Abstract
Background
Immune thrombocytopenia (ITP) is an immune-mediated disease caused by autoantibodies against platelets membrane glycoproteins GPIIb/IIIa and GPIb/IX. The etiology of ITP remains unclear. This study evaluated the association of polymorphisms in interleukin (IL)-1B-31, IL-1B-511, and IL-1Ra with ITP.
Methods
Genotyping of IL-1B-31, IL-1B-511, and IL-1Ra was performed in 118 ITP patients and 100 controls by polymerase chain reaction restriction fragment length polymorphism and detection of variable number tandem repeats.
Results
Genotype differences in IL-1B-31 and IL-1Ra were significantly associated with ITP. Patients showed a higher frequency of the IL-1B-31 variant allele (T) and a 1.52-fold greater risk of susceptibility to ITP (odds ratio [OR]=1.52, 95% confidence interval [CI]=1.04–2.22, P=0.034). The frequencies of both homozygous and heterozygous variant genotypes of IL-1B-31 were higher (OR=2.33, 95% CI=1.069–5.09, P=0.033 and OR=2.044, 95% CI=1.068–39, P=0.034) among patients and were significantly associated with ITP susceptibility. Both homozygous and heterozygous variant genotypes of IL-1Ra were also more frequent (OR=4.48, 95% CI=1.17–17.05, P=0.0230 and OR=1.80, 95% CI=1.03–3.14, P=0.0494) among patients and were associated with ITP risk. IL-1B-31 and IL-1Ra also showed significant association with severe ITP. However, IL-1B-511 was not associated with ITP.
Immune thrombocytopenia (ITP) is an immune-mediated disease caused by autoantibodies against glycoproteins (GP) IIb/IIIa and GPIb/IX found in the platelet membrane [1]. According to recent guidelines from the International Working Group, ITP is clinically classified into three types, newly diagnosed (for all cases at diagnosis), persistent (for cases with ITP lasting between 3–12 mo from diagnosis, spontaneous remission not achieved after the removal of treatment in between 3–12 mo from diagnosis), and chronic ITP (lasting for more than 12 mo) [2]. The etiology of ITP remains unclear, but both environmental and genetic factors appear to play an important role in its pathogenesis [3]. The development of autoantibodies by B cells, multi-dysfunction in cellular immunity, and cytokine dysregulation are thought to play central roles in the pathophysiology of ITP [4].
A high Th1/Th2 ratio and inflammatory cytokine gene polymorphisms were shown to be associated with ITP [567]. T cell activity and cytokine abnormalities were found to play a major role in the pathophysiology of autoimmune diseases, including ITP [89]. Cytokines are key players known to maintain the balance between Th1 and Th2 cells. An Th1/Th2 imbalance leads to autoreactive B cell differentiation in ITP, and glucocorticoid treatment helps to restore the levels of Th1 and Th2 in ITP patients [10]. Activation of CD4+ T cells leads to a proinflammatory response with the production of autoreactive antibodies and increased expression of interleukin-2 (IL-2) and interferon-γ [111213].
Among genetic factors, polymorphisms in inflammatory cytokine genes, human leukocyte antigen, Fcγ receptors, and tumor necrosis factor are related to ITP [141516]. In addition, Wu et al. [1718] found that IL-4, IL-10, and IL-1Ra polymorphisms contribute to childhood chronic ITP, while an IL-1β exon 5 polymorphism is associated with childhood ITP. In ITP, IL-1 family members are involved in the stimulation of megakaryocytopoiesis, regulation of platelet production, and generation of autoantibodies [19]. The IL-1 family consists of three related genes, IL-1A, IL-1B, and IL-1Ra, and each plays a different functional role in autoimmune diseases and ITP.
IL-1B and IL-1Ra are found on chromosome 2q14 within a 360-kb region. IL-1 beta (IL-1β) is a proinflammatory cytokine released by macrophages in systemic inflammatory responses and regulates inflammatory reactions and immune responses by promoting cytokines such as IL-6 and IL-12 [7]. IL-1B has two diallelic polymorphisms at positions −511 and −31 in the promoter region and at position +3954 in the fifth exon. The polymorphism −31 T>C (rs1143627) in the promoter region affects IL-1B expression. IL-1Ra is a penta-allelic polymorphic site in intron 2 with variable numbers of an 86-base pair (bp) tandem repeat sequence. Pociot and Addas-Carvalho reported that the IL-1B −511 T and +3954 T alleles enhance IL-1β and IL-1Ra production in humans [2021].
However, few studies have examined polymorphisms in ITP and most have evaluated children and adult from only two Eastern populations, Japan and China. No studies have been conducted on Indian subjects; therefore, our aim was to evaluate the correlation of polymorphisms in IL-1β and IL-1Ra with ITP.
The study protocol was approved by the Institutional Ethical Committee. Diagnosis, classification (severe ITP and non-severe ITP), and response assessment were conducted according to the International Working Group Classification 2009. Briefly, ITP was diagnosed as isolated thrombocytopenia (platelet counts <100×109/L) in the absence of other causes or disorders that may be associated with thrombocytopenia. Cases of ITP associated with secondary causes such as human immune deficiency virus, systematic lupus erythematous, and Helicobacter pylori were excluded from the study. The response criteria were as follows: 1. Complete response, platelet count ≥100×109/L measured on two occasions >7 days apart and the absence of bleeding, 2. Response, platelet count ≥30×109/L and at least 2-fold increases in platelets count from baseline measured on 2 occasions >7 days apart and the absence of bleeding, 3. No response, platelet counts <30×109/L or less than 2-fold increase in baseline platelets count or bleeding. The presence of bleeding at presentation sufficient to mandate treatment or the occurrence of new bleeding requiring additional therapeutic intervention with a different platelet-enhancing agent or an increase dose was classified as severe ITP. All others were classified as non-severe ITP [2]. Sample size was calculated using Quanto software version 1.2 by an institutional statistician. Samples were collected after obtaining informed consent from 118 cases and 100 controls.
Five milliliters of peripheral venous blood were collected in EDTA vials from all participants and stored at −80℃. Genomic DNA was extracted using a commercially available genomic DNA extraction kit, the QIAamp DNA extraction Kit (Blood DNA Mini Kit, Qiagen, Hilden, Germany) according to the blood and body fluid spin protocol. Genotyping was performed by polymerase chain reaction-restriction fragment length polymorphism. The reaction mixture of genotypes consisted of 10X buffer containing MgCl2 (2.5 µL), Taq 1 U/µL (1.5 µL), dNTPs 10 mmol (1 µL), forward primer 10 pmol (1 µL), reverse primer 10 pmol (1 µL), DNA (1.5–50 ng/µL), and 16.5 µL high-performance liquid chromatography-grade water in a 25-µL reaction mixture. Primer sequences of IL-1B-31 C>T rs1143627 and IL-1B-511 T>C rs16944 were as follows: forward- 5′-AGCTTCCACCAATACTCTTTTCCCCTTTCC-3′, reverse 5′-TACACACAAAGAGGCAGAGAGACAGA-3′ and forward 5′-TGG CATTGATCTGGTTCATC-3′ and reverse 5′-GTTTAGGAATCTTCCCACTT-3′. The primer sequence for IL-1Ra was forward 5′-CCCCTCAGCAACACTCC-3′ and reverse 5′-GGTCAG AAGGGCAGAGA-3′. PCR conditions were as follows: initial denaturation at 110℃ for 5 min, 35 cycles of denaturation at 94℃ for 45 sec, annealing for 45 sec (at 62.3℃ for IL-1B-31, at 63℃ for IL-1B-511), and extension at 72℃ for 1.5 min, followed by final extension at 72℃ for 5 min and holding at 15℃. Aliquots of amplified uncut PCR products were resolved by electrophoresis in 2% agarose gels with 0.5 µg/mL ethidium bromide and visualized under a UV illuminator. Samples were then used for restriction fragment length polymorphism analysis. For both IL-1B-31 and IL-1B-511, the PCR products were digested with 2 U of Ava I for IL-1B-31 and Alu I for IL-1B-511 (New England Biolabs, Ipswich, MA, USA) restriction enzymes at 37℃ overnight. The IL-1Ra PCR products and product sizes were calculated relative to the 100-bp DNA ladder. The product sizes of different alleles were as follows: I-IL1Ra 1, 410 bp; II-L1Ra 2, 240 bp; III-IL1Ra 3, 500 bp; IV-IL1Ra 4, 325 bp; and V-IL1Ra 5, 595 bp. The digested products were separated by 15% polyacrylamide gel electrophoresis and stained with ethidium bromide. Gel images were acquired using the molecular imager gel doc XR System (Bio-Rad, Hercules, CA, USA).
Allele and genotype frequencies were estimated by the allele counting method. Hardy-Weinberg estimates (HWE) for genotype frequencies were calculated using Haploview software. HWE was tested using the χ2 test with a degree of freedom of 1. A P-value <0.05 was considered to indicate statistical significance. Genotypes were analyzed under additive, recessive, and dominant models of inheritance. Odds ratios were calculated and the confidence interval (95%) was estimated using Fisher's exact test. Values were considered significant when P≤0.05. All calculations were carried out using commercial statistical analysis software (GraphPad Software ver. 3.05; GraphPad, La Jolla, CA, USA).
In this study, a total of 218 individuals (Patients=118 and healthy controls=100) were recruited. ITP is found to be more prevalent in women than in men. The proportion of women in ITP patient and healthy control groups was 62.71% and 58% respectively. Severe & non-severe ITP cases were 62 (52.54%) and 56 (47.46%) respectively. Eighty patients (67.80%) showed complete response to corticosteroid therapy. All the demographic details of patients and healthy controls are given in (Table 1).
The present study revealed that genotypes of both homozygous and heterozygous variants of IL-1B-31 were higher among patients and significantly associated with the susceptibility to ITP (OR=2.33, 95% CI=1.069–5.09, P=0.033 and OR=2.044, 95% CI=1.068–39, P=0.034). The frequency of the variant allele (T) was also higher among patients and showed a 1.52-fold increased risk of susceptibility to ITP (OR=1.52, 95% CI=1.04–2.22, P=0.034). Similarly, both the homozygous and heterozygous variant genotypes of IL-1Ra were higher among the patient group and were associated with ITP risk compared to healthy controls (OR=4.48, 95% CI=1.17–17.05, P=0.0230 and OR=1.80, 95% CI=1.03–3.14, P=0.0494). The variant allele frequency of IL-1Ra also showed a significant risk association with ITP (OR=1.75, 95% CI=1.15–2.66, P=0.0091). Further, neither the genotype nor allele frequencies of IL-1B-511 showed an association with ITP (Table 2).
To determine the association between these polymorphisms and disease severity, we analyzed the data between severe, non-severe, and healthy controls. Notably, a significant association was observed between homozygous variant genotypes of IL-1B31 and severe ITP compared to healthy controls (OR=2.76, 95% CI=1.076–7.10, P=0.037). In addition, the variant allele of IL-B-31 showed a significant risk association with severe ITP (OR=1.64, 95% CI=1.046–2.58, P=0.039). We found no association between the IL-1B-511 genotype and either severe or non-severe ITP. The ORs of severe ITP and healthy controls were 0.77 (95% CI=0.180–3.28, P=1.000), OR for the heterozygous genotype was 0.92 (95% CI=0.477–1.79, P=0.867), and their variant allele frequencies were an OR of 0.90 (95% CI=0.540–1.52, P=0.793). Similarly, we found no association between the IL-1B-511 genotype and non-severe patients and controls. However, a significant difference was observed in the distribution of IL-1Ra genotypes among severe ITP patients and healthy controls. A significant association was observed for the homozygous variant (OR=5.83 95% CI=1.382–24.63, P=0.014). Moreover, the mutant allele (II) of IL-1Ra showed a significant risk contribution to severe ITP (OR=1.93 95% CI=1.188–3.14, P=0.0085) (Table 3).
The genotypic and allelic frequencies of IL-1B-31, IL-1B-511, and IL-1Ra are shown in Table 4. Polymorphisms in IL-1B-31, IL-1B-511, and IL-1Ra showed no association with the response to steroid in ITP patients.
ITP is an autoimmune disorder characterized by the presence of platelet antibodies that accelerate platelet destruction and inhibit their production [22]. Although the etiology of ITP remains unclear, it is generally accepted that both environmental and genetic factors play an important role in development of the disease [6152324]. Many recent studies have focused on the association between cytokine gene polymorphism ITP susceptibility [161718]. Alternations in cytokine levels may contribute to disease status [1923]. Few studies have examined cytokine gene polymorphisms in patients with ITP in India. Therefore, in the present study, we evaluated polymorphisms in various cytokine genes (IL-1B-31, IL-1B-511, and IL-1Ra) and their association with ITP susceptibility and diseases phenotype.
The data from the present study demonstrate an association between polymorphisms in both homozygous and heterozygous variants of IL-1B-31 and the susceptibility to ITP. Subjects with the variant allele showed a 1.52-fold higher susceptibility to ITP (Table 2). This differs from the results of various studies [6715], in which these associations were not detected. The difference in findings may be related to ethnic and geographical differences between study populations. This is the first study of IL-1B-31 gene polymorphism in ITP in India, and thus no comparison is possible among different ethnic groups within India. However, studies conducted in India reported an association between IL-1B-31 gene polymorphisms and other diseases such as chronic periodontitis, aggressive periodontitis, and lung cancer [2526]. Patients with ITP and IL-1B-31 polymorphism not only showed an increased susceptibility to ITP, but also had a more severe form of the disease, particularly those with homozygous mutant and variant alleles (Table 3).
We found that the presence of allele-II in IL-1Ra was associated with a 1.75-fold increased risk of developing ITP. Both the homozygous and heterozygous variant genotypes showed an association with ITP (Table 2). The heterozygous and variant allele of IL-1Ra genotypes also showed a significant association with severe ITP (Table 3). Our results differ from those described by Foster et al. [15], but their study population included children with chronic ITP rather than adults as in our study. Further, our findings are consistent with those of a previous study in which polymorphism in IL-1Ra was associated with ITP [2728]. These genes belong to the IL-1 family comprised of three related genes, IL-1A, IL-1B, and IL-1Ra, which encode the pro-inflammatory cytokines IL-1α and IL-1β and their receptor IL-1Ra. IL-1 plays a major role in inflammation-mediated autoimmune diseases. IL-1Ra is a natural anti-inflammatory molecule that neutralizes the pro-inflammatory effect of IL-1B and thus plays a role in maintaining homeostasis. It is possible that polymorphisms in IL-1B-31 and IL-1Ra alter the production of IL-1B and IL-1Ra, thus disturbing homeostasis and increasing the susceptibility to ITP.
In conclusion, IL-1B-31 and IL-1Ra polymorphisms may have a significant impact on the pathogenesis of ITP. One limitation of this study was the relatively small size of the sample used for polymorphism analysis. Thus, these results must be verified in multicenteric studies with larger samples and populations of different ethnicities.
Figures and Tables
Table 2
Influence of IL-1B-31, IL-1B-511, and IL-1Ra polymorphisms on susceptibility to ITP and on control subjects.
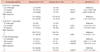
References
1. Cines DB, Bussel JB, Liebman HA, Luning Prak ET. The ITP syndrome: pathogenic and clinical diversity. Blood. 2009; 113:6511–6521.


2. Rodeghiero F, Stasi R, Gernsheimer T, et al. Standardization of terminology, definitions and outcome criteria in immune thrombocytopenic purpura of adults and children: report from an international working group. Blood. 2009; 113:2386–2393.


3. Wang T, Zhao H, Ren H, et al. Type 1 and type 2 T-cell profiles in idiopathic thrombocytopenic purpura. Haematologica. 2005; 90:914–923.
4. Johnsen J. Pathogenesis in immune thrombocytopenia: new insights. Hematology Am Soc Hematol Educ Program. 2012; 2012:306–312.


5. Ogawara H, Handa H, Morita K, et al. High Th1/Th2 ratio in patients with chronic idiopathic thrombocytopenic purpura. Eur J Haematol. 2003; 71:283–288.


6. Satoh T, Pandey JP, Okazaki Y, et al. Single nucleotide polymorphisms of the inflammatory cytokine genes in adults with chronic immune thrombocytopenic purpura. Br J Haematol. 2004; 124:796–801.


7. Suzuki T, Matsushima M, Shirakura K, et al. Association of inflammatory cytokine gene polymorphisms with platelet recovery in idiopathic thrombocytopenic purpura patients after the eradication of Helicobacter pylori. Digestion. 2008; 77:73–78.


8. Hu Y, Li H, Zhang L, et al. Elevated profiles of Th22 cells and correlations with Th17 cells in patients with immune thrombocytopenia. Hum Immunol. 2012; 73:629–635.


9. Cao J, Chen C, Li L, et al. Effects of high-dose dexamethasone on regulating interleukin-22 production and correcting Th1 and Th22 polarization in immune thrombocytopenia. J Clin Immunol. 2012; 32:523–529.


10. Guo XH, Zhao F, Shi W, et al. Detection and clinical significance of Th1/Th2 cytokines in patients with idiopathic thrombocytopenic purpura. Xi Bao Yu Fen Zi Mian Yi Xue Za Zhi. 2012; 28:1185–1187.
11. Li H, Ge J, Zhao H, et al. Association of cytotoxic T-lymphocyte antigen 4 gene polymorphisms with idiopathic thrombocytopenic purpura in a Chinese population. Platelets. 2011; 22:39–44.


12. Li Z, Mou W, Lu G, et al. Low-dose rituximab combined with short-term glucocorticoids up-regulates Treg cell levels in patients with immune thrombocytopenia. Int J Hematol. 2011; 93:91–98.


13. Sarpatwari A, Bussel JB, Ahmed M, et al. Single nucleotide polymorphism (SNP) analysis demonstrates a significant association of tumour necrosis factor-alpha (TNFA) with primary immune thrombocytopenia among Caucasian adults. Hematology. 2011; 16:243–248.


14. Kuwana M, Kaburaki J, Pandey JP, et al. HLA class II alleles in Japanese patients with immune thrombocytopenic purpura. Associations with anti-platelet glycoprotein autoantibodies and responses to splenectomy. Tissue Antigens. 2000; 56:337–343.


15. Foster CB, Zhu S, Erichsen HC, et al. Polymorphisms in inflammatory cytokines and Fcgamma receptors in childhood chronic immune thrombocytopenic purpura: a pilot study. Br J Haematol. 2001; 113:596–599.


16. Yadav DK, Tripathi AK, Kumar A, et al. Association of TNF-α −308G>A and TNF-β +252A>G genes polymorphisms with primary immune thrombocytopenia: a North Indian study. Blood Coagul Fibrinolysis. 2016; 27:791–796.


17. Wu KH, Peng CT, Li TC, et al. Interleukin 4, interleukin 6 and interleukin 10 polymorphisms in children with acute and chronic immune thrombocytopenic purpura. Br J Haematol. 2005; 128:849–852.


18. Wu KH, Peng CT, Li TC, Wan L, Tsai CH, Tsai FJ. Interleukin-1beta exon 5 and interleukin-1 receptor antagonist in children with immune thrombocytopenic purpura. J Pediatr Hematol Oncol. 2007; 29:305–308.


19. Andersson J. Cytokines in idiopathic thrombocytopenic purpura (ITP). Acta Paediatr Suppl. 1998; 424:61–64.


20. Pociot F, Mølvig J, Wogensen L, Worsaae H, Nerup J. A TaqI polymorphism in the human interleukin-1 beta (IL-1 beta) gene correlates with IL-1 beta secretion in vitro. Eur J Clin Invest. 1992; 22:396–402.


21. Addas-Carvalho M, Origa AF, Saad ST. Interleukin 1 beta and tumor necrosis factor levels in stored platelet concentrates and the association with gene polymorphisms. Transfusion. 2004; 44:996–1003.


23. Semple JW, Milev Y, Cosgrave D, et al. Differences in serum cytokine levels in acute and chronic autoimmune thrombocytopenic purpura: relationship to platelet phenotype and antiplatelet T-cell reactivity. Blood. 1996; 87:4245–4254.


24. Fujimoto TT, Inoue M, Shimomura T, Fujimura K. Involvement of Fc gamma receptor polymorphism in the therapeutic response of idiopathic thrombocytopenic purpura. Br J Haematol. 2001; 115:125–130.


25. Shete AR, Joseph R, Vijayan NN, Srinivas L, Banerjee M. Association of single nucleotide gene polymorphism at interleukin-1beta +3954, −511, and −31 in chronic periodontitis and aggressive periodontitis in Dravidian ethnicity. J Periodontol. 2010; 81:62–69.


26. Bhat IA, Naykoo NA, Qasim I, et al. Association of interleukin 1 beta (IL-1β) polymorphism with mRNA expression and risk of non small cell lung cancer. Meta Gene. 2014; 2:123–133.

