I. Introduction
Table 1
Study design of four experiments for bone regeneration evaluation using BMP and BMP carriers
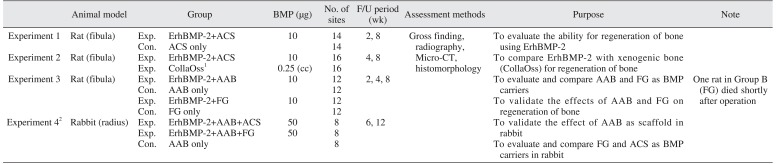
(BMP: bone morphogenetic protein, Exp.: experimental group, Con.: control group, ErhBMP-2: Escherichia coli-derived recombinant human BMP-2, ACS: absorbable collagen sponge, AAB: autoclaved autogenous bone, FG: fibrin glue, F/U: follow-up, Micro-CT: microtomography)
1CollaOss: porcine bone and collagen (Bioland, Korea).
2Experiment 4 was already published in the article of Choi et al. (Maxillofac Plast Reconstr Surg 2014;36:94-102)10.
Experiment 1: the effect of ErhBMP-2/ACS on bone regeneration in a critical sized segmental defect in the rat fibula, Experiment 2: the effect of collagen-combined xenogenic bone (CollaOss) and ErhBMP-2/ACS on bone regeneraton in a critical-size segmental defect in the rat fibula, Experiment 3: comparison of AAB and FG as BMP carriers for bone regeneration in a critical sized segmental defect in the rat fibula, Experiment 4: the bone regerative properties of AAB as a scaffold and comparison of ErhBMP-2/AAB/ACS and ErhBMP-2/AAB/FG for bone regeneration in a critical sized segmental defect in the rabbit radius.

II. Materials and Methods
1. Animals
2. Agents and biomaterials
3. Surgical procedure and experimental design
![]() | Fig. 2Experimental sites of rat fibulae in Experiments 1–3. A. Absorbable collagen sponge with/without recombinant human bone morphogenetic protein-2 (rhBMP-2) in Experiments 1 and 2. B. Xenogenic bone (CollaOss; Bioland, Korea) in Experiment 2. C. Autoclaved autogenous bone (arrow, inset) with/without rhBMP-2 in Group A of Experiment 3. D. Fibrin glue block (arrow, inset) with/without rhBMP-2 in Group B of Experiment 3. |
4. Histologic procedures and evaluation methods

III. Results
1. Gross and radiographic findings
![]() | Fig. 3Gross findings in Experiment 3 (A–D: Group A, E–H: Group B). A, C, D. The 2-, 4-, 8-week experimental groups with rhBMP-2/AAB. Complete bony unions were observed in all groups. The 2-week group showed an ectopic irregular and very excessive generated bone, but the amount of newly formed bone decreased over time through remodeling. E, G, H. The 2-, 4-, 8-week experimental groups with rhBMP-2/FG. Complete bony unions were observed in all groups as Group A. However, the new bone of the 2-week group was formed within relatively limited area comparing with Group A. In the 4-week group, similar or more newly formed bone was observed than in the 2-week group, and ectopic bony growth was also seen. In the 8-week group, a complete union having significantly diminishing the amount of regenerated bone than in the 4-week group was seen. B, F. The 2-week control groups with AAB or FG only. B. Non-union was observed between AAB and the residual fibula ends. F. Segmental bony defect remained on the fibula. (rhBMP-2: recombinant human bone morphogenetic protein-2, AAB: autoclaved autogenous bone, FG: fibrin glue) |
![]() | Fig. 4Microtomography (micro-CT) findings in the experimental groups of Group A in Experiment 3. Comparison of the bone density between the tibia and the newly formed fibula using Micro-CT images. A. The 2-week group; A high-density bi-cortical bone and a low-density regular bone marrow was observed on the adjacent tibia, in contrast with the newly formed fibula bone, which showed an irregular pattern. A high-density bi-cortical bone was also observed on grafted autoclaved autogenous bone (AAB) area. B. The 4-week group; The newly formed fibula bone showed a similar bone density pattern as the adjacent tibia with lower intensity of its cortical portion. Both also showed a low-dense and regular bone marrow. C. The 8-week group; Compared with the 4-week group, the distribution of the bone density was similar to normal bone. The formation of a regular and low-dense cancellous bone was noticeable between the two peaks traced by the cortex of the newly formed bone. |
![]() | Fig. 5Microtomography (micro-CT) findings in the experimental groups of Group B in Experiment 3. Comparison of the bone density between the tibia and the newly formed fibula using Micro-CT images. A. The 2-week group; In the adjacent tibia, a high dense cortical bone was seen as well as a regular and less dense inner cancellous bone. In the fibula site, an over-regenerated bone with an irregular bone density was observed. B. The 4-week group; Same findings as the 2-week experimental group with no remarkable change in bone density. C. The 8-week group; The density pattern of the newly formed fibula, composed of the cortical bone and bone marrow, was similar to that of the adjacent tibia. |
2. Histomorphological findings
![]() | Fig. 7Histomorphologic findings in Group A of Experiment 3 (H&E staining, A–D: ×10, inset: ×100). A. The 2-week experimental group; The formation of immature woven bone and simultaneous bone remodeling, surrounding the transplanted autoclaved autogenous bone (AAB) were observed (arrowhead). B. The 4-week experimental group; A continuous connecting cortical bone was noticeable between remaining fibula bone and newly formed bone and the inner part was gradually replaced by bone marrow. C. The 8-week experimental group; A complete union between the regenerated bone and the pre-existing fibula was observed with a continuous and homogeneous connection of the bone marrow and cortical bone. D. The 8-week control group; A complete union between the newly formed bone and the remaining fibula was observed. The new bone formation and ossification progress were seen surrounding the grafted AAB (arrowhead). |
![]() | Fig. 8Histomorphologic findings in Group B of Experiment 3 (H&E staining, A–D: ×10, inset: ×100). A. The 2-week experimental group; The newly formed bone was observed including endochondral ossification in the both borders near the existing fibula. B. The 4-week experimental group; The cortical layer of the newly formed bone showed a similar continuity as the remaining fibula. The inner part was gradually replaced by bone marrow. C, D. The 8-week experimental and control groups; Both experimental and control groups showed complete unions of the fibula defects. |

IV. Discussion
1. The effect of BMP-2 for bone regeneration (Table 2)
1) Bone regeneration capacity of BMP-2
2) Comparison with xenogenic bone graft material
Table 2
Relative comparison of the amount of bone regeneration on segmental defects of fibulae in rats; Experiments 1, 2, and 3
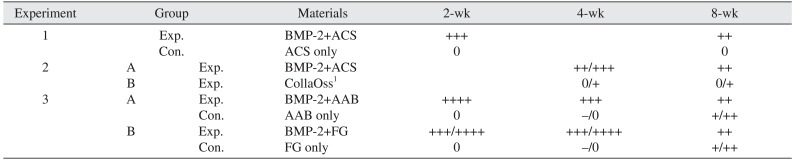
(Exp.: experimental group, Con.: control group, BMP: bone morphogenetic protein, ACS: absorbable collagen sponge, AAB: autoclaved autogenous bone, FG: fibrin glue)
1CollaOss: porcine bone and collagen (Bioland, Korea).
0: no change of size of segmental bony defects, −: widening of segmental bonys defects by resorption of cut ends of fibulae, +: new bone formation without complete unions of segmental bony defects, ++: complete unions of segmental bony defects with newly formed bone, similar to original shape of fibulae, +++: complete unions of segmental bony defects with overgrowing newly formed bone (mild), ++++: complete unions of segmental bony defects with overgrowing newly formed bone (more than mild).
Experiment 1: the effect of ErhBMP-2/ACS on bone regeneration in a critical sized segmental defect in the rat fibula.
Experiment 2: the effect of collagen-combined xenogenic bone (CollaOss) and ErhBMP-2/ACS on bone regeneraton in a critical-size segmental defect in the rat fibula; Group A: BMP-2/ACS group, Group B: CollaOss group.
Experiment 3: comparison of AAB and FG as BMP carriers for bone regeneration in a critical sized segmental defect in the rat fibula; Group A: AAB group (with/without BMP-2), Group B: FG group (with/without BMP-2).
2. Evaluation of biomaterials for a BMP carrier (Table 2)
1) ACS
2) FG
3) AAB

V. Conclusion
