Ektacytometry, sodium dodecyl sulfate polyacrylamide gel electrophoresis (SDS-PAGE), and molecular analysis were performed only in selected atypical cases [18]. Therefore, despite the recommendations of the British Committee for Standards in Haematology, the CHT has not yet been incorporated by many HS diagnostic centers [10]. The survey also revealed a lack of consensus on which screening test(s) have the best specificity and sensitivity for detecting HS. The majority of centers indicated the use of a combination of tests [18].
The CHT values for the normal group in the present study were similar to those reported by Streichman and Gescheidt in 42 healthy controls (7.0±4.4%); however, the HS groups were more heterogeneous (36.7±24.4%) because for them CHT values were 42±13% [15]. In 1990, Streichman et al. reported a CHT sensitivity of 100% and specificities of 94%, 97%, and 86% in healthy controls, hospitalized patients, and autoimmune hemolytic anemia (AIHA) patients, respectively [7]. Similarly, Iglauer et al. [19] calculated a 95% sensitivity and 96% specificity; however, the CHT sensitivity reported by Mariani et al. [20] was just 53%. Crisp et al. [21] reported a 78.7% sensitivity and 95.3% specificity and found the CHT more efficient than the EMA binding test. However, Park et al. [22] reported CHT sensitivity and specificity of 48.5% and 76.7%, respectively; when CHT was compared to the EMA binding and FCM OF tests, it showed the worst behavior. In agreement with the majority of the authors, in the present study, CHT was very specific (92%) but moderately sensitive (62%). The differences may be due to the different types of anemia included in the group of patients with another etiology, which was mainly microcytic hypochromic. Iglauer et al. [19] included mostly patients with hemolytic anemia, while Park et al. [22] selected subjects with anemia of chronic disease (ACD), iron deficiency anemia (IDA), and AIHA. In addition, there were methodological differences, as well as differences in reference ranges, among the studies. According to the ROC curve analysis, the method sensitivity would be improved by changing the cutoff to 15%.
The spherocytic shape and surface area/volume ratio of red cells do not influence the CHT. The HbS erythrocytes in one patient were sensitive in the CHT. This effect was described by Streichman et al., who found it to be independent of the patient's clinical condition and the hematologic parameters. On the other hand, β-thalassemia trait carriers were no more resistant to cryohemolysis than patients with normal cells, as reported by Streichman et al. [14]. Conversely, one patient showed increased CHT values. Recently, Crisp et al. [23] demonstrated that patients with HS have a lower level of aquaporin-1 (AQP1) in their erythrocyte membranes, which is correlated with the disease severity. The behavior of erythrocytes in the CHT does not depend only on membrane protein defects but also on the depletion of AQP1. Therefore, multiple mechanisms may explain blood cell lysis in cold hypertonic media.
The Youden index is a main summary statistic of ROC curves used for the interpretation and evaluation of a biomarker and defines their maximum potential effectiveness. The immediate and incubated hemolysis percentage in 5.5 g/L NaCl and initial hemolysis in the EOF test showed the highest Youden index levels. This particular salt concentration was chosen because it provided the best discriminatory results between normal and HS samples in previous studies. Streichman and Gescheidt observed that the incubated EOF at 5.5 g/L NaCl was a much better parameter than the salt concentration that causes 50% hemolysis (H50) because it identified all probands, but failed to identify two obligatory carriers of the disease, while the H50 resulted in six false negative subjects [15]. Similar to previous reports, the sensitivity of the EOF was greater when performed on incubated blood than on fresh blood [152024]. With respect to initial hemolysis, Aixalá and Sarandria described similar results (6.1±1.1) [25]. The efficiency of the immediate and incubated 5.5 g/L NaCl in a single tube makes this an advisable screening method for laboratories that lack the specific methodology for HS diagnosis.
The hematimetric indices provided by hematological counters are inexpensive and not time-consuming and provide an automatic red cell morphological description. Red blood cells in patients with HS have a normal shaped when young but become progressively thicker with age. The mean cell volume (MCV) decreases slightly and the MCHC increases as they acquire the spherocytic and hyperchromic appearances typical of the disorder [26]. The reticulocyte response of these individuals, together with the presence of microspherocytes, results in marked anisocytosis in peripheral blood smears and an increased RDW [12]. Automated MCHC and RDW measurement is useful for the diagnosis of HS. Michaels et al. [27] reported that an MCHC cutoff value >350 g/L provided 70% sensitivity and 86% specificity; similarly, they were 85% and 97%, respectively, with an RDW cutoff >14%. Eberle et al. [12] proposed different cutoff levels (MCHC>345 g/L and RDW>14.5%); for MCHC, they reported a 86.17% sensitivity and 90.43% specificity and 93.62% and 88.30%, respectively, for RDW. Using an MCHC>355 g/L as diagnostic criteria for HS, Tao et al. [28] observed a sensitivity of only 41.07% and specificity of 94.47%. However, only MCHC appeared to be a good marker of disease because RDW was not sensitive and specific and had one of the lowest Youden index values (0.44). Although the MCHC and RDW are sufficiently efficient, not all patients with HS demonstrated elevation of both indexes. Like Michaels et al., the combination of both indexes increased sensitivity to 100% and Youden index at 0.68 [27].
Patients with HS had significantly lower Hb/MCHC, Hb/RDW, and MCHC/RDW ratios than those of the healthy controls in the present study; however, these indexes did not differ significantly from those of patients with other anemia. These results can be attributed to the decreased Hb and increased MCHC and RDW in patients with HS. For Rocha et al., [13] these ratios appeared to be more sensitive markers than MCHC and RDW alone. However, the actual results did not match; thus, the calculated indexes were very sensitive but not specific. The Hb/MCHC range in healthy controls was similar to the 0.36–0.48 reported by Park et al.; however, they described a higher ratio in HS subjects than in patients with AIHA, ACD, and IDA. The last effect was not observed in the present study, likely because Park et al. [22] observed higher MCHC values in subjects with other anemias.
The most traditional method for HS diagnosis is the EOF test; however, it is laborious and cumbersome. Several authors reported that EOF failed to identify nearly one-quarter of patients with HS [415192129], but incubated EOF is still generally considered the gold standard for diagnosing HS in patients with DCT-negative hemolytic anemia [24]. EMA binding and FCM OF tests are satisfactory for discriminating patients with HS since their sensitivity and specificity were, respectively, above 70% and 95% [2122303132]. Since flow cytometers are not available in all diagnostic laboratories, as well as specific tests for HS diagnosis, the immediate and incubated EOF at 5.5 g/L NaCl single tube may be an alternative screening method.
A limitation of this work is the lack of measurement of EMA binding, which is recommended in cases of equivocal HS diagnosis [101132]. Therefore, it is likely that mild cases of HS were not detected using the actual diagnostic tests. The low number of samples is another limitation of this report and is related to the low prevalence of the pathology in the study region.
It is clear that the definitive diagnosis of HS, especially mild and atypical cases, requires several laboratory tests. Bolton-Maggs et al. [29] recommended the selection of an appropriate test for determining mild or atypical HS condition in a patient should consider the sensitivity and specificity of the test for HS, the complexity of the protocol, and the total cost of instrument(s) and its maintenance. In low-income countries, besides EOF test, the CHT and MCHC are advisable for the diagnosis of HS patients because of their low cost, simple procedure, quick results, minimal blood sample requirement, and specificity.
Notes
References
1. Gallagher PG. Abnormalities of the erythrocyte membrane. Pediatr Clin North Am. 2013; 60:1349–1362. PMID: 24237975.


2. Basu A, Chakrabarti A. Defects in erythrocyte membrane skeletal architecture. Adv Exp Med Biol. 2015; 842:41–59. PMID: 25408336.


3. Delaunay J. The molecular basis of hereditary red cell membrane disorders. Blood Rev. 2007; 21:1–20. PMID: 16730867.


4. Iolascon A, Avvisati RA. Genotype/phenotype correlation in hereditary spherocytosis. Haematologica. 2008; 93:1283–1288. PMID: 18757847.


5. King MJ, Zanella A. Hereditary red cell membrane disorders and laboratory diagnostic testing. Int J Lab Hematol. 2013; 35:237–243. PMID: 23480868.


6. Bucx MJ, Breed WP, Hoffmann JJ. Comparison of acidified glycerol lysis test, Pink test and osmotic fragility test in hereditary spherocytosis: effect of incubation. Eur J Haematol. 1988; 40:227–231. PMID: 3356239.


7. Streichman S, Gesheidt Y, Tatarsky I. Hypertonic cryohemolysis: a diagnostic test for hereditary spherocytosis. Am J Hematol. 1990; 35:104–109. PMID: 2399901.


8. King MJ, Behrens J, Rogers C, Flynn C, Greenwood D, Chambers K. Rapid flow cytometric test for the diagnosis of membrane cytoskeleton-associated haemolytic anaemia. Br J Haematol. 2000; 111:924–933. PMID: 11122157.


9. Won DI, Suh JS. Flow cytometric detection of erythrocyte osmotic fragility. Cytometry B Clin Cytom. 2009; 76:135–141. PMID: 18727072.


10. Bolton-Maggs PH, Langer JC, Iolascon A, Tittensor P, King MJ. General Haematology Task Force of the British Committee for Standards in Haematology. Guidelines for the diagnosis and management of hereditary spherocytosis-2011 update. Br J Haematol. 2012; 156:37–49. PMID: 22055020.
11. King MJ, Garçon L, Hoyer JD, et al. ICSH guidelines for the laboratory diagnosis of nonimmune hereditary red cell membrane disorders. Int J Lab Hematol. 2015; 37:304–325. PMID: 25790109.


12. Eberle SE, Sciuccati G, Bonduel M, Díaz L, Staciuk R, Torres AF. Erythrocyte indexes in hereditary spherocytosis. Medicina (B Aires). 2007; 67:698–700. PMID: 18422060.
13. Rocha S, Costa E, Rocha-Pereira P, et al. Complementary markers for the clinical severity classification of hereditary spherocytosis in unsplenectomized patients. Blood Cells Mol Dis. 2011; 46:166–170. PMID: 21138793.


14. Streichman S, Kahana E, Tatarsky I. Hypertonic cryohemolysis of pathologic red blood cells. Am J Hematol. 1985; 20:373–381. PMID: 4073012.


15. Streichman S, Gescheidt Y. Cryohemolysis for the detection of hereditary spherocytosis: correlation studies with osmotic fragility and autohemolysis. Am J Hematol. 1998; 58:206–212. PMID: 9662272.


16. Roper D, Layton M. Investigation of the hereditary haemolytic anaemias: membrane and enzyme abnormalities. In : Lewis SM, Bain BJ, Bates I, editors. Dacie and Lewis practical haematology. 10th ed. Philadelphia, PA: Churchill Livingstone;2006. p. 205–237.
17. Ledesma Achem E, Haro C, Jiménez C, Issé B, Lazarte S. Application of the cryohemolysis test in the diagnosis of hereditary spherocytosis. Biocell. 2013; abst 137. (Annual Scientific Meeting Abstracts).
18. Vives-Corrons JL, Manú Pereira MM, Romeo-Casabona C, editors. ENERCA recommendations for centres of expertise in rare anaemias. A white book. Madrid, Spain: Prodrug Multimedia, S.L.;2013. p. 140–142.
19. Iglauer A, Reinhardt D, Schröter W, Pekrun A. Cryohemolysis test as a diagnostic tool for hereditary spherocytosis. Ann Hematol. 1999; 78:555–557. PMID: 10647879.


20. Mariani M, Barcellini W, Vercellati C, et al. Clinical and hematologic features of 300 patients affected by hereditary spherocytosis grouped according to the type of the membrane protein defect. Haematologica. 2008; 93:1310–1317. PMID: 18641031.


21. Crisp RL, Solari L, Vota D, et al. A prospective study to assess the predictive value for hereditary spherocytosis using five laboratory tests (cryohemolysis test, eosin-5′-maleimide flow cytometry, osmotic fragility test, autohemolysis test, and SDS-PAGE) on 50 hereditary spherocytosis families in Argentina. Ann Hematol. 2011; 90:625–634. PMID: 21080168.


22. Park SH, Park CJ, Lee BR, et al. Comparison study of the eosin-5′-maleimide binding test, flow cytometric osmotic fragility test, and cryohemolysis test in the diagnosis of hereditary spherocytosis. Am J Clin Pathol. 2014; 142:474–484. PMID: 25239414.


23. Crisp RL, Maltaneri RE, Vittori DC, et al. Red blood cell aquaporin-1 expression is decreased in hereditary spherocytosis. Ann Hematol. 2016; 95:1595–1601. PMID: 27465156.


24. Bianchi P, Fermo E, Vercellati C, et al. Diagnostic power of laboratory tests for hereditary spherocytosis: a comparison study in 150 patients grouped according to molecular and clinical characteristics. Haematologica. 2012; 97:516–523. PMID: 22058213.


25. Aixála MT, Sarandría CN. Microspherocytosis. Erythroid profile and its relation with different laboratory tests. Medicina (B Aires). 2001; 61:417–423. PMID: 11563170.
26. Conway AM, Vora AJ, Hinchliffe RF. The clinical relevance of an isolated increase in the number of circulating hyperchromic red blood cells. J Clin Pathol. 2002; 55:841–844. PMID: 12401822.


27. Michaels LA, Cohen AR, Zhao H, Raphael RI, Manno CS. Screening for hereditary spherocytosis by use of automated erythrocyte indexes. J Pediatr. 1997; 130:957–960. PMID: 9202619.


28. Tao YF, Deng ZF, Liao L, Qiu YL, Chen WQ, Lin FQ. Comparison and evaluation of three screening tests of hereditary spherocytosis in Chinese patients. Ann Hematol. 2015; 94:747–751. PMID: 25501660.


29. Bolton-Maggs PH, Stevens RF, Dodd NJ, et al. Guidelines for the diagnosis and management of hereditary spherocytosis. Br J Haematol. 2004; 126:455–474. PMID: 15287938.


30. Ciepiela O, Adamowicz-Salach A, Zgodzińska A, Łazowska M, Kotuła I. Flow cytometric osmotic fragility test: Increased assay sensitivity for clinical application in pediatric hematology. Cytometry B Clin Cytom. 2017; [Epub ahead of print].


31. Kuzminova JA, Plyasunova SA, Jogov VV, Smetanina NS. The cytometric technique of binding of eosin-5-maleimide in diagnostic of inherent spherocytosis. Klin Lab Diagn. 2016; 61:168–172. PMID: 27506108.
32. Farias MG. Advances in laboratory diagnosis of hereditary spherocytosis. Clin Chem Lab Med. 2017; 55:944–948. PMID: 27837594.


Fig. 1
CHT and HS discriminator indices in the groups under study.
Abbreviations: CHT, cryohemolysis test; HS, hereditary spherocytosis; MCHC, mean corpuscular hemoglobin concentration; N, normal; RDW, red blood cell distribution width.
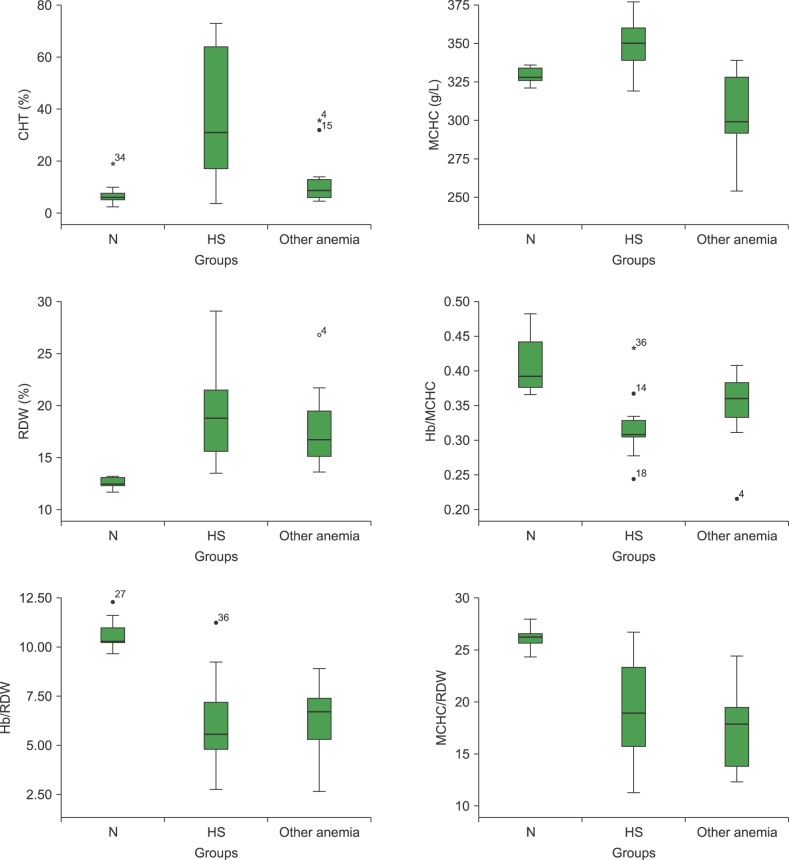
Fig. 2
Receiver operating characteristic (ROC) curves of the test results for discriminating HS patients from those with other anemias A: ROC curves of CHT, MCHC, and RDW; B: ROC curves of calculated ratios.
Abbreviations: CHT, cryohemolysis test; HS, hereditary spherocytosis; MCHC, mean corpuscular hemoglobin concentration; RDW, red blood cell distribution width.
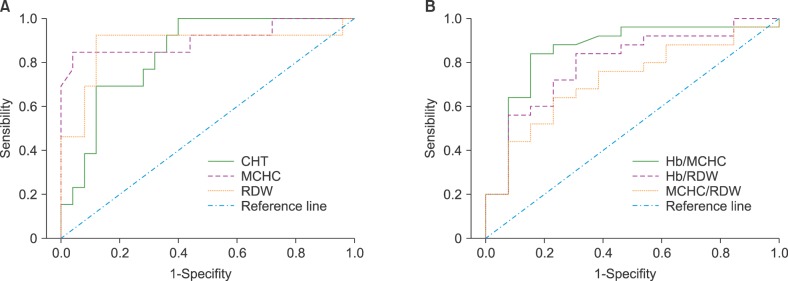
Table 1
Results in the normal, HS, and anemia due to other causes groups.
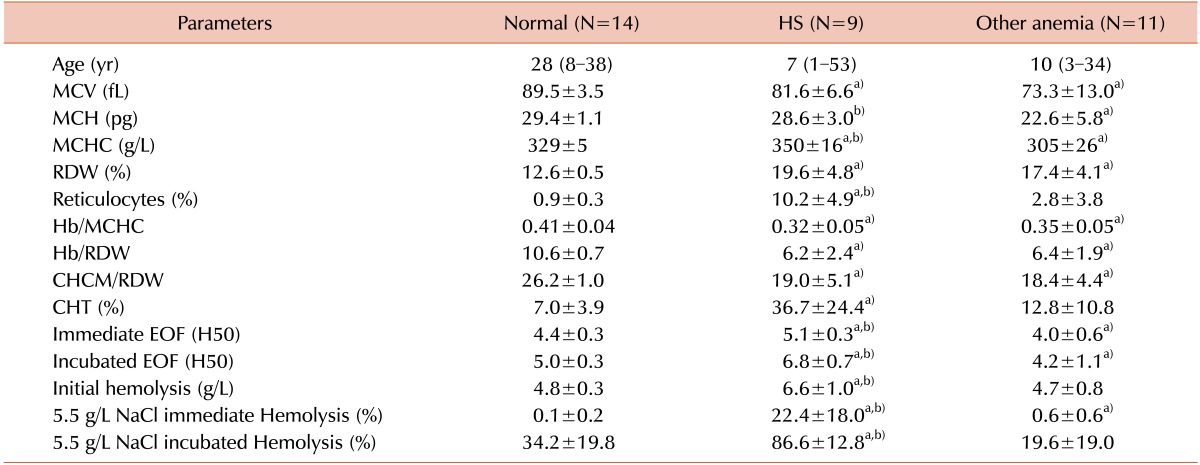
a)P<0.05 between the normal and patient groups; b)P<0.05 between the HS and other anemia groups.
Abbreviations: M, median; R, range; CHT, cryohemolysis test; EOF, erythrocyte osmotic fragility; H50, 50% hemolysis; MCH, mean corpuscular hemoglobin; MCHC, mean corpuscular hemoglobin concentration; MCV, mean corpuscular volume; RDW, red blood cell distribution width.