Abstract
Objectives
Bisphosphonate-related osteonecrosis of the jaw (BRONJ) is a side effect of bisphophonate therapy that has been reported in recent years. Osteoclastic inactivity by bisphosphonate is the known cause of BRONJ. Bone morphogenetic protein-2 (BMP-2) plays an important role in the development of bone. Recombinant human BMP-2 (rhBMP-2) is potentially useful as an activation factor for bone repair. We hypothesized that rhBMP-2 would enhance the osteoclast-osteoblast interaction related to bone remodeling.
Materials and Methods
Human fetal osteoblast cells (hFOB 1.19) were treated with 100 µM alendronate, and 100 ng/mL rhBMP-2 was added. Cells were incubated for a further 48 hours, and cell viability was measured using an MTT assay. Expression of the three cytokines from osteoblasts, receptor activator of nuclear factor-κB ligand (RANKL), osteoprotegerin (OPG), and macrophage colony-stimulating factor (M-CSF), were analyzed by real-time polymerase chain reaction and enzyme-linked immunosorbent assay.
Results
Cell viability was decreased to 82.75%±1.00% by alendronate and then increased to 110.43%±1.35% after treatment with rhBMP-2 (P<0.05, respectively). OPG, RANKL, and M-CSF expression were all decreased by alendronate treatment. RANKL and M-CSF expression were increased, but OPG was not significantly affected by rhBMP-2.
Bisphosphonates (BPs) are used to treat osteoporosis, bone metastasis and other conditions involving fragile bone. Oral BPs are used primarily in the treatment of osteoporosis, and alendronate is one of the most potent antiosteoporotic agents known. As a side effect of using BPs, BP-related osteonecrosis of the jaw (BRONJ) was reported in 2003; in the field of oral and maxillofacial surgery, it is one of the most difficult diseases to treat1.
Alendronate pharmacologically inhibits farnesyl diphosphate synthase in the mevalonate pathway, which is essential for the prenylation of proteins in osteoclasts. This ultimately causes mechanical inhibition of osteoclast adhesion on the bone margin where absorption takes place through osteoclast apoptosis. The effect of BPs on osteoclasts could also arise, at least in part, from modulation of the synthesis of resorptionpromoting or resorption-inhibiting factors by osteoblasts2. Osteoblast/stromal cells regulate osteoclastogenesis by producing proteins such as macrophage colony-stimulating factor (M-CSF), receptor activator of nuclear factor-κB ligand (RANKL), and osteoprotegerin (OPG). Through cell-to-cell contact of osteoblast/stromal cells with osteoclasts, RANKL and M-CSF induce osteoclast progenitor cells to differentiate into osteoclasts3.
Bone morphogenetic protein (BMP), a subgroup of the transforming growth factor-β (TGF-β) superfamily4, induces the formation of bone and cartilage5,6,7,8,9,10. To date, there are more than 20 known BMP subgroups, of which BMP-2 is the primary subgroup used as a clinical treatment in many in vitro and in vivo studies11. Recombinant human BMP-2 (rh-BMP-2) is an activation factor for bone healing and has been used in dentistry due to its osteogenic effects.
The aim of the present study was to explore the effect of rhBMP-2 on the regulation of OPG, RANKL, and M-CSF in osteoclastogenesis in human osteoblast treated with alendronate.
The following reagents were commercially obtained: Pro-Long Gold Antifade Reagent with DAPI, from Molecular Probes (Eugene, OR, USA); Dulbecco's modified Eagle's medium F12 (DMEM/F12) and fetal bovine serum (FBS), from Gibco (Gaithersburg, MD, USA); Alendronate, Dimethyl sulfoxide (DMSO), Hoechst 33342, RNase A, proteinase K, aprotinin, leupeptin, Triton X-100, PMSF and thiazolyl blue tetrazolium bromide (MTT), from Sigma (St. Louis, MO, USA); SuperSignal West Femto enhanced-chemiluminescence Western blotting detection reagent, from Pierce (Rockford, IL, USA).
An hFOB 1.19 human fetal osteoblast cell line was purchased from the American Type Culture Collection (Rockville, MD, USA). The cells were maintained at 34℃ with 5% CO2 in DMEM/F12 with 4 mM L-glutamine, 1.5 g/L sodium bicarbonate, 4.5 g/L glucose and 1.0 mM sodium pyruvate supplemented with 10% FBS, under an air atmosphere.
A total of 1×104 cells were seeded in a 96-well plate, incubated for 24 hours, and treated with 0, 100, 500 or 1,000 µM concentrations of alendronate. The cells were then treated with 500 µg/mL of MTT stock solution and incubated at 34℃ in a 5% CO2 atmosphere for 4 hours. The medium was aspirated, and the formazan crystals were dissolved in DMSO. Cell viability was monitored on an enzyme-linked immunosorbent assay (ELISA) reader (Tecan, Männedorf, Switzerland) at a 570 nm excitatory emission wave length. In cells treated with 100 µM alendronate, cell viability was approximately 80% of the control. Consequently, that concentration was selected for the experimental group.
Expression levels of the three proteins, RANKL, OPG and M-CSF, were measured with an ELISA kit (Quantikine; R&D Systems, Minneapolis, MN, USA) according to the manufacturer's instructions. Briefly, cultured hFOB 1.19 cells were uniformly seeded into 6-well culture dishes at a concentration of 2×105 cells/well. When the cells were adherent 24 hours later, the original medium was replaced with medium containing 100 µM alendronate for 48 hours (alendronate group) after which 100 ng/mL BMP-2 (Cowellmedi, Busan, Korea) was added, and the cells were incubated for a further 48 hours (alendronate+rhBMP-2 group). The supernatants were then collected from each group, and an ELISA was used to determine the RANKL, OPG and M-CSF contents of each sample. The sample values were read from the standard curve set at 450 nm with 540-570 nm wavelength correction and with the measurement units expressed as pg/mL. All samples were assayed simultaneously.
The hFOB 1.19 cells were subjected to RNA extraction using spin columns (Rneasy; Qiagen, Hilden, Germany) according to the manufacturer's instructions. RNA (2 µg) was reverse-transcribed, using the RevertAid First-Strand Synthesis System kit for RT-PCR (Thermo Fisher Scientific, Pittsburgh, PA, USA) according to the manufacturer's protocol. The cDNA was amplified with the PCR master mix SYBR Green kit (Applied Biosystems, Warrington, UK), and PCR amplification was performed using the Chromo4 Real-Time PCR Detection System (Bio-Rad Laboratories Inc., Hercules, CA, USA). The running conditions were as follows: denaturing at 95℃ for 3 minutes, 40 cycles of amplification at 95℃ for 15 seconds and 60℃ for 30 seconds. After the final cycle, a melting curve analysis was performed at 55℃-95℃ intervals in 0.5℃ steps. The sense and anti-sense primer sequences of OPG, RANKL, M-CSF, and the housekeeping gene glyceraldehyde 3-phophate dehydrogenase (GAPDH) are listed in Table 1.
An MTT assay was performed at 48 hours with 0, 100, 500, 1,000 µM alendronate concentrations.(Fig. 1) Alendronate inhibited cellular viability at 100 µM (P<0.05). When the cells were exposed to concentrations above 100 µM, there was a significant decrease in cell viability (P<0.05).
To investigate the reverse effects of rhBMP-2 on cell viability, cell viability assays were repeated with application of rhBMP-2 to cells treated with 100 µM alendronate after 48 hours.(Table 2) Cell viability significantly increased relative to the control and alendronate alone group (P<0.05).
To evaluate OPG expression, RT-PCR and an ELISA were performed. OPG expression in the alendronate group was significantly decreased (P=0.012) as compared to the control group. In the alendronate+rhBMP-2 group, the OPG level increased relative to alendronate group, but this increase was not significant (P=0.482).(Fig. 2, Tables 3, 4)
RT-PCR and an ELISA were also performed to evaluate RANKL. In the alendronate group, RANKL expression was significantly decreased (P=0.003) as compared to the control. In the alendronate+rhBMP-2 group, RANKL expression was significantly increased relative to the alendronate alone group (P=0.003).(Fig. 3, Tables 3, 4)
To determine whether alendronate and rhBMP-2 treatment change the expression of M-CSF, RT-PCR and an ELISA were also done. In the alendronate group, M-CSF expression Maxilwas decreased significantly compared with the control group (P=0.000). In the alendronate+rhBMP-2 group, the M-CSF level was decreased significantly compared with the control group but increased significantly compared with the alendronate group (P=0.002).(Fig. 4, Table 3)
We investigated the effects of rhBMP-2 on osteoblasts treated with alendronate, since alendronate is the most widely prescribed oral BP and appears most likely to cause BRONJ12. The effects of BPs on osteoclasts are well understood, and the effects of BPs on osteoclastic toxicity are thought to influence the occurrence of BRONJ. Although the majority of in vitro BPs studies have focused on BPs actions in osteoclastic lineage cells, recent studies have suggested that the presence of osteoblastic lineage cells is required for the anti-resorptive effects of BPs13. An understanding of the effects of alendronate on hFOB cells, particularly in the OPG/RANKL system, however, is lacking. To address this lack of data, the current study investigated the expression of RANKL, OPG and M-CSF in BP-treated hFOB cells.
Here, alendronate was shown to have a strong negative dose-dependent influence on the viability of osteoblasts. MTT assays performed at 48 hours with 0, 100, 500, and 1,000 µM alendronate concentrations revealed that the 100 µM concentration significantly reduced cell viability, with cell viability being approximately 80% of the control group. García-Moreno et al.14 reported that high concentrations of alendronate inhibit osteoblast proliferation in primary hFOB cells and indicated that at lower concentrations the drug did not significantly inhibit proliferative effects compared to controls (≤10-5 M). The results of the current study are in agreement with García-Moreno et al.14 and further suggest that alendronate at higher concentrations affects proliferation and viability of hFOB cells.
We determined that expression of OPG, RANKL and MCSF was significantly decreased in the alendronate-treated group. In contrast, in a human osteoblast-culture study, Koch et al.15 reported that zoledronate and ibandronate significantly induced RANKL expression.
Several studies have evaluated the impact of BPs on osteoclastogenesis. Sudhoff et al.16 reported that osteoclasts were suppressed and osteoclast apoptosis was induced in mouse bone-marrow cell (BMC) culture by the addition of M-CSF, RANKL and zolendronic acid. Kwak et al.17, having investigated a BMC/osteoblast co-culture, reported that risedronate inhibits osteoclast differentiation and suppresses RANKL and that addition of M-CSF mediates osteoclast differentiation in a bone-marrow-derived macrophage culture, with no direct BPs-cytoxicity effect on the osteoclasts.
Although the reasons for these differences have not been completely elucidated, the distinct effects of various BPs (pamidronate, zoledronate, and alendronate), use at different concentrations, and use of different cell lines (human vs. rat and primary vs. cancer) clearly play a role15,18
BMP-2 is well recognized as the most effective boneinduction treatment19,20,21,22,23 and has been approved by the U.S. Food and Drug Administration. There is evidence that BMP-2 can directly influence osteoclastogenesis through osteoblast/stromal cells24,25,26,27. Itoh et al.27 reported that BMP-2 enhanced the survival of purified osteoclasts supported by RANKL but not by M-CSF. In our present investigation, we found that a lower dose of rhBMP-2, 100 ng/mL, was able to induce osteoclast potency, indicating that at lower concentrations, BMP-2 can have osteoinductive potency28. The alendronate+rhBMP-2 group showed significant elevation in expression levels of RANKL and M-CSF relative to the alendronate alone group. Our results show that BMP-2 induced expression of M-CSF but that RANKL was otherwise suppressed by alendronate. This suggest that decreased RANKL expression by BPs can be corrected by treatment of BMP-2.
This study was conducted to establish whether alendronate and rhBMP-2 influence the mRNA levels and protein expression levels of M-CSF, RANKL, and OPG in osteoblasts. Our results indicate that alendronate suppressed the expression of M-CSF, RANKL, and OPG and that rhBMP-2 increased BP-impaired RANKL, M-CSF, and OPG expression levels. Together with the previous results, the present study provides an experimental basis for the clinical effects of rhBMP-2 as a BRONJ-treatment modality.
References
1. Marx RE. Pamidronate (Aredia) and zoledronate (Zometa) induced avascular necrosis of the jaws: a growing epidemic. J Oral Maxillofac Surg. 2003; 61:1115–1117. PMID: 12966493.


2. Kim HK, Kim JH, Abbas AA, Yoon TR. Alendronate enhances osteogenic differentiation of bone marrow stromal cells: a preliminary study. Clin Orthop Relat Res. 2009; 467:3121–3128. PMID: 18665432.


3. Aubin J, Liu F, Bilezikian J, Raisz L, Rodan G. Principles of bone biology. 2nd ed. San Diego: Academic Press;1996.
5. Reddi AH. Bone and cartilage differentiation. Curr Opin Genet Dev. 1994; 4:737–744. PMID: 7849513.


6. Reddi AH. Role of morphogenetic proteins in skeletal tissue engineering and regeneration. Nat Biotechnol. 1998; 16:247–252. PMID: 9528003.


7. Bostrom MP, Asnis P. Transforming growth factor beta in fracture repair. Clin Orthop Relat Res. 1998; (355 Suppl):S124–S131. PMID: 9917633.


8. Barnes GL, Kostenuik PJ, Gerstenfeld LC, Einhorn TA. Growth factor regulation of fracture repair. J Bone Miner Res. 1999; 14:1805–1815. PMID: 10571679.


9. Bessa PC, Casal M, Reis RL. Bone morphogenetic proteins in tissue engineering: the road from the laboratory to the clinic, part I (basic concepts). J Tissue Eng Regen Med. 2008; 2:1–13. PMID: 18293427.


10. Bessa PC, Casal M, Reis RL. Bone morphogenetic proteins in tissue engineering: the road from laboratory to clinic, part II (BMP delivery). J Tissue Eng Regen Med. 2008; 2:81–96. PMID: 18383454.


11. Chen D, Zhao M, Mundy GR. Bone morphogenetic proteins. Growth Factors. 2004; 22:233–241. PMID: 15621726.


12. Marx RE. Clinical concerns of alendronate use. J Oral Maxillofac Surg. 2008; 66:1322. PMID: 18486814.


13. Sahni M, Guenther HL, Fleisch H, Collin P, Martin TJ. Bisphosphonates act on rat bone resorption through the mediation of osteoblasts. J Clin Invest. 1993; 91:2004–2011. PMID: 8486770.


14. García-Moreno C, Serrano S, Nacher M, Farré M, Díez A, Mariñoso ML, et al. Effect of alendronate on cultured normal human osteoblasts. Bone. 1998; 22:233–239. PMID: 9580147.


15. Koch FP, Merkel C, Ziebart T, Smeets R, Walter C, Al-Nawas B. Influence of bisphosphonates on the osteoblast RANKL and OPG gene expression in vitro. Clin Oral Investig. 2012; 16:79–86.


16. Sudhoff H, Jung JY, Ebmeyer J, Faddis BT, Hildmann H, Chole RA. Zoledronic acid inhibits osteoclastogenesis in vitro and in a mouse model of inflammatory osteolysis. Ann Otol Rhinol Laryngol. 2003; 112:780–786. PMID: 14535562.


17. Kwak HB, Kim JY, Kim KJ, Choi MK, Kim JJ, Kim KM, et al. Risedronate directly inhibits osteoclast differentiation and inflammatory bone loss. Biol Pharm Bull. 2009; 32:1193–1198. PMID: 19571384.


18. Idris AI, Rojas J, Greig IR, Van't Hof RJ, Ralston SH. Aminobisphosphonates cause osteoblast apoptosis and inhibit bone nodule formation in vitro. Calcif Tissue Int. 2008; 82:191–201. PMID: 18259679.


19. Suh DY, Boden SD, Louis-Ugbo J, Mayr M, Murakami H, Kim HS, et al. Delivery of recombinant human bone morphogenetic protein-2 using a compression-resistant matrix in posterolateral spine fusion in the rabbit and in the non-human primate. Spine (Phila Pa 1976). 2002; 27:353–360. PMID: 11840099.


20. Kübler NR, Würzler KK, Reuther JF, Sieber E, Kirchner T, Sebald W. Effect of different factors on the bone forming properties of recombinant BMPs. Mund Kiefer Gesichtschir. 2000; (4 Suppl 2):S465–S469. PMID: 11094517.
21. Uludag H, D'Augusta D, Palmer R, Timony G, Wozney J. Characterization of rhBMP-2 pharmacokinetics implanted with biomaterial carriers in the rat ectopic model. J Biomed Mater Res. 1999; 46:193–202. PMID: 10379997.


22. Linde A, Hedner E. Recombinant bone morphogenetic protein-2 enhances bone healing, guided by osteopromotive e-PTFE membranes: an experimental study in rats. Calcif Tissue Int. 1995; 56:549–553. PMID: 7648485.


23. Yamamoto M, Takahashi Y, Tabata Y. Controlled release by biodegradable hydrogels enhances the ectopic bone formation of bone morphogenetic protein. Biomaterials. 2003; 24:4375–4383. PMID: 12922150.


24. Toth JM, Boden SD, Burkus JK, Badura JM, Peckham SM, McKay WF. Short-term osteoclastic activity induced by locally high concentrations of recombinant human bone morphogenetic protein-2 in a cancellous bone environment. Spine (Phila Pa 1976). 2009; 34:539–550. PMID: 19240666.


25. Kanatani M, Sugimoto T, Kaji H, Kobayashi T, Nishiyama K, Fukase M, et al. Stimulatory effect of bone morphogenetic protein-2 on osteoclast-like cell formation and bone-resorbing activity. J Bone Miner Res. 1995; 10:1681–1690. PMID: 8592944.


26. Kaneko H, Arakawa T, Mano H, Kaneda T, Ogasawara A, Nakagawa M, et al. Direct stimulation of osteoclastic bone resorption by bone morphogenetic protein (BMP)-2 and expression of BMP receptors in mature osteoclasts. Bone. 2000; 27:479–486. PMID: 11033442.


27. Itoh K, Udagawa N, Katagiri T, Iemura S, Ueno N, Yasuda H, et al. Bone morphogenetic protein 2 stimulates osteoclast differentiation and survival supported by receptor activator of nuclear factorkappaB ligand. Endocrinology. 2001; 142:3656–3662. PMID: 11459815.
28. Zhu W, Kim J, Cheng C, Rawlins BA, Boachie-Adjei O, Crystal RG, et al. Noggin regulation of bone morphogenetic protein (BMP) 2/7 heterodimer activity in vitro. Bone. 2006; 39:61–71. PMID: 16488673.


Fig. 2
Effect of alendronate and rhBMP-2 on OPG expression. ELISA of OPG expression. (rhBMP-2: recombinant human bone morphogenetic protein-2, OPG: osteoprotegerin, ELISA: enzymelinked immunosorbent assay)
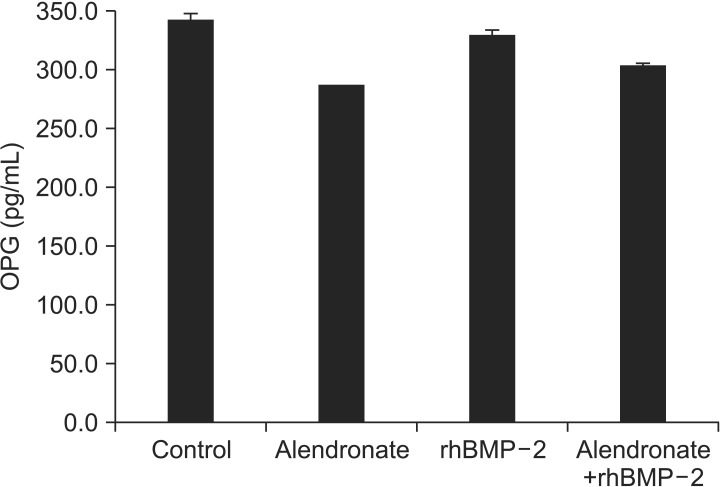
Fig. 3
Effect of alendronate and rhBMP-2 on RANKL expression. ELISA of RANKL expression. (rhBMP-2: recombinant human bone morphogenetic protein-2, RANKL: receptor activator of nuclear factor-κB ligand, ELISA: enzyme-linked immunosorbent assay)
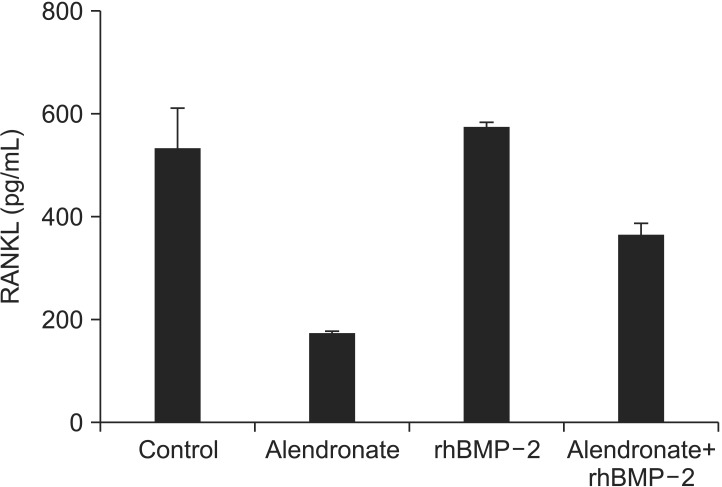
Fig. 4
Effect of alendronate and rhBMP-2 on M-CSF expression. ELISA of M-CSF expression. (rhBMP-2: recombinant human bone morphogenetic protein-2, M-CSF: macrophage colony-stimulating factor, ELISA: enzyme-linked immunosorbent assay)
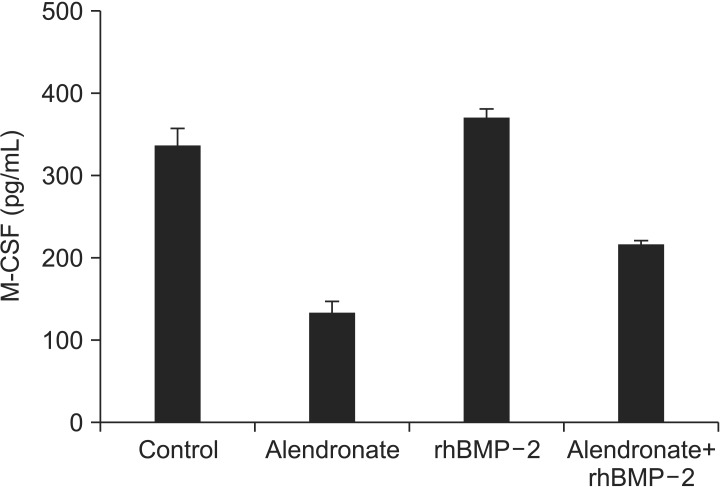
Table 2
Effects of alendronate and recombinant human bone morphogenetic protein-2 (rhBMP-2) on cell viability
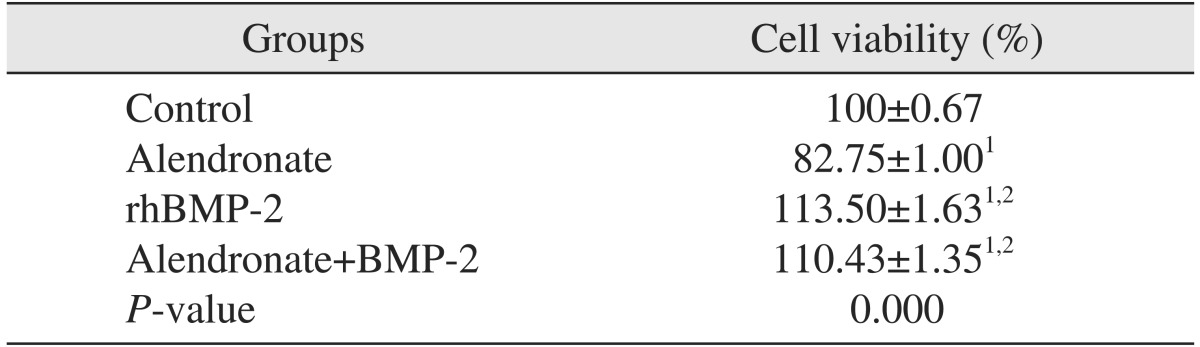
Table 3
Expression of OPG, RANKL and M-CSF in RT-PCR

(OPG: osteoprotegerin, RANKL: receptor activator of nuclear factor-κB ligand, M-CSF: macrophage colony-stimulating factor, RT-PCR: real-time polymerase chain reaction, rhBMP-2: recombinant human bone morphogenetic protein-2)
1Statistically significant (P<0.05) compared with control group.
2Statistically significant (P<0.05) compared with alendronate group.
Values are presented as mean±standard deviation.