TO THE EDITOR: This study compared the erythropoietic capacities of erythropoietin (EPO) and antioxidant drug U-74389G based on the findings of 2 preliminary studies. Hemoglobin augmentation was evaluated using a hypoxia reoxygenation (HR) protocol in an animal model. Hemoglobin levels were evaluated at the 60th reoxygenation minute (for groups A, C, and E) and at the 120th reoxygenation minute (for groups B, D, and F) in 60 rats. Groups A and B were administered no drugs, groups C and D were administered EPO, and groups E and F were administered U-74389G. The first preliminary study of EPO did not show a significant increase in hemoglobin levels. However, the second preliminary study of U-74389G showed a significant increase in hemoglobin levels by 2.5±1.3% (P=0.0423). These 2 studies were co-evaluated because they were conducted in the same experimental setting. In non-deficient EPO rats, U-74389G demonstrated an approximately 2-times higher erythropoietic potency than EPO (P=0.0000). This is because the anti-oxidant capacity of U-74389G increased the acute erythropoietic potency.
A previous study claims that U-74389G harbors a remarkable acute erythropoietic capacity [1]. U-74389G is a novel antioxidant factor and has shown tissue protective effects in tissue hypoxia and reoxygenation (HR) experiments. U-74389G, also known as 21-[4-(2,6-di-1-pyrrolidinyl-4-pyrimidinyl)-1-piperazinyl]-pregna-1,4,9(11)-triene-3,20-dione maleate salt, prevents both arachidonic acid-induced and iron-dependent lipid peroxidation. It has been shown to protect against HR injury in animal heart, liver, and kidney models. These membrane-associating anti-oxidants are particularly effective in preventing permeability changes in brain microvascular endothelial cells monolayers. Lazaroids, or 21-aminosteroids, a novel series of glucocorticoid compounds, scavenge free radicals. U-74389G is one of the 132 similar lazaroid compounds. It has a molecular weight of 726.90406 g/mol and demonstrates selective action on the vascular endothelium with vitamin E-like properties.
However, the erythropoietic capacity of U-74389G appears more comprehensible when compared with that of a standard known drug. One such well-studied drug, wherein erythropoietic capacity was confirmed (P=0.3984), is EPO. Indeed, EPO has been implicated in over 29,946 known biomedical studies. Among these studies, 30.65% concern tissue HR experiments. However, only a few reports that were found to be related with this study did not completely address the specific matter of antioxidant factors. The aim of this experimental work was to compare the acute erythropoietic capacities of U-74389G and EPO in a non-deficient EPO rat model using an HR protocol. Their effects were assessed on the basis of increase in hemoglobin levels.
The veterinarian licenses for the research were provided under the 3693/12-11-2010 & 14/10-1-2012 decisions. The institute and place of experiment are mentioned in the related references [1 2]. The experimental protocol, which involved Albino female Wistar rats, adhered to the ethical rules of the relevant organization. For 7 days pre-experimentally, the rats were placed under normal housing and fed ad libitum in the laboratory. Continuous intra-experimental general anesthesia, oxygen supply, electrocardiography, acidometry, and post-experimental euthanasia were provided. Subsequently, 16–18-week-old rats were randomly divided into 6 groups (N=10) according to the HR protocol: hypoxia for 45 minutes followed by reoxygenation for 60 minutes (group A); hypoxia for 45 minutes followed by reoxygenation for 120 minutes (group B); hypoxia for 45 minutes followed by immediate intravenous (IV) EPO administration and reoxygenation for 60 minutes (group C); hypoxia for 45 minutes followed by immediate IV EPO administration and reoxygenation for 120 minutes (group D); hypoxia for 45 minutes followed by immediate U-74389G IV administration and reoxygenation for 60 minutes (group E); hypoxia for 45 minutes followed by immediate U-74389G IV administration and reoxygenation for 120 minutes (group F). The dose height selection criteria for EPO and U-74389G were assessed in preliminary studies as 10 mg/kg body mass for both drugs.
Hypoxia was induced by laparotomic clamping of the inferior aorta over the renal arteries with forceps for 45 minutes. Clamp removal restored the inferior aorta patency and reoxygenation. After exclusion of the blood flow, the HR protocol was followed as described above for each experimental group. The drugs were administered at the time of reperfusion through the inferior vena cava catheter. The hemoglobin levels were determined at the 60th minute of reoxygenation (for groups A, C, and E) and the 120th minute of reoxygenation (for groups B, D, and F).
Table 1 presents the percent (%) augmentation influence of both drugs according to reoxygenation time. Chi-square tests were applied using the ratios, which yielded the percent (%) results per endpoint (Table 2). The statistical analysis was performed using Stata 6.0 (Stata 6.0, StataCorp LP, Texas, USA). The chi-square tests revealed that U-74389G favored erythropoiesis at 1 hour by 1.268689-fold (1.26728–1.2701) compared with EPO by 1.839035-fold (1.836445–1.84163) at 1.5 hour, by 13.1658-fold (13.11695–13.21483) at 2 hour, by 1.252422-fold (1.250726–1.25412) without drugs, and by 1.94889-fold (1.945431–1.952355) when all variables were considered (P=0.0000).
The only available study investigating the effect of U-74389G on increasing hemoglobin levels was a preliminary one [1]. Himmelfarb et al. [3] reported an increase in the markers of oxidative stress (OS), such as F2 isoprostanes and isofurans, with decreased EPO responsiveness; however, they failed to confirm the hypothesis that oral antioxidant therapy administered over 6 months would decrease the levels of OS biomarkers and improve erythropoietic response in patients receiving maintenance hemodialysis therapy. Jilani and Iqbal [4] observed that the prevention of polyunsaturated fatty acid oxidation in red blood cell membranes reduced the fragility of erythrocytes, inhibited premature erythrocytolysis, increased the number of colony forming units of erythroid precursors, enhanced erythropoiesis, and improved blood hemoglobin levels. Martinez Mdel et al. [5] reported reduced free heme pool-inducing heme oxygenase levels and δ-aminolevulinate synthase activity and a low rate of holo/apo tryptophan pyrrolase activity. Prats et al. [6] suggested that impaired erythrocyte antioxidant defense and OS may play a role in the development of anemia in chronic kidney disease and promote erythropoiesis-stimulating agent resistance because of lower extracellular glutathione peroxidase activity in non-responders to erythropoiesis-stimulating agents. Martinez Mdel et al. [7] reported, with regard to the heme biosynthetic pathway, that a decrease in δ-aminolevulinate synthase activity exerts antioxidant-protective effects on OS, thus decreasing lipid peroxidation and the activity of some antioxidant enzymes. Goldstein and Harber [8] demonstrated lipid peroxidation as follows: (a) the formation of 2-thiobarbituric acid reactants; (b) the presence of conjugated diene bonds in red cell lipid; (c) the selective loss of unsaturated fatty acids proportional to the number of carbon-carbon double bonds. The formation of peroxides leads to oxygen-dependent colloid osmotic hemolysis, before which there is a decline in cell membrane sulfhydryl groups and a loss in activity of the cell membrane enzyme acetylcholinesterase, which is accelerated with the addition of linoleic acid.
Another study confirmed the short-term or long-term erythropoietic effect of various EPO preparations in 55 IR laboratory or clinical biomedical studies on human individuals or animals [2]. Arslantaş et al. [9] reported increasing hemoglobin levels after subsequent intraperitoneal 400 µ/kg/day EPO injections following a wound formation, administered once daily for up to 10 days after operation and accompanied by a transient surge in healing on day 5 in Sprague-Dawley rats. Khodosovskiĭ and Zinchuk [10] showed that hepatic 30-minute ischemia/120-minute reperfusion (IR) led to significant decreases in the hemoglobin oxygen affinity. Recombinant human erythropoietin (rhEPO) administered at 100 IU/kg aggravates the decreasing hemoglobin oxygen affinity during IR. rhEPO infusion (id dose 1,000 IU/kg) shifts the oxyhemoglobin dissociative curve to the left. Kalantzi et al. [11] observed an early peak in serum EPO levels that occurred within 2 days after renal transplantation and a late peak, between weeks 2 and 4, which resulted in increased blood hemoglobin levels. Normal hemoglobin values were restored approximately 3 months after successful renal transplantation. Hernández-Navarrete et al. [12] observed stable hemoglobin levels at 24 months postransplant after pretransplant EPO and iron dextran administration with the use of an intraoperative cell saver in 3 kidney transplants patients. Zabaneh et al. [13] reported that most peritoneal dialysis patients with chronic kidney disease maintained hemoglobin levels between 10 g/dL and 12 g/dL (63.0%) and within ±1.0 g/dL of the baseline level (60.9%) during the 25-week evaluation period. The mean hemoglobin value during the evaluation period was 11.3±1.07 g/dL and the mean change from baseline was 0.10±1.15 g/dL (95% confidence limit; -0.24–0.44 g/dL). The median weekly epoetin α or β dose at baseline was 96.0 U/kg. Bartnicki et al. [14] claimed that EPO involves some degree of risk, which increases with increasing hemoglobin levels. A growing number of studies have assessed the renoprotective effects of EPO in acute kidney injury and chronic kidney disease.
U-74389G increases the erythropoietic potency by approximately 2-fold of that with EPO (P=0.0000; Table 3), a trend enhanced with time in non-deficient EPO animals. This trend was enhanced during the early stages of the experiment. Furthermore, a comparison of efficacy in terms of 9 hematologic variables levels showed similar outcomes (Table 3) [15].
Figures and Tables
Table 2
U-74389G/erythropoietin efficacy ratios according to hemoglobin levels augmentation after chi-square tests application.
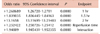
Table 3
A U-74389G/erythropoietin efficacies ratios meta-analysis on 9 hematologic variableς (7 variables with balancing efficacies and 2 variables with opposite efficacies) [15].
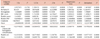
References
1. Tsompos C, Panoulis C, Toutouzas K, Zografos G, Papalois A. The effect of the antioxidant drug “U-74389G” on haemoglobin levels following a hypoxemia/re-oxygenation protocol in rats. J Crit Care Med. 2015; 1:102–106.


2. Tsompos C, Panoulis C, Toutouzas K, Zografos G, Papalois A. The short-term effect of erythropoietin on hemoglobin during ischemia reperfusion injury in rats. Fiziologia. 2013; 23:26–32.
3. Himmelfarb J, Ikizler TA, Ellis C, et al. Provision of antioxidant therapy in hemodialysis (PATH): a randomized clinical trial. J Am Soc Nephrol. 2014; 25:623–633.


4. Jilani T, Iqbal MP. Does vitamin E have a role in treatment and prevention of anemia. Pak J Pharm Sci. 2011; 24:237–242.
5. Martinez Mdel C, Afonso SG, Meiss RP, Buzaleh AM, Batlle A. Hepatic damage and oxidative stress induced by Griseofulvin in mice. Cell Mol Biol (Noisy-le-grand). 2009; 55:127–139.
6. Prats M, Font R, García C, et al. Oxidative stress markers in predicting response to treatment with ferric carboxymaltose in nondialysis chronic kidney disease patients. Clin Nephrol. 2014; 81:419–426.


7. Martinez Mdel C, Afonso SG, Buzaleh AM, Batlle A. Protective action of antioxidants on hepatic damage induced by griseofulvin. ScientificWorldJournal. 2014; 2014:982358.
8. Goldstein BD, Harber LC. Erythropoietic protoporphyria: lipid peroxidation and red cell membrane damage associated with photohemolysis. J Clin Invest. 1972; 51:892–902.


9. Arslantaş MK, Arslantaş R, Tozan EN. Effects of systemic erythropoietin on ischemic wound healing in rats. Ostomy Wound Manage. 2015; 61:28–33.
10. Khodosovskiĭ MN, Zinchuk VV. Erythropoietin influence on the blood oxygen transport and prooxidant-antioxidant state during hepatic ischemia-reperfusion. Ross Fiziol Zh Im I M Sechenova. 2014; 100:592–601.
11. Kalantzi M, Kalliakmani P, Papachristou E, et al. Parameters influencing blood erythropoietin levels of renal transplant recipients during the early post-transplantation period. Transplant Proc. 2014; 46:3179–3182.


12. Hernández-Navarrete LS, Hernández-Jiménez JD, Jiménez-López LA, Budar-Fernández LF, Méndez-López MT, Martínez-Mier G. Experience in kidney transplantation without blood transfusion: kidney transplantation transfusion-free in Jehovah's Witnesses. First communication in Mexico. Cir Cir. 2013; 81:450–453.
13. Zabaneh R, Roger SD, El-Shahawy M, et al. Peginesatide to manage anemia in chronic kidney disease patients on peritoneal dialysis. Perit Dial Int. 2015; 35:481–489.


14. Bartnicki P, Kowalczyk M, Rysz J. The influence of the pleiotropic action of erythropoietin and its derivatives on nephroprotection. Med Sci Monit. 2013; 19:599–605.


15. Tsompos C, Panoulis C, Toutouzas K, Triantafyllou A, Zografos GC, Papalois A. Comparison of the attenuating capacities of erythropoietin and U-74389G concerning blood platelet counts. J Sci Achiev. 2017; 2:18–21.