Abstract
Background
Immune thrombocytopenia (ITP) is the most common cause of acquired childhood thrombocytopenia and is characterized by increased immune-mediated destruction of circulating thrombocytes. Oxidative damage may be involved in ITP pathogenesis; paraoxonase (PON) and arylesterase (ARE) enzymes are closely associated with the cellular antioxidant system. We investigated the effect of short-term high-dose methylprednisolone (HDMP) treatment on the total oxidant status (TOS), total antioxidant capacity (TAC), oxidative stress index (OSI), and PON and ARE enzymatic activity in children with acute ITP.
Methods
Thirty children with acute ITP constituted the study group and 30 healthy children constituted the control group. Children with acute ITP were treated with HDMP: 30 mg/kg for 3 days, then 20 mg/kg for 4 days. The TOS, TAC, OSI, PON, and ARE levels were determined before and after 7 days of HDMP treatment.
Results
The TAC level (P<0.001), and PON (P<0.001) and ARE (P=0.001) activities were lower and the TOS (P=0.003) and OSI (P<0.001) levels were higher in children with acute ITP than those in healthy children in the control group. We also observed statistically significant increases in the TAC (P<0.01), PON (P<0.001) and ARE levels (P=0.001) and decreases in the TOS (P<0.05) and OSI levels (P<0.05) with 7 days of HDMP treatment compared to their values before treatment.
Immune thrombocytopenia (ITP) is a common childhood disorder characterized by increased destruction of circulating platelets by anti-platelet antibodies, and an increased tendency to bleed. ITP occurs in all age groups, and while most cases are considered idiopathic, some are secondary to coexisting conditions [1]. The current estimate of acute ITP incidence in children is between 1.9 and 6.4/105 children/year [2]. There have been many advances in our understanding of the disease pathophysiology, and some reports have implied that oxidative damage may play an important role [3456]. Furthermore, the disease-associated platelet destruction and bleeding may play a significant role in the elevation of lipid peroxidation and reduction in the antioxidant capacity of these patients [7].
Paraoxonase-1 (PON-1) is an antioxidant enzyme principally synthesized in the liver and is carried in the plasma in association with the high-density lipoprotein (HDL). PON has extensive hydrolytic activity, including arylesterase (ARE), phosphodiesterase, and lactonase activities [8]. In addition, due to its role in the regulation of the antioxidant activity of HDL by protecting low-density lipoproteins (LDL) against lipid peroxidation, it reduces the development of atherosclerosis [9]. It is reported that PON/ARE activity is inhibited throughout the LDL oxidation under oxidative stress [9]. Furthermore, PON activity and the apolipoprotein A-1 component of HDL are decreased in systemic or vascular inflammation [1011]. Van Lenten et al. [12] reported that HDL loses the PON activity during an acute-phase response. Also, an inverse relationship exists between serum PON activity and cardiovascular diseases [13], and PON activity decreases in cases of chronic renal failure, inflammatory arthritis, and hypercholesterolemia [14].
Oxidative stress is increased in ITP, possibly due to the systemic inflammation and platelet destruction [7], which then causes oxidation of LDL, and the enzymatic action of PON and ARE on HDL is inhibited due to the release of metabolites such as cholesterol esters and oxidized phospholipids [9]. This inhibition of enzymatic activity impairs the anti-atherogenic effects of HDL [1011], which may in turn lead to the development of atherosclerotic changes. The objective of this study was to investigate the role of oxidative stress and PON/ARE activity in the pathogenesis of acute ITP and to demonstrate how these parameters are changed during high dose methylprednisolone (HDMP) treatment. The schematic representation of the possible role of oxidative stress and PON/ARE in the pathogenesis of ITP is depicted in Fig. 1.
Thirty children with acute ITP (11 boys, 19 girls) who were treated in the pediatric hematology clinic of the Harran University Research Hospital, Turkey, between September 2009 and August 2010, were enrolled in this study. Among the patients with platelet counts <30,000/µL, a bone marrow aspiration was performed to exclude leukemia and other hematologic malignancies. The diagnostic criteria for acute ITP included normal or increased megakaryocytes in an otherwise normal bone marrow, thrombocytopenia (platelet count<30,000/µL), and absence of other causes of thrombocytopenia. The HDMP therapy was administered orally to patients with hematuria, gastrointestinal hemorrhage, gingival hemorrhage, and prolonged epistaxis, at a dose of 30 mg/kg/day for 3 days and then 20 mg/kg/day for 4 days. The hemoglobin level, platelet counts, PON and ARE activities, TOS, TAC, and OSI levels were measured before and after the 7 days of HDMP therapy. The control group consisted of 30 healthy age and sex matched children (11 boys, 19 girls) without any dietary restrictions or medicine use that could affect the serum analyses, and with platelet counts within normal range (>150,000/µL). Treatment efficacy was assessed based on clinical signs and laboratory findings. This study was approved institutional review board of Harran University (Ethics approval No: 09.06.14), and written informed consent was obtained from a parent or guardian of the child.
Children with acute or chronic infectious or inflammatory diseases, with a history of steroid usage, or who were previously treated for ITP with intravenous immunoglobulin [IVIG] or other treatment modality were excluded from the study.
Serum TOS and TAC levels were determined using a commercially available kit developed by Erel [1516]. The results are expressed as mmol Trolox Eqv/L and mmol H2O2 Eqv/L, respectively. The OSI was calculated as the percent ratio of TOS to TAC level, according to the following formula: OSI (arbitrary unit)=TOS (mmol H2O2 Eqv./L)/TAC (mmol Trolox Eqv./L)×100.
The serum PON and ARE activities were measured using commercially available kits (Rel Assay Diagnostics, Mega Tıp, Gaziantep, Turkey). The PON activity was assayed both in the absence and presence of NaCl (salt-stimulated activity). The rate of paraoxon hydrolysis (diethyl-p-nitrophenyl phosphate) was measured by monitoring the increase in absorption at 412 nm at 37℃. The amount of p-nitrophenol generated was calculated from its molar absorption coefficient at pH 8.5 (18.29 M-1cm-1) [17], and the PON activity was expressed as U/L of serum. Phenylacetate was used as a substrate to measure the ARE activity and the enzymatic activity was calculated from the molar absorption coefficient of the produced phenol (1,310 M-1cm-1). One unit of ARE activity was defined as 1 µmol of phenol generated per minute under the abovementioned conditions and was expressed as U/L [18]. complete blood cell count was performed using an automated hematology analyzer (Cell dyn 3700, Abbott Laboratories, IL, USA).
All analyses were performed using SPSS 21.0 statistical software package (SPSS for Windows 21.0; SPSS, Chicago, IL, USA). The Shapiro-Wilk test was used to test the normality of distribution for the studied variables. The mean and standard deviation (mean±SD) were calculated for normally distributed variables, and the median and interquartile ranges were calculated for all other variables. A student’s t-test and Mann-Whitney U-test were used to compare variables between the acute ITP and control group. A paired sample t-test and the Wilcoxon test were used to compare test variables before and after treatment. The Pearson’s chi-square test was used to determine whether the gender distribution differed among the study groups. The level of significance was set at <0.05 for all statistical analyses.
Clinical characteristics and laboratory parameters of the study and control group are provided in Table 1. There were no significant differences between the two groups with respect to median age, and gender distribution (P>0.05). The mean TAC levels, mean serum ARE and PON activity, and the median platelet counts were significantly lower, while the mean OSI and TOS levels were significantly higher in children with acute ITP compared with healthy children in the control group (Table 1).
The mean TAC levels (P<0.01), mean ARE (P=0.001) and PON (P<0.001) activities, and the median platelet counts (P<0.001) increased significantly after 7 days of HDMP treatment. In addition, the mean TOS (P<0.05) and OSI (P<0.05) levels reduced significantly after 7 days of treatment compared to their levels before treatment. However, the mean hemoglobin level remained unchanged (P>0.05) following HDMP treatment (Table 2 and Fig. 2, 3, 4, 5).
Although 7 days of HDMP treatment significantly modified oxidative stress indices, the mean TAC level (P<0.01), mean ARE activity (P<0.05), median platelet counts (P=0.002), and the mean OSI level (P<0.01) in the treated acute ITP-affected children could not reach the levels observed in the healthy children. However, 7 days of HDMP treatment significantly modified the mean TOS level and PON activity in acute ITP-affected children, and the post-treatment values were similar to those observed in the healthy children (Table 2). We could not identify a correlation between platelet count and other study variables before or after 7 days of HDMP treatment: TAC (before treatment: r=-0.147, P=0.44; after treatment: r=-0.13, P=0.468); TOS (before treatment: r=-0.30, P=0.876; after treatment: r=-0.20, P=0.289); OSI (before treatment: r=0.12, P=0.851; after treatment: r=-0.11, P=0.556); PON (before treatment: r=0.73, P=0.913; after treatment: r=-0.06, P=0.442); and ARE (before treatment: r=-0.21, P=0.702; after treatment: r=-0.46, P=0.744).
Primary immune thrombocytopenia is one of the most common childhood hemorrhagic disorders, and it is diagnosed by ruling out other causes of thrombocytopenia. The disease is essentially antibody mediated platelet destruction [19], and manifests as acute and rapidly occurring bruising and petechiae in previously healthy children.
ITP in children commonly occurs after a viral infection that stimulates the production of reactive oxygen species by phagocytes. Excessive production of reactive oxygen species and inadequate antioxidant capacity results in an imbalance in the redox system [20], and ITP patients are reported to have markedly higher oxidative stress levels compared to healthy individuals [3]. It has been argued that the increased oxidative stress causes oxidative changes in the surface proteins either directly or indirectly through malondialdehydes. Indeed, previous studies have reported increased malondialdehyde levels in ITP patients [67]. Moreover, proteins with modified antigenic structure are highly immunogenic and can generate an antibody response by stimulating the adaptive immune system [20].
Some previous studies have demonstrated that oxidative stress is involved in the pathogenesis of ITP. Acute ITP patients with active disease and those in remission are reported to have markedly reduced platelet antioxidant capacity [4]. A study of 84 adult chronic ITP patients showed that the patient group had a significantly lower TAC level and a high TOS level compared to the control group. Further, the patients’ platelet counts correlated positively with the TAC and negatively with TOS levels [5]. However, few studies have evaluated oxidative stress in ITP-affected children. A study by Akbayram et al. [6] on 52 pediatric ITP patients reported that the TOS and OSI levels were high, and the TAC level was low in both acute and chronic ITP patients. The study also demonstrated that in acute ITP-affected children before and after treatment, platelet counts correlated positively with TAC and negatively with OSI. We observed that the mean TAC level decreased with increase in TOS and OSI levels in our pediatric ITP patient cohort, demonstrating a decrease in the antioxidant capacity and increased oxidative stress, as has been reported in other autoimmune disorders. In their study, Akbayram et al. did not find significant differences in the oxidative stress and antioxidant capacity following steroid treatment [6]. However, our results demonstrate an increase in the mean TAC level, and a decrease in the mean TOS and OSI levels following one week of HDMP treatment in ITP-affected children. In addition, we showed that the PON and ARE enzymatic activities were significantly lower in children with ITP compared with the healthy controls. The correlation between activities of PON and ARE activities and TOS, TAC, OSI levels before or after HDMP treatment was not observed.
PON is an antioxidant responsible for the breakdown of lipid peroxides and plays an important role in the antioxidant system [21]. Studies have shown that oxidative stress can lead to reduced PON activity [2223], and the effects of antioxidant nutrients on PON have also been studied. In rat models, the PON activity was normalized following vitamin E supplementation in experimentally induced hypothyroidism [24]. The PON level was also increased after vitamin E and C supplementation in humans [25]. In a previous study, we have reported that PON and ARE activities were significantly lower in children with vitamin B12 deficiency anemia, and that they return to near-normal levels following vitamin B12 supplementation therapy [26].
We also observed a statistically significant increase in PON and ARE activity as well as TAC levels following treatment with HDMP. In a previous study investigating the effect of steroids on oxidative stress related disorders, the PON gene expression was reported to increase in patients (1–21 years old) with inflammatory bowel disorder following steroid treatment [27]. Another cell culture-based study showed that the reactive oxygen species generation was markedly reduced and PON gene expression was increased with dexamethasone treatment [28]. In addition, Sanner et al. [29] demonstrated a direct effect of dexamethasone and prednisolone on the generation of reactive oxygen species from platelets, and this inhibitory effect of both drugs was dose-dependent. In concurrence, our results suggest that high-dose steroid treatment partially reduces the oxidative stress and increases the antioxidant capacity. However, in the acute ITP-affected children treated with HDMP, the OSI level was still high and the ARE activity was still low compared to the control group at the end of our study. This may be due to the continued immune-mediated destruction of platelets in these patients.
Since ITP is a self-limiting condition, the oxidative stress may be resolved spontaneously; however, treatment is necessary in cases with severe mucosal bleeding. Probably, the HDMP therapy reduces oxidative stress, probably due to its anti-inflammatory effect, in ITP patients with severe clinical conditions requiring therapy. A previous Turkish study has shown that short-term, high-dose oral steroid treatment is more cost-effective for acute childhood idiopathic thrombocytopenic purpura than IVIG treatment, and prevents disease chronicity [30]. Therefore, HDMP treatment is the drug of choice for acute childhood idiopathic thrombocytopenic purpura.
In conclusion, PON and ARE activity levels were closely associated with the changes in the oxidative balance in pediatric ITP patients. However, we could not determine whether these changes in the antioxidant enzymes were the cause or the result of changes in the oxidative balance. Further, we could not determine whether the positive changes in the oxidative balance were a direct or indirect effect of the administered steroids. Indeed, there was no correlation between the platelet counts and the TOS, TAC, and OSI levels and the PON and ARE activities, either before or after treatment, suggesting that there may not be a direct relationship between the oxidative stress markers and platelet counts. The HDMP treatment may reduce oxidative stress independent of the improvements in the platelet counts. Our study showed that the TAC and ARE levels remained low whereas OSI levels remained high, and oxidative stress persisted in HDMP treated patients compared to the control group. In ITP, the decreased PON and ARE activities associated with increased oxidative stress may increase the risk of developing atherosclerosis. Further studies are needed to identify the acute ITP disease phase associated with the increased oxidative stress and to assess whether these patients are at a higher risk of developing atherosclerosis later in life.
References
1. Cines DB, Bussel JB, Liebman HA, Luning Prak ET. The ITP syndrome: pathogenic and clinical diversity. Blood. 2009; 113:6511–6521. PMID: 19395674.


2. Terrell DR, Beebe LA, Vesely SK, Neas BR, Segal JB, George JN. The incidence of immune thrombocytopenic purpura in children and adults: A critical review of published reports. Am J Hematol. 2010; 85:174–180. PMID: 20131303.


3. Zhang B, Lo C, Shen L, et al. The role of vanin-1 and oxidative stress-related pathways in distinguishing acute and chronic pediatric ITP. Blood. 2011; 117:4569–4579. PMID: 21325602.


4. Kamhieh-Milz J, Bal G, Sterzer V, Kamhieh-Milz S, Arbach O, Salama A. Reduced antioxidant capacities in platelets from patients with autoimmune thrombocytopenia purpura (ITP). Platelets. 2012; 23:184–194. PMID: 21913810.


5. Jin CQ, Dong HX, Cheng PP, Zhou JW, Zheng BY, Liu F. Antioxidant status and oxidative stress in patients with chronic ITP. Scand J Immunol. 2013; 77:482–487. PMID: 23551069.


6. Akbayram S, Doğan M, Akgün C, et al. The association of oxidant status and antioxidant capacity in children with acute and chronic ITP. J Pediatr Hematol Oncol. 2010; 32:277–281. PMID: 20404751.


7. Polat G, Tamer L, Tanriverdi K, Gürkan E, Baslamisli F, Atik U. Levels of malondialdehyde, glutathione and ascorbic acid in idiopathic thrombocytopaenic purpura. East Afr Med J. 2002; 79:446–449. PMID: 12638848.


8. Gaidukov L, Tawfik DS. High affinity, stability, and lactonase activity of serum paraoxonase PON1 anchored on HDL with ApoA-I. Biochemistry. 2005; 44:11843–11854. PMID: 16128586.


9. Aviram M, Rosenblat M, Billecke S, et al. Human serum paraoxonase (PON 1) is inactivated by oxidized low density lipoprotein and preserved by antioxidants. Free Radic Biol Med. 1999; 26:892–904. PMID: 10232833.


10. Lilly SM, Rader DJ. Disorders of HDL metabolism. In : Kwiterovich PO, editor. The Johns Hopkins textbook of dyslipidemia. Philadelphia, PA: Lippincott Williams & Wilkins;2010. p. 105–118.
11. Zheng C, Aikawa M. High-density lipoproteins: from function to therapy. J Am Coll Cardiol. 2012; 60:2380–2383. PMID: 23141492.
12. Van Lenten BJ, Hama SY, de Beer FC, et al. Anti-inflammatory HDL becomes pro-inflammatory during the acute phase response. Loss of protective effect of HDL against LDL oxidation in aortic wall cell cocultures. J Clin Invest. 1995; 96:2758–2767. PMID: 8675645.


13. Mackness B, Davies GK, Turkie W, et al. Paraoxonase status in coronary heart disease: are activity and concentration more important than genotype? Arterioscler Thromb Vasc Biol. 2001; 21:1451–1457. PMID: 11557671.
14. Soran H, Younis NN, Charlton-Menys V, Durrington P. Variation in paraoxonase-1 activity and atherosclerosis. Curr Opin Lipidol. 2009; 20:265–274. PMID: 19550323.


15. Erel O. A novel automated method to measure total antioxidant response against potent free radical reactions. Clin Biochem. 2004; 37:112–119. PMID: 14725941.


16. Erel O. A new automated colorimetric method for measuring total oxidant status. Clin Biochem. 2005; 38:1103–1111. PMID: 16214125.


17. Eckerson HW, Wyte CM, La Du BN. The human serum paraoxonase/arylesterase polymorphism. Am J Hum Genet. 1983; 35:1126–1138. PMID: 6316781.
18. Haagen L, Brock A. A new automated method for phenotyping arylesterase (EC 3.1.1.2) based upon inhibition of enzymatic hydrolysis of 4-nitrophenyl acetate by phenyl acetate. Eur J Clin Chem Clin Biochem. 1992; 30:391–395. PMID: 1525262.


19. D'Orazio JA, Neely J, Farhoudi N. ITP in children: pathophysiology and current treatment approaches. J Pediatr Hematol Oncol. 2013; 35:1–13. PMID: 23073045.
20. Zhang B, Zehnder JL. Oxidative stress and immune thrombocytopenia. Semin Hematol. 2013; 50:e1–e4. PMID: 23953344.


21. Précourt LP, Amre D, Denis MC, et al. The three-gene paraoxonase family: physiologic roles, actions and regulation. Atherosclerosis. 2011; 214:20–36. PMID: 20934178.


22. Selek S, Aslan M, Horoz M, Gur M, Erel O. Oxidative status and serum PON1 activity in beta-thalassemia minor. Clin Biochem. 2007; 40:287–291. PMID: 17296173.


23. Cakmak A, Soker M, Koc A, Erel O. Paraoxonase and arylesterase activity with oxidative status in children with thalassemia major. J Pediatr Hematol Oncol. 2009; 31:583–587. PMID: 19636263.


24. Sarandöl E, Taş S, Dirican M, Serdar Z. Oxidative stress and serum paraoxonase activity in experimental hypothyroidism: effect of vitamin E supplementation. Cell Biochem Funct. 2005; 23:1–8. PMID: 15386442.


25. Jarvik GP, Tsai NT, McKinstry LA, et al. Vitamin C and E intake is associated with increased paraoxonase activity. Arterioscler Thromb Vasc Biol. 2002; 22:1329–1333. PMID: 12171796.


26. Koc A, Cengiz M, Ozdemir ZC, Celik H. Paraoxonase and arylesterase activities in children with iron deficiency anemia and vitamin B12 deficiency anemia. Pediatr Hematol Oncol. 2012; 29:345–353. PMID: 22568797.
27. Alkhouri RH, Baker SS, Hashmi H, Liu W, Baker RD, Zhu L. Paraoxonase gene expression in pediatric inflammatory bowel disease. J Clin Cell Immunol. 2014; 5:224.


28. Lim JA, Kim SH. Transcriptional activation of an anti-oxidant mouse Pon2 gene by dexamethasone. BMB Rep. 2009; 42:421–426. PMID: 19643039.


29. Sanner BM, Meder U, Zidek W, Tepel M. Effects of glucocorticoids on generation of reactive oxygen species in platelets. Steroids. 2002; 67:715–719. PMID: 12117619.


30. Ozsoylu S, Sayli TR, Oztürk G. Oral megadose methylprednisolone versus intravenous immunoglobulin for acute childhood idiopathic thrombocytopenic purpura. Pediatr Hematol Oncol. 1993; 10:317–321. PMID: 8292515.
Fig. 1
The possible role of oxidative stress and paraoxonase (PON)/arylesterase (ARE) in the pathogenesis of acute immune thrombocytopenia. Following a triggering event such as infection or inflammation, antibody-coated platelets are destroyed by macrophages in the spleen and liver. The released foreign peptide fragments are presented to the T- and B-lymphocytes. While antibody synthesis continues with the stimulation of the B-lymphocytes, the peripheral thrombocyte destruction continues due to the cytotoxic T-lymphocytes activation. During the destruction of platelets in the reticuloendothelial system and the periphery, reactive oxygen species (ROS) are produced. Increasing ROS levels induces a decrease in the total antioxidant capacity (TAC) and an increase in the total oxidant status (TOS). As a result of this increased oxidative stress, deoxyribonucleic acid (DNA), protein, and lipid peroxidation ensues. Lipid peroxidation leads to formation of oxidized low-density lipoproteins (LDL). The released oxidized phospholipids and cholesterol esters interact with the free sulfhydryl groups of paraoxonase (PON), and inhibit the PON and arylesterase (ARE) activities. In addition, the PON activity is also reduced due to systemic inflammation. As a result, dysfunctional high-density lipoproteins (HDL) with impaired anti-atherogenic effects are produced. This impaired anti-atherogenic effect of HDL may increase the tendency to atherosclerosis.
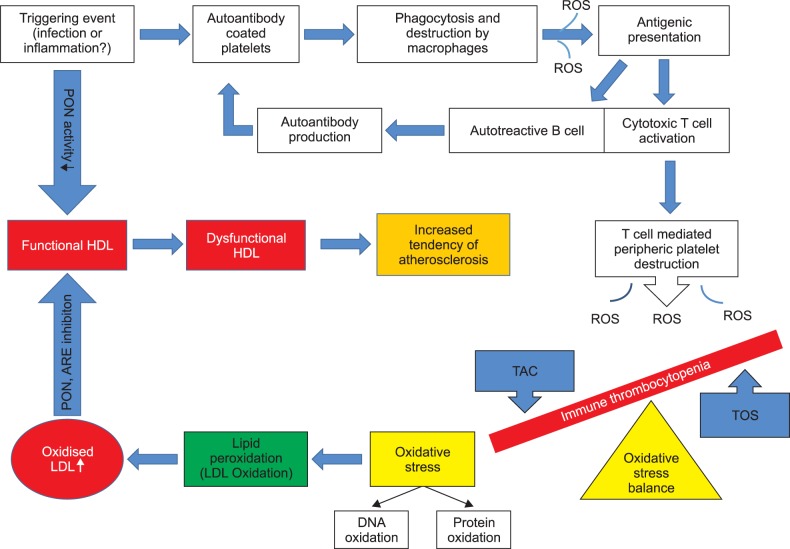
Fig. 2
Boxplot graph of TAC levels before and 7 days of after treatment in the ITP group and control group.
Abbreviations: TAC, total antioxidant capacity; ITP, Immune thrombocytopenia.
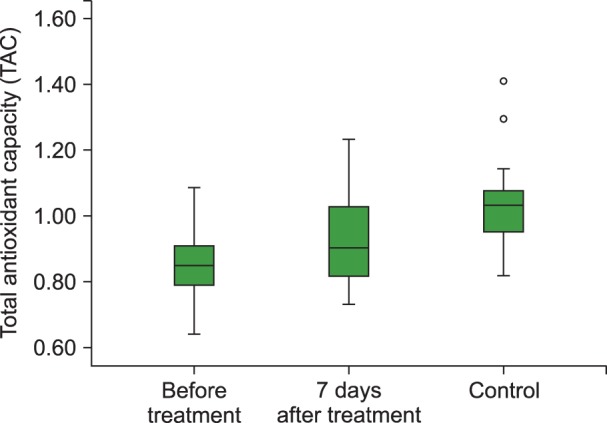
Fig. 3
Boxplot graph of OSI levels before and 7 days of after treatment in the ITP group and control group.
Abbreviations: OSI, oxidative stress index; ITP, Immune thrombocytopenia.
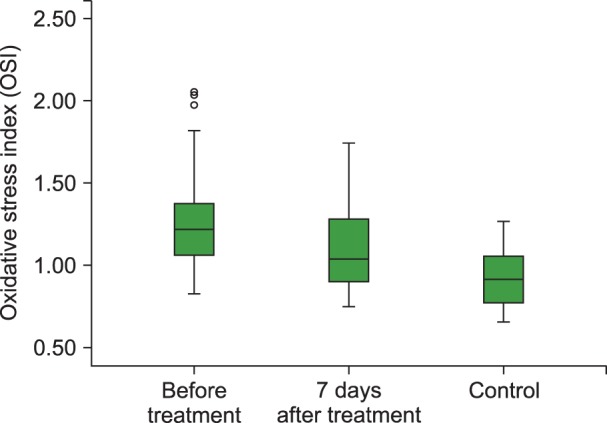
Fig. 4
Boxplot graph of PON levels before and 7 days of after treatment in the ITP group and control group.
Abbreviations: PON, paraoxonase; ITP, Immune thrombocytopenia.
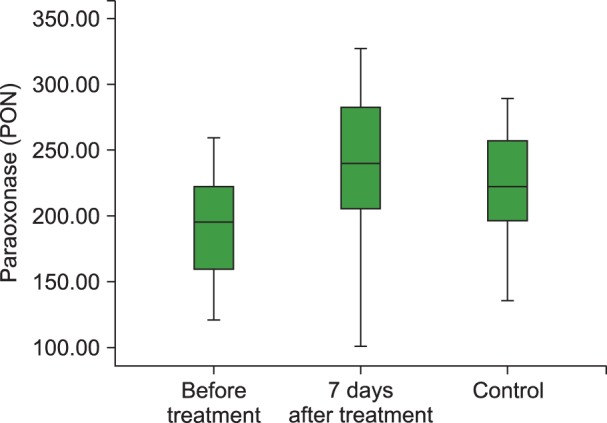
Fig. 5
Boxplot graph of ARE levels before and 7 days of after treatment in the ITP group and control group.
Abbreviations: ARE, arylesterase; ITP, Immune thrombocytopenia.
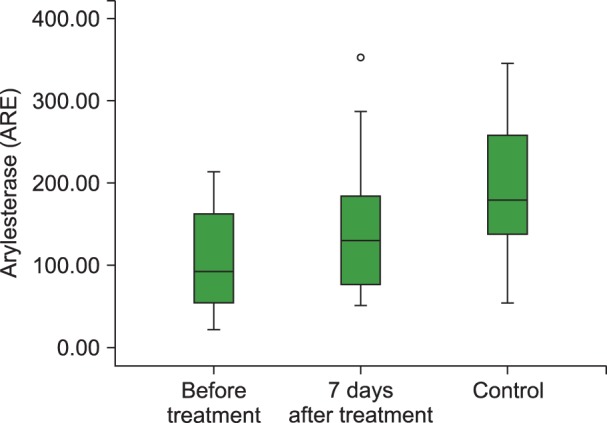
Table 1
Clinical and laboratory parameters associated with the cellular antioxidant system in the children with acute ITP and control group.
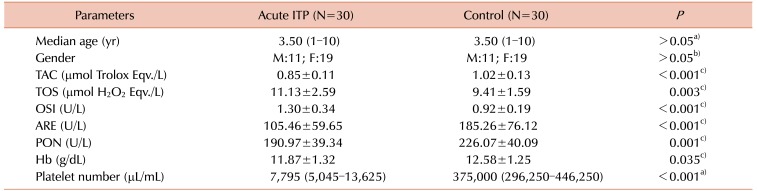
Table 2
Laboratory parameters associated with the cellular antioxidant system of children with acute ITP before and after HDMP treatment.
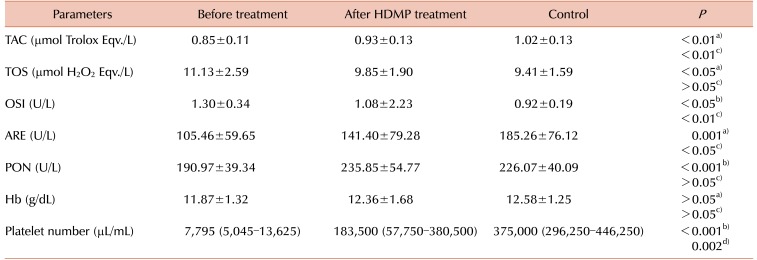
a)Paired sample test, b)Wilcoxon test, acute ITP before treatment vs. after 7 days of treatment, c)Independent sample test, d)Mann-Whitney test, acute ITP after 7 days of treatment vs. control group.
Abbreviations: HDMP, methylprednisolone; TAC, total antioxidant capacity; TOS, total oxidant status; OSI, oxidative stress index; ARE, arylesterase; PON, paraoxonase; Hb, hemoglobin.