Abstract
Hemophagocytic lymphohistiocytosis (HLH) is a life-threatening hyperinflammatory clinical syndrome of uncontrolled immune response which results in hypercytokinemia due to underlying primary or secondary immune defect. A number of genetic defects in transport, processing and function of cytotoxic granules which result in defective granule exocytosis and cytotoxicity of cytotoxic T lymphocytes (CTL) and natural killer (NK) cells have been well identified at the cellular and molecular level. Important advances have been made during the last 20 years in the diagnosis and treatment of HLH. The Histiocyte Society has proposed diagnostic guideline using both clinical and laboratory findings in HLH-2004 protocol, and this has been modified partly in 2009. HLH used to be a fatal disease, but the survival of HLH patients has improved to more than 60% with the use of chemoimmunotherapy combined with hematopoietic cell transplantation (HCT) over the past 2 decades. However, HCT is still the only curative option of treatment for primary HLH and refractory/relapsed HLH after proper chemoimmunotherapy. The outcome of HCT for HLH patients was also improved steadily during last decades, but HCT for HLH still carries significant mortality and morbidity. Moreover, there remain ongoing controversies in various aspects of HCT including indication of HCT, donor selection, timing of HCT, conditioning regimen, and mixed chimerism after HCT. This review summarized the important practical issues which were proven by previous studies on HCT for HLH, and tried to delineate the controversies among them.
HLH is a life-threatening hyperinflammatory clinical syndrome of uncontrolled immune response which results in hypercytokinemia due to underlying primary or secondary immune defect. HLH can be classified into genetic (primary) and acquired (secondary) forms according to the underlying defect. The primary HLH can be categorized into 2 subgroups, one including 5 subtypes of familial HLH (FHL), and the other expanding subgroup of separate primary immune deficiencies including Chediak-Higashi syndrome (CHS), Griscelli syndrome type 2 (GS2), Hermansky-Pudlak syndrome and X-linked lymphoproliferative (XLP) syndrome 1 and 2 (XLP1, XLP2) [1234567] (Table 1).
A number of genetic defects in transport, processing and function of cytotoxic granules which result in defective granule exocytosis and cytotoxicity of natural killer cells and cytotoxic T lymphocytes have been well identified at the cellular and molecular level [45678910] (Fig. 1). The acquired forms of HLH develop due to a variety of underlying conditions and are more frequent than genetic forms. It is reported that more than 90% of children with HLH were acquired HLH in a nationwide survey in Japan. The most common triggering causes of acquired HLH are infections, autoimmune diseases, and malignancies [45678911].
Important advances have been made during the last 20 years in the diagnosis and treatment of HLH. The Histiocyte Society has proposed diagnostic guideline using both clinical and laboratory findings in HLH-2004 protocol [12], and this has been modified partly in 2009. HLH can be diagnosed by genetic confirmation only with molecular diagnosis consistent with FLH or XLP, or clinically if at least 3 fulfilled out of 4 major symptoms and signs including fever, splenomegaly, cytopenias (at least 2 cell lines), hepatitis, and also at least 1 fulfilled out of 4 laboratory findings including hemophagocytosis, increased ferritin, increased sIL2Rα, absent or very low NK cell function. Hypertriglyceridemia, hypofibrinogenemia, and hyponatremia are also regarded as supportive evidences of HLH diagnosis [13].
The strategies for proper treatment of HLH should include countermeasures for suppression of the hyperinflammation, elimination of triggering causes, killing of infected cells, and replacing defective immune system for patients with genetic disorders. HLH used to be a fatal disease with 1-year overall survival of only 5% [14], but the survival of HLH patients has improved to more than 60% with the use of chemoimmunotherapy combined with HCT over the past 2 decades. However, HCT is still the only curative option of treatment for primary HLH and refractory/relapsed HLH after chemoimmunotherapy. The outcome of HCT for HLH patients has improved steadily during past decades, but HCT for HLH still carries significant mortality and morbidity and there remains controversies in various aspects of HCT [151617181920]. The purpose of this review is to summarize the facts which were proven by previous studies on HCT for HLH and to delineate the future perspectives in the field of HCT for HLH.
The first successful allogeneic HCT performed for the treatment of HLH was reported by Fischer et al. in 1986 [21], and the introduction of HCT has dramatically improved the prognosis of the disease thereafter. The Histiocyte Society introduced VP-16 and HCT into the HLH-94 protocol, and these are regarded as the main reasons for the successful improvement in the overall survival around 66±8% of HLH patients who were treated with this protocol. In HLH-94, HCT was recommended for patients with familial, persistent, or recurrent disease, and conventional myeloablative conditioning (MAC) was suggested [22]. Chemoimmunotherapy is only temporarily efficient in the control of FLH, and the outcome is uniformly lethal unless the patient undergoes HCT [1223].
Since 2005, different series of clinical studies have been published on the outcome of HCT for HLH patients reporting 5-year overall survival rates of 50-70% with MAC [182425262728], and 75-92% with reduced intensity conditioning (RIC) [293031]. While the overall survival was increased with HCT using MAC (MAC-HCT), this approach revealed high early transplant-related mortality (TRM) within post-transplant 100 days due to multifactorial causes such as high incidence of veno-occlusive disease (VOD) and pneumonia, and high incidence of primary non-engraftment about 9-22%.
Overall survival rate of the HLH patients not in remission status at the time of HCT was reported to be inferior than that of patients in remission, thus revealing the importance of optimal disease control before HCT [23]. The superior overall survival rate of HCT using RIC (RIC-HCT) regimen for HLH patients compared to MAC were reported by various group since 2006. As the data of RIC-HCT for HLH patients accumulates, various aspects regarding HCT need to be clarified such as indication of HCT, donor selection, timing of HCT, optimal conditioning regimen, and mixed chimerism (MC) after HCT.
HCT is generally recommended in patients with documented FHL, recurrent or progressive HLH despite recommended chemoimmunotherapy, and CNS involvement [32].
The HLH-2004 protocol recommends HCT for the patients who have genetically verified or familial disease, and for patients with non-familial disease which is severe and persistent or reactivated after 8 weeks of initial therapy. The reactivation of HLH can be diagnosed if the patients have achieved a remission, and then again develop 3 or more of the HLH diagnostic criteria proposed in HLH-2004 protocol.
HCT is mandatory in the treatment of XLP and FHL patients and is the treatment of choice for refractory cases of EBV associated HLH [283334]. HCT should only be performed in patients with GS2 who have a mutation of the RAB27A gene, since only these patients will develop immunodeficiencies and early lymphohistiocytic infiltrates [35].
Selection of optimal stem cell donor and source is important for HLH patients undergoing HCT. It has been reported that the outcome of HCT using matched unrelated donor is comparable to that of HCT using matched sibling donor for HLH patients [181927]. HCT using haploidentical donor for HLH patients who do not have matched donor was reported to be feasible, and the outcome is reported to be improving [3136]. There had been conflicting data regarding the cord blood transplantation (CBT) for HLH.
Japanese group reported comparable outcome of HCT using cord blood stem cells for HLH patients with that of HCT using matched unrelated donor. FHL patients showed either an equal or better outcome even after CBT compared with the recent reports. This study asserted that cord blood might therefore be acceptable as an alternate HCT source for HLH patients, although the optimal conditioning remains to be determined [17]. On the other hand, Korean group reported poorer outcome with CBT [37], and one definite drawback of CBT is that the cases of CBT lack source for donor lymphocyte infusion (DLI) when they show dwindling donor chimerism. Therefore it is necessary for the experts of HLH to consider their institution's experience in HCT when selecting the optimal donor for HCT, as there is considerable center effect.
The possibility of the sibling carrying the disease should be considered when searching for an HCT donor and all family members should be screened for FHL as there are cases with late-onset FHL. To use matched sibling donor for HCT of HLH patients, the donor needs to be confirmed with genetic study and/or NK cell activity test. HLA-matched heterozygous carrier sibling is acceptable as a donor for HCT, and usually these is no relevant dominant negative effect.
As HCT is indicated in all the patients with primary HLH, it is necessary to start donor search as soon as possible if the diagnosis of primary HLH is made [32]. Rapid identification of genetic immune defects allows differential diagnosis from secondary HLH, thereby enabling early HCT [38].
Patients with active HLH at the time of HCT generally have worse outcomes compared to patients with inactive disease, as active disease increases graft failure and thereby decreases overall survival rate [161827]. Complete responses to conventional HLH therapies are only observed in 50-75% of patients, and the only independent association with improved survival is inactive disease after 2 months of HLH-94 therapy [1939]. Therefore optimal control of disease before HCT is important, and some delay in HCT to treat residual disease optimally is justified. Serial monitoring of soluble CD25 is one of the most useful clinical markers of disease activity in the pre-transplant period, and it can give helpful information for the optimal timing of HCT [23].
However, the best treatment strategy for the reactivated patients before HCT or for patients with residual CNS abnormalities after chemoimmunotherapy is not well established until now, and usually reintensification of treatment before HCT is used to achieve a remission. These subgroup of patients might have the chance of successful HCT, and CNS disease may become permanently controlled after successful HCT [40].
Conventional MAC-HCT mostly adopted busulfan, cylophosphamide, etoposide with or without ATG. However, it is repeatedly reported that the outcome of MAC-HCT for HLH patients revealed high early TRM due to multifactorial causes and high incidence of primary non-engraftment around 9-22%. Patients with XIAP deficiency were found to have poor tolerance to MAC [41]. This increased TRM after MAC-HCT has prompted the use of less toxic approach, RIC-HCT. RIC-HCT has been most extensively studied in patients with nonmalignant disorders and sufficient data now exist for HLH to support its routine use even in patients without comorbidity. The goals of a RIC regimen are to prevent graft rejection and to establish stable donor-derived hematopoiesis at a level sufficient for cure of the underlying disease. RIC regimens have also enabled HCT to be performed in children with preexisting comorbidities that preclude conventional conditioning [42].
RIC with alemtuzumab, fludarabine, and melphalan has demonstrated better outcomes in HCT, and its use is increasing [2943]. Fludarabine-based conditioning regimen including melphalan and alemtuzumab or ATG is one of the widely used RIC regimens for HLH patients, and various modifications of this combination were reported. Marsh et al. reported a 3-year survival rate of 92% in their RIC cohort, compared with 43% in their MAC cohort. However, high incidences of MC and graft loss are significant concerns after RIC-HCT. The transplant physicians treating HLH should be well aware of the countermeasures in these circumstances [43].
Alemtuzumab is a humanized recombinant monoclonal antibody directed against CD52 on lymphocytes, and it targets both activated T cell and macrophages. It is frequently included in RIC regimens as it is an effective immunosuppressant. Alemtuzumab persists at lympholytic concentration for 1-2 months after infusion, and it can exert effects on both the recipient and the graft due to this long pharmacologic half-life. Administration of alemtuzumab distal to the stem cell infusion date (beginning about day -21), likely produces predominant effects on the recipient, with decreased risk of graft rejection but less effect on graft-versus-host disease (GVHD) prevention. Proximal administration (beginning about day -9) may result in more circulating drug after stem cell infusion and in vivo T-cell depletion of the graft, thereby resulting in reduction of GVHD, increased risk of graft failure, and impaired immune reconstitution. Intermediate administration (beginning day -13) may balance these risks and benefits. Cincinnati group recently reported that intermediate RIC reduces the risk of MC, is associated with a low incidence of acute GVHD, and decreases the need for additional hematopoietic cell products after HCT [434445]. Although the RIC approach appears promising, more follow-up in larger cohort of HLH patients undergoing HCT is needed to ascertain the long-term outcome [5].
Conditioning regimens with reduced toxicity based on melphalan or treosulfan are promising alternatives. It is reported that fludarabine, treosulfan, alemtuzumab, and thiotepa represent a conditioning regimen with a high rate of disease-free survival and low toxicity in the high risk group of patients with hereditary HLH [46]. RIC-HCT using a haploidentical donor was reported to sufficiently restore immune regulation in infants with FHL, while decreasing TRM and long-term sequelae [47]. Japanese group reported the feasibility of RIC in unrelated CBT for patients with primary HLH using melphalan, fludarabine and anti-lymphocyte globulin or anti-thymocyte globulin [4849]. Choice of conditioning regimen when CBT is the only option should be carefully considered, as the lack of source of DLI is a significant concern due to the high incidence of MC after RIC-HCT [43].
MAC-HCT using busulfan, cyclophosphamide, and etoposide with or without ATG has long been the standard of care for patients with HLH. However, MAC-HCT is associated with high TRM (30-50%), and main causes of early TRM include infections, VOD, pneumonitis, graft failure, and GVHD [121824252627282950].
As TRM may be due to the incomplete control of HLH before HCT in some patients, every effort must be made to achieve remission of underlying FHL before HCT. However, significant TRM has also been noted in patients with apparently good control of underlying FHL before HCT. It is possible that occult liver or lung damage from HLH may predispose FHL patients to high rates of VOD or pneumonitis when treated with a busulfan-based MAC. A significant number of early deaths before post-transplant 100 days are attributed to HLH reactivation. The patients who survived 100 days after HCT with durable engraftment usually experience long-term disease free survival [1723253051]. Most deaths occur in the first year post-transplantation, and the likelihood of relapse is limited after the second year [2032].
Approximately half of patients with HLH treated with RIC, however, experience MC, which may be unstable in the early post-transplant months, and raises concerns for subsequent HLH relapse and graft loss. This may be due to the inclusion of alemtuzumab in the preparative regimen, as a significant effect of timing of alemtuzumab was noted upon the incidence of MC. Rapid immunosuppression withdrawal, donor lymphocyte infusion (DLI), or stem cell boost are frequently employed interventions to prevent MC progression, graft loss, and need for second transplant. However further study in larger cohort of patients are needed to establish consensus approach in the optimal dosing, timing, and efficacy of these interventions [435152].
Murine studies and clinical observations suggested that a stable chimerism with 10-20% donor cells may be sufficient for correction of the underlying condition in a variety of non-malignant conditions. Mixed hematopoietic or T-cell chimerism above a minimal threshold restores perforin-dependent immune regulation in perforin-deficient mice [453]. It appears that whole blood donor chimerism above 10-20% does indeed act against HLH, as HLH relapse was noted only after donor chimerism fell to less than 10%. Frequent monitoring of chimerism studies is essential, and centers should be prepared to stabilize donor chimerism with early withdrawal of GVHD prophylaxis or administration of DLI if needed [43].
The timing of alemtuzumab administration is closely linked to underlying disease, thereby making it difficult to draw definitive conclusions regarding the influence of timing on development of MC and graft loss. Distal administration of alemtuzumab in patients with non-malignant diseases resulted in a low incidence of MC and graft loss supporting this dosing strategy in this subset [445254].
Decisions regarding DLI should currently be based on patient chimerism trends, availability of DLI product, active infections, history of GVHD, and other factors. DLI is often recommended when donor contribution to MC is rapidly or persistently declining during the early post-HCT period towards thresholds that cause concern for eventual HLH relapse and graft loss. The patients with trends of decreasing donor contribution to hematopoiesis to levels lower than 40-60% within the first 6 months post-transplant need to be considered for DLI at this point so as not to further decrease to levels below 20%. As patients with earlier onset or more rapid decline of donor chimerism appear to be at higher risk of graft loss or HLH recurrence, the onset and rate of decline of donor chimerism are also to be considered [45].
The overall estimated 3-year survival who received HCT according to HLH-94 protocol was 64%. The best results following MAC have been achieved when HLA-matched related or unrelated donors were used, and CNS disease was absent or quiescent at the time of HCT. The best results with HCT have been observed in children who achieved prompt and complete response to chemoimmunotherapy prior to HCT. Thus the disease state after initial treatment, the stem cell source of the transplant, and the donor type were the important prognostic factors that affected the overall survival of the HLH patients who underwent HCT [272829303151].
It is also reported in a multivariate analysis that a younger age at diagnosis, severe transaminasemia, and a coagulation abnormality were also other independent prognostic factors for survival [55]. Ohga et al. reported that EBV-HLH patients showed a better prognosis after HCT than FHL patients in their series of patients [1733]. Children with HLH may have long-term significant cognitive and psychosocial impairments, even after a successful HCT. These impairments can occur despite no obvious neurologic involvement with the disease process at diagnosis [56]
HCT-HLH is thought to develop more often than previously suspected, because the number of HLA-mismatched HCT is currently increasing. A prospective observational study on HCT-HLH with 171 patients who underwent HCT reported a relatively high incidence (8.8%) after allogeneic HCT [57].
Because it is difficult to use immunosuppressive or cytoreductive agents in the immune-compromised stage within the first 30 days after HCT, the criteria for HCT-HLH in the early-onset group need to be stringent. In patients who develop a sustained fever without an apparent causative infection in early post-transplant period, HCT-HLH should be included in the differential diagnosis. Very high levels of serum ferritin, soluble IL-2 receptor, and the presence of hemophagocytosis are findings suggestive of HLH. HLH directed therapy should be considered for these patients, and early treatment with low-dose VP-16 appears to be a successful approach for early-onset HCT-HLH. [5859]
Mutations in STXBP2 affect not only cytotoxic T lymphocytes but also cause changes in the intestinal and renal epithelium resulting in severe, osmotic diarrhea and renal proximal tubular dysfunction. A severe, life-threatening form of enteropathy may precede the typical features of FHL, and FHL5 must also be considered as a differential diagnosis in patients with intractable diarrhea. As these defects persist after successful treatment of HLH by HCT, FHL5 patients who received otherwise successful HCT in their childhood may suffer from gastrointestinal, nephrological, neurological and other non-hematological disorders. Clinical manifestations in FHL5 patients despite successful HCT may therefore be related to defective membrane trafficking in the gut and kidney [60].
CHS, GS2, and XLP are diseases showing similar immunological manifestations, and predispose to a fulminant HLH. Treatment delay increases the risk of fatal outcome and may increase the risk for neurological complications. HLH treatment can be an effective first line treatment to induce remission in patients with CHS, GS2, and XLP [61].
The most effective treatment for the hematologic and immune defects in patients with CHS is HCT, despite no evidence of efficacy in delaying or preventing progressive neurologic dysfunction. Although comparative trials are lacking, RIC may be better than MAC with regard to toxicity and overall survival. A prompt diagnosis of the early-onset form of CHS, with special emphasis on the molecular characterization and analysis of CTL cytotoxicity, might help identify patients with a high risk of developing HLH. Patients with absent CTL cytotoxicity might need early HCT because of their high risk of developing HLH. Normal CTL cytotoxicity or bi-allelic missense mutations do not exclude the development of HLH, but a more conservative approach is justified [62].
HCT is an efficient treatment for curing the immune disorder of GS2. Patients with GS2 should urgently be treated with HCT after diagnosis for the risk of HLH. Neurologic HLH before HCT is a major concern, and the neurologic sequelae after otherwise successful HCT are not uncommon. Considering the high observed toxicity of HCT in GS2, RIC might be recommended. Improvement of the transplantation procedure is needed to reduce its toxic effects, and diagnosis should be made as early as possible to reduce long-term, post-transplant neurologic sequelae [6364].
XLP1 is a rare immunodeficiency characterized by severe immune dysregulation and caused by mutations in the SH2D1A/SAP gene. Clinical manifestations are varied including HLH, lymphoma and dysgammaglobulinemia, often triggered by Epstein-Barr virus infection. HLH still remains the most severe feature of XLP1, and HCT should be undertaken in all patients with HLH as the outcome without transplant is extremely poor. The outcome of HCT for other manifestations of XLP1 is very good, and patients must be monitored closely for evidence of disease progression if HCT is not undertaken [65].
Marsh et al. reported that RIC consisting of alemtuzumab, fludarabine, and melphalan is a feasible approach for patients with XLP1, and showed good outcomes in most patients with no relapses of HLH or lymphoma observed in their series. RIC should especially be considered for patients with XLP1 who have a history of HLH [66].
Patients with XLP2 were found to have poor tolerance to MAC, and the value of HCT in XIAP deficiency is an ongoing matter of debate. It is reported that conditioning regimen and HLH activity affected outcome, and the survival after RIC-HCT of patients in remission from HLH was 86%. In this report, the survival after MAC was quite poor, and the authors concluded that MAC should not be used for patients with XIAP deficiency. RIC regimens should be pursued with caution and, if possible, efforts should be made to achieve HLH remission before HCT in these patients [41]. However, the good long-term outcome in several patients who didn't receive HCT, a confirmed low risk of lymphoma development, and the reported high complication rate of HCT in this disease certainly warrants general caution with HCT. [416768].
It was reported that very young HLH patients who are at high risk of fatal FHL with persistently deficient NK activity and/or overt CNS disease require appropriate HCT to reverse CNS disease and achieve a complete cure. Most of the HLH patients with CNS involvement surviving HCT show improvement in neurologic function and cognitive development, and long-term follow-up reveal a normal or near-normal quality of life in most of them. These results strongly indicate the efficacy of HCT for primary HLH patients with CNS disease. Late complications of prior CNS damage can manifest months to years after HCT with neurocognitive deficits [13326970]. It is also reported that notable response to the therapy with reduction of the CNS lesions and normalization of the brain metabolite detected by MR spectroscopy could be observed after successful HCT [71].
As there have been considerable accumulations of data with regard to the various uncertain issues and controversies in HCT for HLH, it is more and more expected that the outcome of HCT for these patients might be significantly improved in in near future.
With the increased use of whole genome sequencing approaches, it is expected that the genetic and molecular bases of the remaining unknown familial cases of hereditary HLH will soon be elucidated [6].
Novel treatment modalities, such as gene therapy for correction of perforin defects, are currently under evaluation. Preliminary data on perforin gene transfer into hematopoietic stem cells of a mouse model of perforin-deficiency have shown encouraging results of improving immune dysregulation [7273].
Together with the improvements in the HCT for patients with HLH, ongoing efforts to improve the pre-transplant treatment of HLH will also improve the outcome of HCT. More data through clinical trials of salvage therapies and second-line treatment are needed to improve HCT outcomes.
References
1. Sieni E, Cetica V, Hackmann Y, et al. Familial hemophagocytic lymphohistiocytosis: when rare diseases shed light on immune system functioning. Front Immunol. 2014; 5:167. PMID: 24795715.


2. Filipovich AH. The expanding spectrum of hemophagocytic lymphohistiocytosis. Curr Opin Allergy Clin Immunol. 2011; 11:512–516. PMID: 21971331.


3. Janka GE, Lehmberg K. Hemophagocytic syndromes-an update. Blood Rev. 2014; 28:135–142. PMID: 24792320.
4. Janka GE, Lehmberg K. Hemophagocytic lymphohistiocytosis: pathogenesis and treatment. Hematology Am Soc Hematol Educ Program. 2013; 2013:605–611. PMID: 24319239.


5. Chandrakasan S, Filipovich AH. Hemophagocytic lymphohistiocytosis: advances in pathophysiology, diagnosis, and treatment. J Pediatr. 2013; 163:1253–1259. PMID: 23953723.


6. Bode SF, Lehmberg K, Maul-Pavicic A, et al. Recent advances in the diagnosis and treatment of hemophagocytic lymphohistiocytosis. Arthritis Res Ther. 2012; 14:213. PMID: 22682420.


8. Tang YM, Xu XJ. Advances in hemophagocytic lymphohistiocytosis: pathogenesis, early diagnosis/differential diagnosis, and treatment. ScientificWorldJournal. 2011; 11:697–708. PMID: 21442147.


9. Gholam C, Grigoriadou S, Gilmour KC, Gaspar HB. Familial haemophagocytic lymphohistiocytosis: advances in the genetic basis, diagnosis and management. Clin Exp Immunol. 2011; 163:271–283. PMID: 21303357.


10. Zhao XW, Gazendam RP, Drewniak A, et al. Defects in neutrophil granule mobilization and bactericidal activity in familial hemophagocytic lymphohistiocytosis type 5 (FHL-5) syndrome caused by STXBP2/Munc18-2 mutations. Blood. 2013; 122:109–111. PMID: 23687090.


11. Ishii E, Ohga S, Imashuku S, et al. Nationwide survey of hemophagocytic lymphohistiocytosis in Japan. Int J Hematol. 2007; 86:58–65. PMID: 17675268.


12. Henter JI, Horne A, Aricó M, et al. HLH-2004: Diagnostic and therapeutic guidelines for hemophagocytic lymphohistiocytosis. Pediatr Blood Cancer. 2007; 48:124–131. PMID: 16937360.


13. Filipovich AH. Hemophagocytic lymphohistiocytosis (HLH) and related disorders. Hematology Am Soc Hematol Educ Program. 2009; 127–131. PMID: 20008190.


14. Janka GE. Familial hemophagocytic lymphohistiocytosis. Eur J Pediatr. 1983; 140:221–230. PMID: 6354720.


15. Aricò M, Janka G, Fischer A, et al. FHL Study Group of the Histiocyte Society. Hemophagocytic lymphohistiocytosis. Report of 122 children from the International Registry. Leukemia. 1996; 10:197–203. PMID: 8637226.
16. Baker KS, Filipovich AH, Gross TG, et al. Unrelated donor hematopoietic cell transplantation for hemophagocytic lymphohistiocytosis. Bone Marrow Transplant. 2008; 42:175–180. PMID: 18454181.


17. Ohga S, Kudo K, Ishii E, et al. Hematopoietic stem cell transplantation for familial hemophagocytic lymphohistiocytosis and Epstein-Barr virus-associated hemophagocytic lymphohistiocytosis in Japan. Pediatr Blood Cancer. 2010; 54:299–306. PMID: 19827139.


18. Ouachée-Chardin M, Elie C, de Saint Basile G, et al. Hematopoietic stem cell transplantation in hemophagocytic lymphohistiocytosis: a single-center report of 48 patients. Pediatrics. 2006; 117:e743–e750. PMID: 16549504.
19. Henter JI, Samuelsson-Horne A, Aricò M, et al. Treatment of hemophagocytic lymphohistiocytosis with HLH-94 immunochemotherapy and bone marrow transplantation. Blood. 2002; 100:2367–2373. PMID: 12239144.


20. Naithani R, Asim M, Naqvi A, et al. Increased complications and morbidity in children with hemophagocytic lymphohistiocytosis undergoing hematopoietic stem cell transplantation. Clin Transplant. 2013; 27:248–254. PMID: 23331022.


21. Fischer A, Cerf-Bensussan N, Blanche S, et al. Allogeneic bone marrow transplantation for erythrophagocytic lymphohistiocytosis. J Pediatr. 1986; 108:267–270. PMID: 3511206.


22. Trottestam H, Horne A, Aricò M, et al. Chemoimmunotherapy for hemophagocytic lymphohistiocytosis: long-term results of the HLH-94 treatment protocol. Blood. 2011; 118:4577–4584. PMID: 21900192.


23. Jordan MB, Filipovich AH. Hematopoietic cell transplantation for hemophagocytic lymphohistiocytosis: a journey of a thousand miles begins with a single (big) step. Bone Marrow Transplant. 2008; 42:433–437. PMID: 18679369.


24. Baker KS, DeLaat CA, Steinbuch M, et al. Successful correction of hemophagocytic lymphohistiocytosis with related or unrelated bone marrow transplantation. Blood. 1997; 89:3857–3863. PMID: 9160694.


25. Cesaro S, Locatelli F, Lanino E, et al. Hematopoietic stem cell transplantation for hemophagocytic lymphohistiocytosis: a retrospective analysis of data from the Italian Association of Pediatric Hematology Oncology (AIEOP). Haematologica. 2008; 93:1694–1701. PMID: 18768529.


26. Dürken M, Horstmann M, Bieling P, et al. Improved outcome in haemophagocytic lymphohistiocytosis after bone marrow transplantation from related and unrelated donors: a single-centre experience of 12 patients. Br J Haematol. 1999; 106:1052–1058. PMID: 10520013.


27. Horne A, Janka G, Maarten Egeler R, et al. Haematopoietic stem cell transplantation in haemophagocytic lymphohistiocytosis. Br J Haematol. 2005; 129:622–630. PMID: 15916685.


28. Imashuku S, Hibi S, Todo S, et al. Allogeneic hematopoietic stem cell transplantation for patients with hemophagocytic syndrome (HPS) in Japan. Bone Marrow Transplant. 1999; 23:569–572. PMID: 10217187.


29. Cooper N, Rao K, Gilmour K, et al. Stem cell transplantation with reduced-intensity conditioning for hemophagocytic lymphohistiocytosis. Blood. 2006; 107:1233–1236. PMID: 16219800.


30. Malinowska I, Machaczka M, Popko K, Siwicka A, Salamonowicz M, Nasiłowska-Adamska B. Hemophagocytic syndrome in children and adults. Arch Immunol Ther Exp (Warsz). 2014; 62:385–394. PMID: 24509696.


31. Cooper N, Rao K, Goulden N, Webb D, Amrolia P, Veys P. The use of reduced-intensity stem cell transplantation in haemophagocytic lymphohistiocytosis and Langerhans cell histiocytosis. Bone Marrow Transplant. 2008; 42(Suppl 2):S47–S50. PMID: 18978744.


32. Jordan MB, Allen CE, Weitzman S, Filipovich AH, McClain KL. How I treat hemophagocytic lymphohistiocytosis. Blood. 2011; 118:4041–4052. PMID: 21828139.


33. Imashuku S. Clinical features and treatment strategies of Epstein-Barr virus-associated hemophagocytic lymphohistiocytosis. Crit Rev Oncol Hematol. 2002; 44:259–272. PMID: 12467966.


34. Imashuku S, Tabata Y, Teramura T, Hibi S. Treatment strategies for Epstein-Barr virus-associated hemophagocytic lymphohistiocytosis (EBV-HLH). Leuk Lymphoma. 2000; 39:37–49. PMID: 10975382.


35. Schuster F, Stachel DK, Schmid I, et al. Griscelli syndrome: report of the first peripheral blood stem cell transplant and the role of mutations in the RAB27A gene as an indication for BMT. Bone Marrow Transplant. 2001; 28:409–412. PMID: 11571516.


36. Amayiri N, Al-Zaben A, Ghatasheh L, Frangoul H, Hussein AA. Hematopoietic stem cell transplantation for children with primary immunodeficiency diseases: single center experience in Jordan. Pediatr Transplant. 2013; 17:394–402. PMID: 23692601.


37. Yoon HS, Im HJ, Moon HN, et al. The outcome of hematopoietic stem cell transplantation in Korean children with hemophagocytic lymphohistiocytosis. Pediatr Transplant. 2010; 14:735–740. PMID: 20113424.


38. Caselli D, Aricò M. The role of BMT in childhood histiocytoses. Bone Marrow Transplant. 2008; 41(Suppl 2):S8–S13. PMID: 18545250.


39. Mahlaoui N, Ouachée-Chardin M, de Saint Basile G, et al. Immunotherapy of familial hemophagocytic lymphohistiocytosis with antithymocyte globulins: a single-center retrospective report of 38 patients. Pediatrics. 2007; 120:e622–e628. PMID: 17698967.


40. Sparber-Sauer M, Hönig M, Schulz AS, et al. Patients with early relapse of primary hemophagocytic syndromes or with persistent CNS involvement may benefit from immediate hematopoietic stem cell transplantation. Bone Marrow Transplant. 2009; 44:333–338. PMID: 19252534.


41. Marsh RA, Rao K, Satwani P, et al. Allogeneic hematopoietic cell transplantation for XIAP deficiency: an international survey reveals poor outcomes. Blood. 2013; 121:877–883. PMID: 23131490.


42. Satwani P, Cooper N, Rao K, Veys P, Amrolia P. Reduced intensity conditioning and allogeneic stem cell transplantation in childhood malignant and nonmalignant diseases. Bone Marrow Transplant. 2008; 41:173–182. PMID: 18037944.


43. Marsh RA, Vaughn G, Kim MO, et al. Reduced-intensity conditioning significantly improves survival of patients with hemophagocytic lymphohistiocytosis undergoing allogeneic hematopoietic cell transplantation. Blood. 2010; 116:5824–5831. PMID: 20855862.


44. Marsh RA, Kim MO, Liu C, et al. An intermediate alemtuzumab schedule reduces the incidence of mixed chimerism following reduced-intensity conditioning hematopoietic cell transplantation for hemophagocytic lymphohistiocytosis. Biol Blood Marrow Transplant. 2013; 19:1625–1631. PMID: 24035782.


45. Marsh RA, Jordan MB, Filipovich AH. Reduced-intensity conditioning haematopoietic cell transplantation for haemophagocytic lymphohistiocytosis: an important step forward. Br J Haematol. 2011; 154:556–563. PMID: 21707584.


46. Lehmberg K, Albert MH, Beier R, et al. Treosulfan-based conditioning regimen for children and adolescents with hemophagocytic lymphohistiocytosis. Haematologica. 2014; 99:180–184. PMID: 24162790.


47. Ohta H, Miyashita E, Hirata I, et al. Hematopoietic stem cell transplantation with reduced intensity conditioning from a family haploidentical donor in an infant with familial hemophagocytic lymphohistocytosis. Int J Hematol. 2011; 94:285–290. PMID: 21863286.


48. Sawada A, Ohga S, Ishii E, et al. Feasibility of reduced-intensity conditioning followed by unrelated cord blood transplantation for primary hemophagocytic lymphohistiocytosis: a nationwide retrospective analysis in Japan. Int J Hematol. 2013; 98:223–230. PMID: 23843148.


49. Nishi M, Nishimura R, Suzuki N, et al. Reduced-intensity conditioning in unrelated donor cord blood transplantation for familial hemophagocytic lymphohistiocytosis. Am J Hematol. 2012; 87:637–639. PMID: 22488407.


50. Méresse V, Hartmann O, Vassal G, et al. Risk factors for hepatic veno-occlusive disease after high-dose busulfan-containing regimens followed by autologous bone marrow transplantation: a study in 136 children. Bone Marrow Transplant. 1992; 10:135–141. PMID: 1525602.
51. Filipovich A, McClain K, Grom A. Histiocytic disorders: recent insights into pathophysiology and practical guidelines. Biol Blood Marrow Transplant. 2010; 16(1 Suppl):S82–S89. PMID: 19932759.


52. Oshrine BR, Olson TS, Bunin N. Mixed chimerism and graft loss in pediatric recipients of an alemtuzumab-based reduced-intensity conditioning regimen for non-malignant disease. Pediatr Blood Cancer. 2014; 61:1852–1859. PMID: 24939325.


53. Terrell CE, Jordan MB. Mixed hematopoietic or T-cell chimerism above a minimal threshold restores perforin-dependent immune regulation in perforin-deficient mice. Blood. 2013; 122:2618–2621. PMID: 23974195.


54. Shenoy S, Grossman WJ, DiPersio J, et al. A novel reduced-intensity stem cell transplant regimen for nonmalignant disorders. Bone Marrow Transplant. 2005; 35:345–352. PMID: 15592491.


55. Koh KN, Im HJ, Chung NG, et al. Clinical features, genetics, and outcome of pediatric patients with hemophagocytic lymphohistiocytosis in Korea: report of a nationwide survey from Korea Histiocytosis Working Party. Eur J Haematol. 2015; 94:51–59. PMID: 24935083.


56. Jackson J, Titman P, Butler S, et al. Cognitive and psychosocial function post hematopoietic stem cell transplantation in children with hemophagocytic lymphohistiocytosis. J Allergy Clin Immunol. 2013; 132:889–895.e1-3. PMID: 23987797.


57. Abdelkefi A, Ben Jamil W, Torjman L, et al. Hemophagocytic syndrome after hematopoietic stem cell transplantation: a prospective observational study. Int J Hematol. 2009; 89:368–373. PMID: 19252966.


58. Asano T, Kogawa K, Morimoto A, et al. Hemophagocytic lymphohistiocytosis after hematopoietic stem cell transplantation in children: a nationwide survey in Japan. Pediatr Blood Cancer. 2012; 59:110–114. PMID: 22038983.


59. Koyama M, Sawada A, Yasui M, Inoue M, Kawa K. Encouraging results of low-dose etoposide in the treatment of early-onset hemophagocytic syndrome following allogeneic hematopoietic stem cell transplantation. Int J Hematol. 2007; 86:466–467. PMID: 18192120.


60. Stepensky P, Bartram J, Barth TF, et al. Persistent defective membrane trafficking in epithelial cells of patients with familial hemophagocytic lymphohistiocytosis type 5 due to STXBP2/MUNC18-2 mutations. Pediatr Blood Cancer. 2013; 60:1215–1222. PMID: 23382066.
61. Trottestam H, Beutel K, Meeths M, et al. Treatment of the X-linked lymphoproliferative, Griscelli and Chédiak-Higashi syndromes by HLH directed therapy. Pediatr Blood Cancer. 2009; 52:268–272. PMID: 18937330.


62. Lozano ML, Rivera J, Sánchez-Guiu I, Vicente V. Towards the targeted management of Chediak-Higashi syndrome. Orphanet J Rare Dis. 2014; 9:132. PMID: 25129365.


63. Tezcan I, Sanal O, Ersoy F, et al. Successful bone marrow transplantation in a case of Griscelli disease which presented in accelerated phase with neurological involvement. Bone Marrow Transplant. 1999; 24:931–933. PMID: 10516709.


64. Pachlopnik Schmid J, Moshous D, Boddaert N, et al. Hematopoietic stem cell transplantation in Griscelli syndrome type 2: a single-center report on 10 patients. Blood. 2009; 114:211–218. PMID: 19403888.


65. Booth C, Gilmour KC, Veys P, et al. X-linked lymphoproliferative disease due to SAP/SH2D1A deficiency: a multicenter study on the manifestations, management and outcome of the disease. Blood. 2011; 117:53–62. PMID: 20926771.


66. Marsh RA, Bleesing JJ, Chandrakasan S, Jordan MB, Davies SM, Filipovich AH. Reduced-intensity conditioning hematopoietic cell transplantation is an effective treatment for patients with SLAM-associated protein deficiency/X-linked lymphoproliferative disease type 1. Biol Blood Marrow Transplant. 2014; 20:1641–1645. PMID: 24923536.
67. Speckmann C, Lehmberg K, Albert MH, et al. X-linked inhibitor of apoptosis (XIAP) deficiency: the spectrum of presenting manifestations beyond hemophagocytic lymphohistiocytosis. Clin Immunol. 2013; 149:133–141. PMID: 23973892.


68. Yang X, Kanegane H, Nishida N, et al. Clinical and genetic characteristics of XIAP deficiency in Japan. J Clin Immunol. 2012; 32:411–420. PMID: 22228567.


69. Imashuku S, Hyakuna N, Funabiki T, et al. Low natural killer activity and central nervous system disease as a high-risk prognostic indicator in young patients with hemophagocytic lymphohistiocytosis. Cancer. 2002; 94:3023–3031. PMID: 12115393.


70. Shuper A, Attias D, Kornreich L, Zaizov R, Yaniv I. Familial hemophagocytic lymphohistiocytosis: improved neurodevelopmental outcome after bone marrow transplantation. J Pediatr. 1998; 133:126–128. PMID: 9672524.


71. Decaminada N, Cappellini M, Mortilla M, et al. Familial hemophagocytic lymphohistiocytosis: clinical and neuroradiological findings and review of the literature. Childs Nerv Syst. 2010; 26:121–127. PMID: 19649640.


72. Carmo M, Risma KA, Arumugam P, et al. Perforin gene transfer into hematopoietic stem cells improves immune dysregulation in murine models of perforin deficiency. Mol Ther. 2015; 23:737–745. PMID: 25523759.


73. Booth C, Carmo M, Gaspar HB. Gene therapy for haemophagocytic lymphohistiocytosis. Curr Gene Ther. 2014; 14:437–446. PMID: 25245087.


Fig. 1
Proposed molecular mechanisms of cytotoxic granule exocytosis illustrated with genetic defects that cause primary HLH. CTL activation by class I MHC-associated antigen of the target cell leads to MTOC polarization and transport of cytotoxic granules. Cytotoxic proteins including granzyme, perforin, and FasL are sorted from the TGN by the M6PR. AP3B1 and LYST are involved in sorting and transport of cytotoxic granules. Using the motor protein dynein-dynactin complex, the granules move along the microtubules toward the MTOC that is polarizing toward the CTL-target cell contact site. The granules then switch from microtubules to the actin filament using another motor protein myosin IIA navigating toward the plasma membrane at the immune synapse, where docking takes place through the interaction of Rab27a and Munc13-4, as well as through the recognition of STX11 and Munc18-2 (STXBP2). After docking, the granules are primed by Munc13-4, which probably triggers the conformational change of STX11 from a closed to an open form by removal of STXBP2. A SNARE complex then forms between a v-SNARE (VAMP) on one side and the t-SNAREs (STX11 and SNAP23) on the other side. Formation of this four-helix SNARE complex bundles leads to membrane fusion and release of the granule contents into the immune synapse. Granzymes enter into the target cell through the pores formed by perforins and provoke apoptosis through activation of caspases. FasL binds to Fas on the target cell surface and initiate apoptosis as well. Various types of primary HLH caused by genetic defects in granule-mediated cytotoxicity are highlighted.
Abbreviations: HLH, hemophagocytic lymphohistiocytosis; CTL, cytotoxic T lymphocyte; MTOC, microtubule organizing center; M6PR, mannose-6-phosphate receptor; AP3B1, AP-3 complex subunit beta-1; LYST, lysosomal trafficking regulator; FasL, Fas ligand; TGN, trans-Golgi network; STX11, syntaxin 11; STXBP2, syntaxin binding protein 2; SNARE, soluble N-ethylmaleimide-sensitive factor attachment protein receptor; v-SNARE, vesicle membrane SNARE; VAMP, vesicle-associated membrane protein; t-SNARE, target membrane SNARE; SNAP23, synaptosomal-associated protein of 23 kDa; CHS, Chédiak-Higashi syndrome; HPS2, Hermansky-Pudlak syndrome type 2; GS2, Griscelli syndrome type 2; FHL, familial HLH.
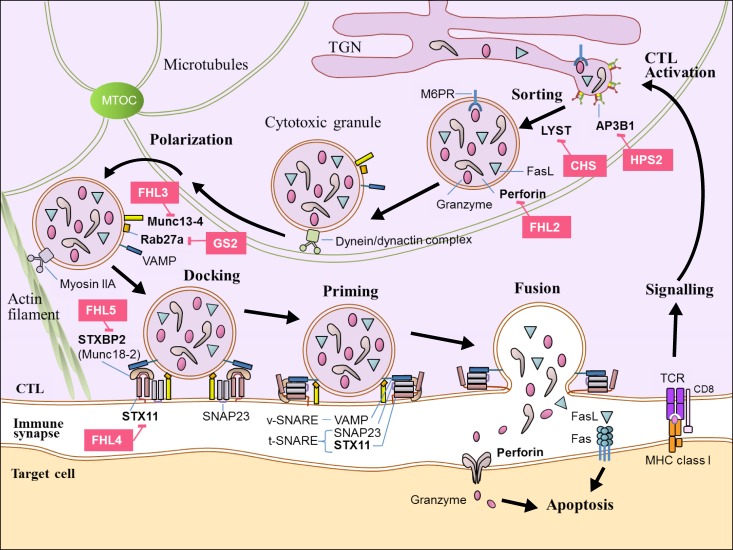