Abstract
Objectives
This study compared the impact of implant surface treatment on the stability and osseointegration of implants in dog mandibles.
Materials and Methods
Six adult dogs received a total of 48 implants that were prepared using four different surface treatments; resorbable blast media (RBM), hydroxyapatite (HA), hydrothermal-treated HA, and sand blasting and acid etching (SLA). Implants were installed, and dogs were separated into 2- and 4-week groups. Implant stability was evaluated via Periotest M, Osstell Mentor, and removal torque analyzers. A histomorphometric analysis was also performed.
Results
The stability evaluation showed that all groups generally had satisfactory values. The histomorphometric evaluation via a light microscope revealed that the HA surface implant group had the highest ratio of new bone formation on the entire fixture. The hydrothermal-treated HA surface implant group showed a high ratio of bone-to-implant contact in the upper half of the implant area.
Edentulous area restoration with an implant is a common therapeutic approach123. Several requirements are necessary to achieve early stage and long-term implant insertion success. The requirements include having good osseointegration between the implant and the alveolar bone2. Albrektsson et al.4 suggested six important elements that affect osseointegration of implants: the material, design and surface of the implant, as well as the bone condition at the implantation site, the surgical procedure, and the loading conditions45. For the past 20 years, studies have been conducted to optimize implant surfaces, and aspects including chemical treatment and physical factors, such as the coarseness of the surface, have been investigated6.
The traditional Brånemark titanium implant is polished mechanically to obtain a smooth surface. However, since it has been reported that implants with a coarse surface could be more useful in the spongy bone with the poor bone quality, many authors have investigated applications of coarse surfaces67. Titanium plasma spray (TPS) is a method that is used to generate a course surface and to increase the physical bone contact area8. Recently, a surface treatment that uses plasma spray, which involves aluminum oxide (Al2O3), titanium dioxide (TiO2), and calcium phosphate (CaP), such as hydroxyapatite (HA) with high biocompatibility, has received more attention9. In addition, acid etching involving sulfuric acid (H2SO4), hydrogen chloride (HCl), or nitric acid (HNO3) can make the surface coarser and facilitate osteocyte generation. Some studies have employed sand blasting and acid etching (SLA)910. Additionally, fluorination or anodic oxidation to increase osseointegration has also been investigated for implant insertion11.
We performed histomorphometric comparison of implant osseointegration when the implant was treated with four different surface treatments; resorbable blast media (RBM), which has recently been commercialized, HA coating, acid etching followed by plasma spray and hydrothermal-treated HA, to improve the degree of particle crystallinity.
The experiments were carried out using six healthy adult dogs aged 18 to 24 months and the study was approved by the Institutional Animal Care and Use Committee, Chosun University (IRB No. CIACUC2013-A0019). Each dog was injected intramuscularly in the thigh with muscle relaxant (2% xylazine hydrochloride, 10 mg/kg, Rompun Inj.; Bayer, Seoul, Korea) and anesthetic (tiletamine, zolazepam, 10 mg/kg, Zoletil 50; Virbac Lab, Carros, France), and 2% hydrochloride lidocaine (Yuhan Co., Ltd., Seoul, Korea) containing 1:100,000 epinephrine was used. The mandibular first and second premolars and molars were extracted on both sides. Wounds were sutured using 4-0 Vicryl (Ethicon, Somerville NJ, USA).
Two months after extracting the teeth, the right mandible of each animal was treated with local anesthetic followed by anesthesia induction. The four types of implants were inserted according to the surface treatment; implants treated with RBM (Clearant; Dentis Inc., Daegu, Korea), HA implants coated with ultra-thin HA film at room temperature (Haptite; Dentis Inc.), hydrothermal-treated HA implants treated with hot water and coated with HA (Dentis Inc.), and SLA implants treated with plasma spray followed by acid etching (One Q SL; Dentis Inc.). A total of 48 implants had identical lengths and diameters (3.7×8.0 mm) and were classified into 2- and 4-week groups. Two implants from each group (a total of eight implants) were inserted in each dog. Furthermore, each implant was inserted up to 1 mm in depth on the superior border of the alveolar bone, with more than 35-N insertion torque. After the insertions, the incised mucosa was relocated and sutured with 4-0 Vicryl.
The animals were sacrificed using suxamethonium chloride hydrate (0.11 mg/kg, Eagle Suxamethonium Inj.; Eaglevet Co., Seoul, Korea). Directly after sacrifice, the mandibles were collected, preserved in formalin for 10 days, and embedded with glycol-metacrylate resin (Spurr low-viscosity embedding media; Polysciences, Warrington, PA, USA). The specimens were cut using a high-precision diamond disc and polished to 50 µm or less in thickness, using a lapping and polishing machine (OMNILAP 2000; SBT, San Clemente, CA, USA). Finally, the histological specimens were prepared using Villanueva osteochrome bone stain (SBT).
To test the primary fixation and stability of the implants, Periotest M (Medizintechnik Gulden Co., Modautal, Germany) was used to estimate the periotest value (PTV) as a representative value on the buccal side of each implant directly after placement and sacrifice. Osstell Mentor (Integration Diagnostics AB, Göteberg, Sweden) was used to simultaneously estimate the implant stability quotient (ISQ) via resonance frequency analysis (RFA) on the medial, distal, buccal and lingual sides of the implant. The MGT 50 removal torque analyzer (Checkline Europe Co., Enschede, the Netherlands) was used to estimate the rotation torque in one of the sacrificed dogs.
An optical microscope (Olympus BX50; Olympus, Tokyo, Japan) was used for the histomorphometric assessment. The ratio of the new bone formation area (NBFA) to the entire implant was determined, and the bone-implant contact (BIC) on the upper half was examined, which was determined to be more significant for implant stability. PASW Statistics version 18 (IBM Co., Armonk, NY, USA) was used to analyze each of the estimates. Statistical significance was determined by one-way ANOVA, and statistical analysis was carried out at the 95% confidence level.
The ISQ was at a favorable level (70 or more) in both the 2- and 4-week groups. The implants treated with SLA showed the highest stability directly after the insertion. Those treated with RBM showed the greatest stability in the 2-week group, although this result was not statistically significant. In the 4-week group, the HA implants showed the most stability, and there were significant differences from hydrothermal-treated HA implants.(Table 1)
The PTV ranged from –4.33 to –6.07 at the time of ISQ estimation. On average, smaller PTV and greater implant stability were observed in the 4-week group when compared to the 2-week group. Directly after insertion, significant differences were observed between the implants coated with HA and those treated with SLA; on average, the latter showed the highest stability in both the 2- and 4-week groups.(Table 2)
The HA-coated implants showed slightly higher removal torque values (100.7 N/cm) in the 2-week group, while all of the implants in the 4-week group displayed the maximum removal torque value (135 N/cm) or higher. The analysis did not identify any statistically significant differences in each group.(Table 3)
The degree of osseointegration between the implant and the alveolar bone plays an essential role in successful implant insertion1213. Research has focused on the surface treatment of implants to improve osseointegration in implant insertions, and morphological studies have examined the thread design in direct contact with the bone2. Ratner and Porter14 contended that the morphological and chemical properties of the implant, including surface charge, wettability, protein absorption, cell-surface interaction and cell and tissue growth on the surface, are important elements that affect the interaction between the surface and the bioactive materials. Davies15 observed that successful osseointegration involves activation of platelets at the implant surface, which causes a knock-on effect between the implant surface and osteocytes, resulting in new bone formation and increased maintenance of bone conduction.
Some studies have indicated that implants with a coarse surface might be preferable compared with mechanically polished smooth titanium implants16. Shalabi et al.6 observed that BIC was correlated with implant surface coarseness. In addition, Thompson et al.7 found that the removal torque increased with the increase in surface coarseness.
In this study, we determined the degree of osseointegration using the Periotest M and Osstell Mentor to estimate implant stability, and we used the MGT 50 gauge to estimate the removal torque. The PTV can be used clinically to estimate the histological degree of osseointegration, and the movement of the metal rod is a convenient and useful way to estimate implant stability17. However, since the measurement is likely to vary according to the site and angle of the abutment, all implant measurements were performed at the same site, to minimize the likelihood of variability. The Osstell Mentor is frequently used to estimate the ISQ through RFA. However, this measurement is affected by the length of the implant above the bone level, and for this reason, we used equal insertion depths for all of the implants. The medial, distal, buccal and lingual sides of each implant were averaged to reduce the disparity among measurement directions13. Histomorphometric analyses, using an optical microscope, were performed to yield immediate and objective findings.
This study involved implants that were treated with recently commercialized RBM, as well as HA or SLA. RBM is used to spray resorbable blast material, such as hydrated titanium and calcium phosphate, to make the surface of the implant coarse18; Piattelli et al.19 reported that RBM-treated implants showed the presence of bone matrix, which was not observed using implants with mechanically polished surfaces. Moreover, RBM-treated implants resulted in greater BIC and bone conduction. Granato et al.20 found no statistical significance in the histomorphometric analysis in adult dogs that received acid-etched and RBM-treated implants. This study found that RBM-treated implants resulted in poor osseointegration with relatively smaller BIC or removal torque on average than HA-coated implants, although this trend was not statistically significant.
Yang et al.21 observed that implants with SLA-treated surfaces showed greater removal torque and BIC than those with mechanically polished surfaces in the 2-, 4- and 8-week groups. Cochran et al.22 used animal testing to determine that the SLA-treated implants had significantly greater BIC than those treated with TPS, probably because marginal bone resorption after implant loading was minimized by SLA, which maximized the secondary bone regeneration.
HA-coated implants have been investigated since 1984, and optical microscopy has shown that the interaction between HA-coated implants and bone is characterized as a type of integration that results from the biochemical combination of the bone and the surface of the implant23. Retrospective clinical research showed fewer failures with HA-coated implants than those treated with TPS24. However, although HA-coated implants show better initial osseointegration after insertion, the HA-implant interaction is more likely to be damaged by prosthetic load, which may lead to unsuccessful implantation over time2425. This damage occurs because the existing plasma spray can make the film permeable, thereby causing residual stress on the surface and changing the initial constitution and degree of crystallinity of the calcium phosphate particles26. To circumvent this potential damage, Yang et al.27 presented an electrochemically effective implant coating that was generated by combining HA coating and nanotechnology, and this coating was associated with greater BIC outcomes than previous implants.
Some authors reported that hot water treatment could maximize anodic oxidation and improve bioactivity9. Ishizawa and Ogino28 reported that anodized titanium coating, combined with 300℃ water treatment, yielded a 1- to 2-µm layer of HA, which could decrease particle cohesion and increase the maximum bond stress up to 40 MPa. In this study, hot water treatment, in conjunction with new technology to improve crystallinity of the particles, also led to better BIC than the HA, RBM and SLA coatings on average, although the differences were not statistically significant.
Ionization of the implant surface can increase surface stress, which can then increase protein absorption and affect the contact angle. In addition, ionization effectively facilitates osteoblast accumulation and differentiation2930; De Maeztu et al.31 found that mechanical polishing combined with CO ionization could generate better BIC than other types of implant surface treatments, such as mechanical polishing without ionization, acid etching, and SLA. In addition, biomolecule coatings using collagen I and osteocalcin have been found to generate successful osseointegration1.
In this study, we examined implant osseointegration using four types of implant surface treatment; RBM, HA coating, HA coating combined with hot water treatment, and SLA-treated implants in the jawbone of adult dogs. In general, all types of implant treatments led to good implant stability. ISQ results for the 4-week group showed that the implants coated with ultra-thin HA film at room temperature were most stable. Histomorphometric analysis revealed that the implants coated with ultra-thin HA film at room temperature showed the highest ratio of NBFA and that the implants treated with hot water and coated with HA showed the greatest BIC on the upper half of the implant.
In general, all types of implant surface treatments led to good osseointegration. Hydrothermal-treated HA showed excellent osseointegration in the upper half of the implant. To improve osseointegration, further research is required to combine the existing implant surface treatment methods with modified and improved technology.
References
1. Mueller CK, Thorwarth M, Schmidt M, Schlegel KA, Schultze-Mosgau S. Comparative analysis of osseointegration of titanium implants with acid-etched surfaces and different biomolecular coatings. Oral Surg Oral Med Oral Pathol Oral Radiol Endod. 2011; 112:726–736. PMID: 21441047.


2. Abuhussein H, Pagni G, Rebaudi A, Wang HL. The effect of thread pattern upon implant osseointegration. Clin Oral Implants Res. 2010; 21:129–136. PMID: 19709058.


3. de Vicente JC, Recio O, Martín-Villa L, Junquera LM, López-Arranz JS. Histomorphometric evaluation of guided bone regeneration around implants with SLA surface: an experimental study in beagle dogs. Int J Oral Maxillofac Surg. 2006; 35:1047–1053. PMID: 16973332.


4. Albrektsson T, Brånemark PI, Hansson HA, Lindström J. Osseointegrated titanium implants. Requirements for ensuring a long-lasting, direct bone-to-implant anchorage in man. Acta Orthop Scand. 1981; 52:155–170. PMID: 7246093.
5. Brånemark PI, Adell R, Breine U, Hansson BO, Lindström J, Ohlsson A. Intra-osseous anchorage of dental prostheses. I. Experimental studies. Scand J Plast Reconstr Surg. 1969; 3:81–100. PMID: 4924041.
6. Shalabi MM, Gortemaker A, Van't Hof MA, Jansen JA, Creugers NH. Implant surface roughness and bone healing: a systematic review. J Dent Res. 2006; 85:496–500. PMID: 16723643.


7. Thompson JI, Gregson PJ, Revell PA. Analysis of push-out test data based on interfacial fracture energy. J Mater Sci Mater Med. 1999; 10:863–868. PMID: 15347966.
8. Buser D, Schenk RK, Steinemann S, Fiorellini JP, Fox CH, Stich H. Influence of surface characteristics on bone integration of titanium implants. A histomorphometric study in miniature pigs. J Biomed Mater Res. 1991; 25:889–902. PMID: 1918105.


9. Le Guéhennec L, Soueidan A, Layrolle P, Amouriq Y. Surface treatments of titanium dental implants for rapid osseointegration. Dent Mater. 2007; 23:844–854. PMID: 16904738.


10. Buser D, Broggini N, Wieland M, Schenk RK, Denzer AJ, Cochran DL, et al. Enhanced bone apposition to a chemically modified SLA titanium surface. J Dent Res. 2004; 83:529–533. PMID: 15218041.


11. Ellingsen JE, Johansson CB, Wennerberg A, Holmén A. Improved retention and bone-tolmplant contact with fluoride-modified titanium implants. Int J Oral Maxillofac Implants. 2004; 19:659–666. PMID: 15508981.
12. Jeong R, Marin C, Granato R, Suzuki M, Gil JN, Granjeiro JM, et al. Early bone healing around implant surfaces treated with variations in the resorbable blasting media method. A study in rabbits. Med Oral Patol Oral Cir Bucal. 2010; 15:e119–e125. PMID: 19767688.


13. Meredith N, Book K, Friberg B, Jemt T, Sennerby L. Resonance frequency measurements of implant stability in vivo. A cross-sectional and longitudinal study of resonance frequency measurements on implants in the edentulous and partially dentate maxilla. Clin Oral Implants Res. 1997; 8:226–233. PMID: 9586467.
14. Ratner BD, Porter SC. Surfaces in biology and biomaterials: description and characterization. In : Brash JL, Wojciechowski PW, editors. Interfacial phenomena and bioproducts. New York: Marcel Dekker;1996. p. 57–83.
15. Davies JE. Understanding peri-implant endosseous healing. J Dent Educ. 2003; 67:932–949. PMID: 12959168.


16. Schwarz ML, Kowarsch M, Rose S, Becker K, Lenz T, Jani L. Effect of surface roughness, porosity, and a resorbable calcium phosphate coating on osseointegration of titanium in a minipig model. J Biomed Mater Res A. 2009; 89:667–678. PMID: 18442101.


17. Olivé J, Aparicio C. Periotest method as a measure of osseointegrated oral implant stability. Int J Oral Maxillofac Implants. 1990; 5:390–400. PMID: 2094658.
18. Coelho PG, Granjeiro JM, Romanos GE, Suzuki M, Silva NR, Cardaropoli G, et al. Basic research methods and current trends of dental implant surfaces. J Biomed Mater Res B Appl Biomater. 2009; 88:579–596. PMID: 18973274.


19. Piattelli M, Scarano A, Paolantonio M, Iezzi G, Petrone G, Piattelli A. Bone response to machined and resorbable blast material titanium implants: an experimental study in rabbits. J Oral Implantol. 2002; 28:2–8. PMID: 12498456.


20. Granato R, Marin C, Suzuki M, Gil JN, Janal MN, Coelho PG. Biomechanical and histomorphometric evaluation of a thin ion beam bioceramic deposition on plateau root form implants: an experimental study in dogs. J Biomed Mater Res B Appl Biomater. 2009; 90:396–403. PMID: 19107801.


21. Yang GL, He FM, Yang XF, Wang XX, Zhao SF. Bone responses to titanium implants surface-roughened by sandblasted and double etched treatments in a rabbit model. Oral Surg Oral Med Oral Pathol Oral Radiol Endod. 2008; 106:516–524. PMID: 18602288.


22. Cochran DL, Schenk RK, Lussi A, Higginbottom FL, Buser D. Bone response to unloaded and loaded titanium implants with a sandblasted and acid-etched surface: a histometric study in the canine mandible. J Biomed Mater Res. 1998; 40:1–11. PMID: 9511093.


23. Block MS, Kent JN, Kay JF. Evaluation of hydroxylapatite-coated titanium dental implants in dogs. J Oral Maxillofac Surg. 1987; 45:601–607. PMID: 3037051.


24. Jones JD, Lupori J, Van Sickels JE, Gardner W. A 5-year comparison of hydroxyapatite-coated titanium plasma-sprayed and titanium plasma-sprayed cylinder dental implants. Oral Surg Oral Med Oral Pathol Oral Radiol Endod. 1999; 87:649–652. PMID: 10397651.


25. Cook SD, Baffes GC, Palafox AJ, Wolfe MW, Burgess A. Torsional stability of HA-coated and grit-blasted titanium dental implants. J Oral Implantol. 1992; 18:354–365. PMID: 1298818.
26. Radin SR, Ducheyne P. Plasma spraying induced changes of calcium phosphate ceramic characteristics and the effect on in vitro stability. J Mater Sci Mater Med. 1992; 3:33–42.
27. Yang GL, He FM, Hu JA, Wang XX, Zhao SF. Effects of biomimetically and electrochemically deposited nano-hydroxyapatite coatings on osseointegration of porous titanium implants. Oral Surg Oral Med Oral Pathol Oral Radiol Endod. 2009; 107:782–789. PMID: 19201624.


28. Ishizawa H, Ogino M. Characterization of thin hydroxyapatite layers formed on anodic titanium oxide films containing Ca and P by hydrothermal treatment. J Biomed Mater Res. 1995; 29:1071–1079. PMID: 8567705.


29. Braceras I, Alava JI, Goikoetxea L, de Maeztu MA, Onate JI. Interaction of engineered surfaces with the living world: ion implantation vs. osseointegration. Surf Coat Technol. 2007; 201:8091–8098.


30. Yang B, Uchida M, Kim HM, Zhang X, Kokubo T. Preparation of bioactive titanium metal via anodic oxidation treatment. Biomaterials. 2004; 25:1003–1010. PMID: 14615165.


31. De Maeztu MA, Braceras I, Alava JI, Gay-Escoda C. Improvement of osseointegration of titanium dental implant surfaces modified with CO ions: a comparative histomorphometric study in beagle dogs. Int J Oral Maxillofac Surg. 2008; 37:441–447. PMID: 18339518.


Fig. 1
Resorbable blast media surface fixture light micrographs in the 2-week (A, B) and 4-week (C, D) groups. The new bone formation area and bone-implant contact increased in the 4-week group (Villanueva osteochrome bone stain, A, C: ×12.5, B, D: ×40).
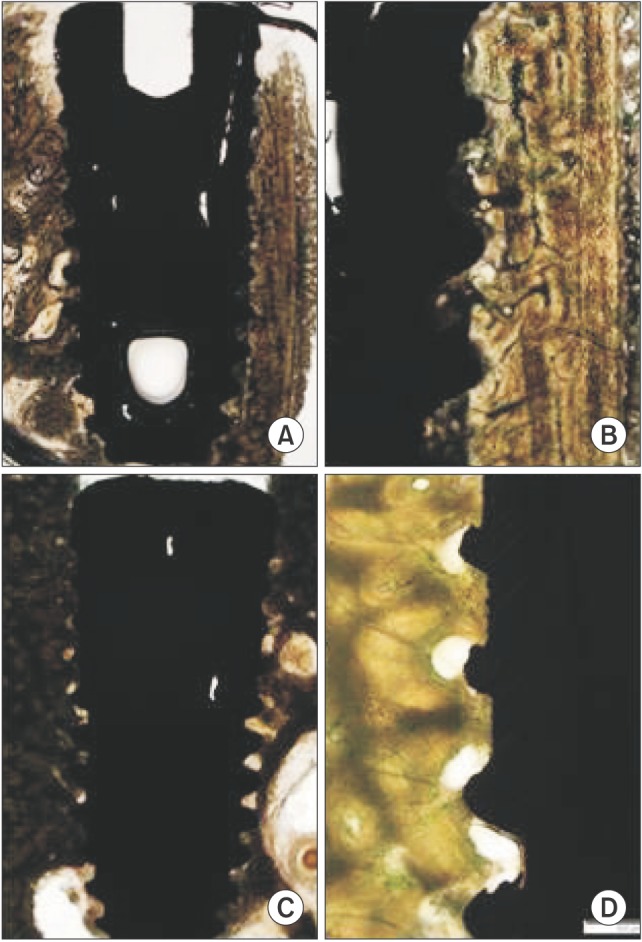
Fig. 2
Hydroxyapatite (HA) surface fixture light micrographs in the 2-week (A, B) and 4-week (C, D) groups. The new bone formation area (NBFA) and bone-implant contact increased in the 4-week group. Compact new bone formation was identified around the implant. The best NBFA value was obtained in this HA group (Villanueva osteochrome bone stain, A, C: ×12.5, B, D: ×40).
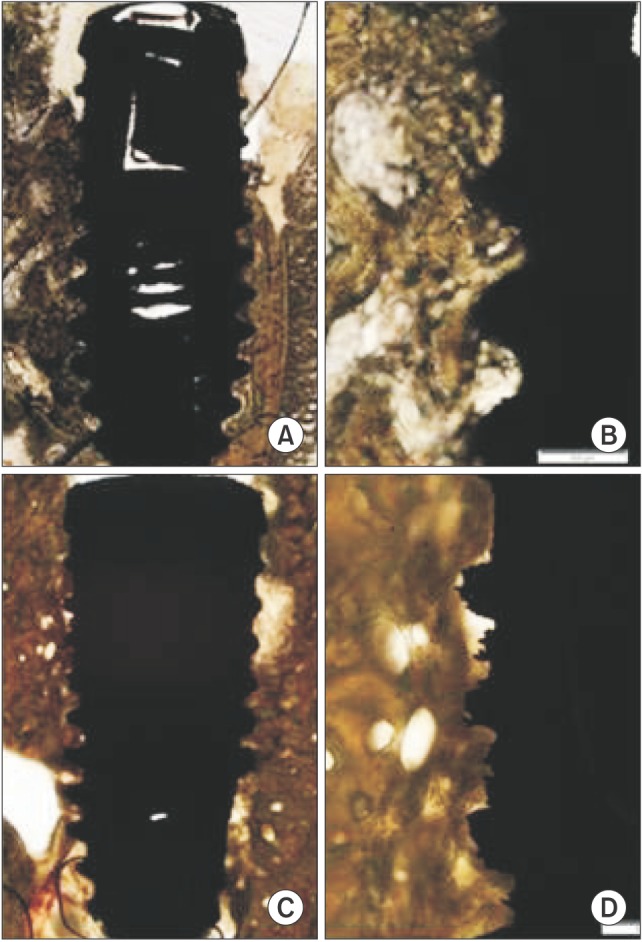
Fig. 3
Hydrothermal-treated hydroxyapatite (HA) surface fixture light micrographs in the 2-week (A, B) and 4-week (C, D) groups. The new bone formation area and bone-implant contact (BIC) increased in the 4-week group. Continuous BIC was identified along the surface of the implant. The best BIC value was obtained in this hydrothermal-treated HA group (Villanueva osteochrome bone stain, A, C: ×12.5, B, D: ×40).
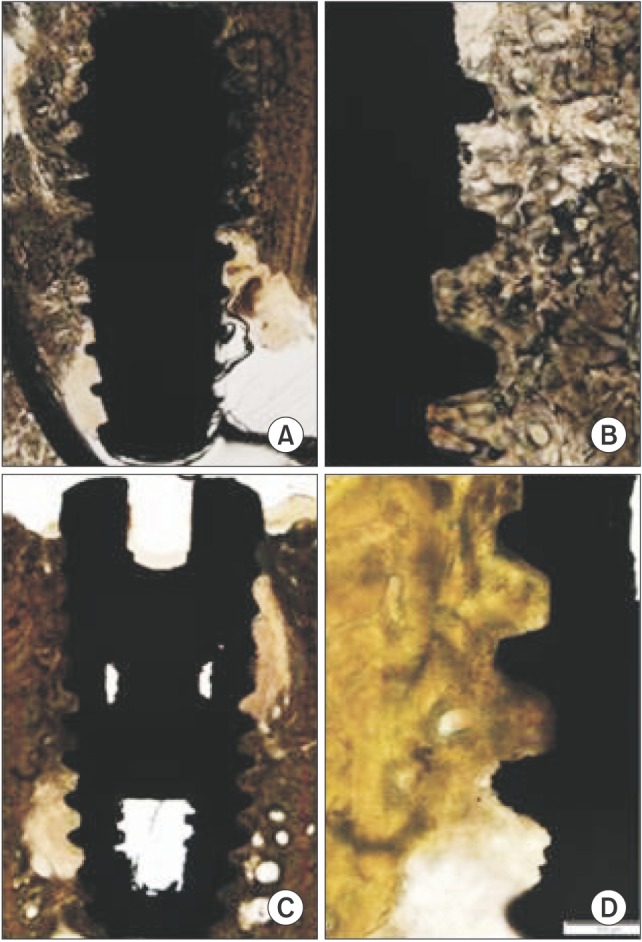
Fig. 4
The sand blasting and acid etching surface fixture light micrographs in the 2-week (A, B) and 4-week (C, D) groups. The new bone formation area and bone-implant contact increased in the 4-week group (Villanueva osteochrome bone stain, A, C: ×12.5, B, D: ×40).
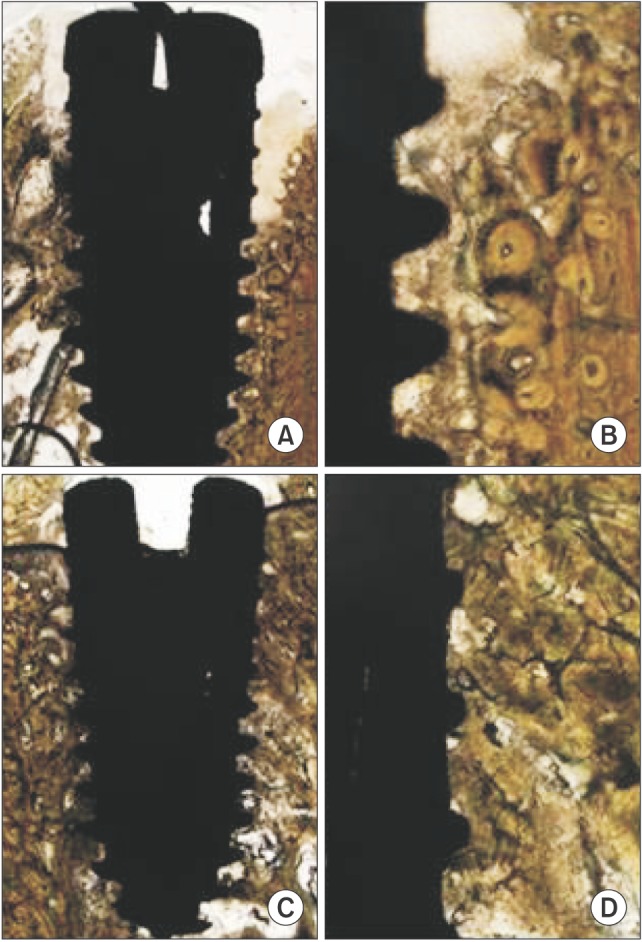
Table 1
Implant stability quotient based on resonance frequency analysis
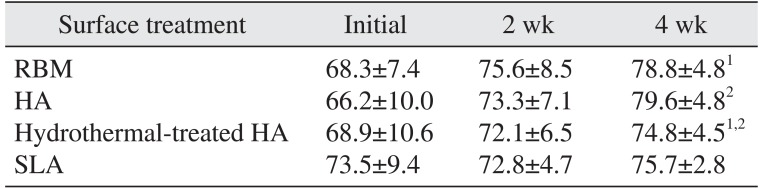
Surface treatment | Initial | 2 wk | 4 wk |
---|---|---|---|
RBM | 68.3±7.4 | 75.6±8.5 | 78.8±4.81 |
HA | 66.2±10.0 | 73.3±7.1 | 79.6±4.82 |
Hydrothermal-treated HA | 68.9±10.6 | 72.1±6.5 | 74.8±4.51,2 |
SLA | 73.5±9.4 | 72.8±4.7 | 75.7±2.8 |
(RBM: resorbable blast media, HA: hydroxyapatite, SLA: sand blasting and acid etching)
1Statistically significant difference RBM and hydrothermal-treated HA groups (P<0.01).
2Statistically significant difference HA and hydrothermal-treated HA group (P<0.01).
Values are presented as mean±standard deviation.
Table 2
Periotest value
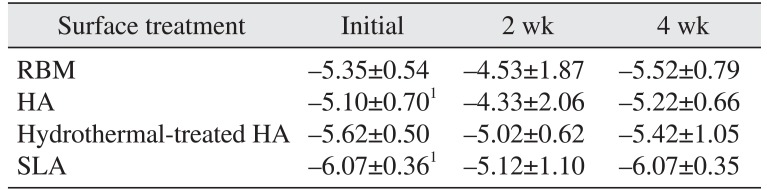
Table 3
Removal torque value (N/cm)
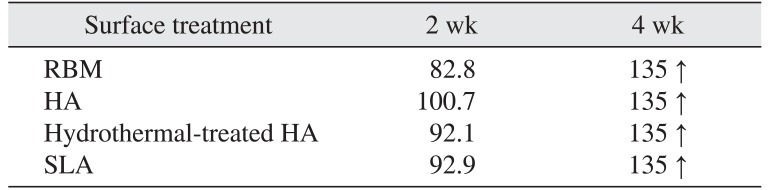
Surface treatment | 2 wk | 4 wk |
---|---|---|
RBM | 82.8 | 135 ↑ |
HA | 100.7 | 135 ↑ |
Hydrothermal-treated HA | 92.1 | 135 ↑ |
SLA | 92.9 | 135 ↑ |
Table 4
New bone formation area ratios (%)
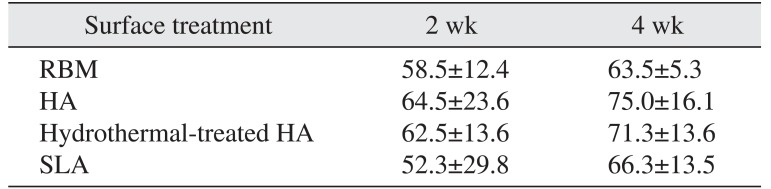
Surface treatment | 2 wk | 4 wk |
---|---|---|
RBM | 58.5±12.4 | 63.5±5.3 |
HA | 64.5±23.6 | 75.0±16.1 |
Hydrothermal-treated HA | 62.5±13.6 | 71.3±13.6 |
SLA | 52.3±29.8 | 66.3±13.5 |