Abstract
Objectives
The objective of this study is to determine the adequate concentration and to evaluate the osteogenic potential of simvastatin in human maxillary sinus membrane-derived stem cells (hSMSC).
Materials and Methods
Mesenchymal stem cells derived from the human maxillary sinus membrane were treated with various concentrations of simvastatin. The adequate concentration of simvastatin for osteogenic induction was determined using bone morphogenetic protein (BMP-2). The efficacy of osteogenic differentiation of simavastatin was verified using osteocalcin mRNA, and the mineralization efficacy of hSMSCs and simvastatin treatment was compared with alkaline phosphatase and von Kossa staining.
Results
Expression of BMP-2 mRNA and protein was observed after three days and was dependent on the concentration of simvastatin. Expression of osteocalcin mRNA was observed after three days in the 1.0 µM simvastatin-treated group. Mineralization was observed after three days in the simvastatin-treated group.
Bone graft is one of the most common treatment modalities for bony defects. Note, however, that this method has many disadvantages, including insufficient bone volume, donor site morbidity, bone resorption, and discomfort. Still, recent studies based on multipotent stem cells have shown new possibilities for the treatment of bony defects. Hematopoietic stem cells (HSCs) have been reported to be able to make skeletal muscle1,2, cardiac muscle3,4, and liver5 because they can differentiate into adipocytes1, condrocytes6,7, myocytes1,8 and osteoblast7,9, but there are ethical and surgical limitations6,10. In contrast, mesenchymal stem cells (MSCs) can overcome these limitations11,12.
Recently, MSCs in the human maxillary sinus membrane have been known to differentiate to osteoblasts under some conditions. Gruber et al.11 reported that porcine sinus mucosa cells were positive for STRO-1 and alkaline phosphatase (ALP) activity. They concluded that the sinus mucosa holds mesenchymal progenitor cells committed to the osteogenic lineage by responding to bone morphogenic protein (BMP)-6 and BMP-7. According to Kim et al.13, MSCs in the human maxillary sinus membrane were differentiated to osteoblasts under osteogenic induction. They found that those cells expressed ALP and osteocalcin (OC). Note, however, that there are controversies regarding MSCs' biological safety and effectiveness.
Statin is an inhibitor in the reduction of 3-hydroxy-3-methylglutaryl coenzyme A (HMG Co-A) and is used for lowering serum cholesterol14. Recently, statin has been reported to be able to enhance bone metabolism and new bone formation. Simvastatin increased cancellous bone volume when orally administered to rats14. It also increased cancellous bone mass and cancellous bone compressive strength15, and these results were related to the increased rate of new bone formation14,16.
As a protein that regulates the differentiation and function of osteoblasts and chondrocytes, BMP has a major role in bone formation17. After the adipose-derived stromal cells modified with the BMP-2 gene were implanted into ulnar defects in the canine model, these cells produced significant new bone18. BMP-2 and vitamin D(3) had synergistic effect on the osteogenic differentiation of adipose stem cells19. For these reasons, osteogenic differentiation of human MSCs has been evaluated with BMP-2 mRNA expression levels20,21.
We hypothesized that simvastatin wields a beneficial effect on the osteogenic potential of human maxillary sinus membrane-derived stem cells (hSMSCs). This study sought to evaluate the osteoinductive potential of simvastatin and osteogenic potential of MSCs derived from the human maxillary sinus membrane.
The subjects of this study were five adults, and approval was obtained from the institutional review board of College of Medicine, The Catholic University of Korea. Informed consent was provided to patients as per the 'Act on Legal Codes for Biomedical Ethics and Safety' and the Declaration of Helsinki.
Human maxillary sinus mucosal membrane (hMSM)-derived stem cells were isolated and cultivated in accordance with the author's previous study13. Maxillary sinus tissue was collected and contained in phosphate-buffered saline (PBS; Gibco BRL, Grand island, NY, USA) including 1% antibiotic and antimycotic (10× antibiotic & antimycotic, Gibco BRL) and transported to the laboratory under aseptic condition. It was then washed with PBS (Gibco BRL) including antibiotic and antimycotic, cut into small pieces with a mesh under aseptic condition, treated with 0.06% collagenase type II (Invitrogen, Carlsbad, CA, USA), shaken with an incubator (CO2 incubator; Forma Scientific, Marietta, OH, USA) containing 5% CO2 at 37℃ for 4 hours, and centrifuged at 1,000 rpm for 10 minutes (large-capacity table-top centrifuge; Hanil Science Industrial, Gangneung, Korea). The precipitate was suspended onto α-MEM (Sigma-Aldrich, St. Louis, MO, USA) containing 10% fetal bovine serum (Hyclone, Logan, UT, USA) and 1% Penicillin-Streptomycin (Gibco BRL) and filtered with 40 µm cell strainer (BD Bioscience, Bedford, MA, USA). Tissues except hMSM-derived cells were filtered, with the filtered out solution cultured in the incubator. The daily morphological characteristics were observed with inverted microscope, and the culture solution was replaced every other day. When the medium was changed, cells not adhering to the culture plate were removed; only the adhering cells were cultured. When culture dishes became near-confluent, cells were separated with Trypsin-EDTA (Gibco BRL) and subsequently repeated for continued passaging. The cells were cultured at passage 3.
Colony-forming unit-fibroblast assay was used to evaluate MSC presence in hMSM. When the initially cultured cells became near confluent, cells were separated with Trypsin-EDTA, plated at 1×104 cells/mL onto the 100 mm culture plate, stained with hematoxilin (Sigma-Aldrich) after 24 hours, and observed with inverted microscope.
Flow cytometry on cell surface markers STRO-1, CD105, and CD34 for the separation of MSCs from hMSM was conducted. The hMSM-derived cells at passage 3 were plated into a test tube (Bectom Dickinson, Franklin Larks, NJ, USA) by 1×104 cells/mL. The antibodies of CD34 (BD Bioscience) and CD105 (Serotec, Oxford, UK) - to which fluorescein isothicyanate (FITC) and phycoerythrin were adhered - were treated for 1 hour and washed with wash buffer 3 times, and the origin of stem cell was observed with flow cytometry (BD Bioscience). For STRO-1 (human anti-mouse monoclonal antibody, IgM subclass; R&D system, Minneapolis, MA, USA), antibody was treated for 1 hour and washed 3 times with wash buffer; the secondary antibody to which FITC was attached was treated for 30 minutes, washed 3 times with wash buffer, and observed with flow cytometry.
Sinus mucosa-derived stem cells were washed with PBS 72 hours after treatment on normal media and simvastatin (0.1, 0.2, 0.5, 1.0, 2.0 µM)-treated media. Afterward, the cells were collected with cell scraper (Sarsted, Newton, NC, USA). After the total RNA was separated by QIAGEN RNA extraction kit (QIAGEN, Hilden, Germany), it underwent reverse transcription reaction with the separated RNA, sense primer, antisense primer, and reverse transcriptase-polymerase chain reaction (RT-PCR) premix (Bioneer, Daejeon, Korea) for 60 minutes at 42℃ and 5 minutes at 94℃ and denatured for 5 minutes at 94℃ and 1 minute at 94℃, and genes were annealed for 90 seconds at 52℃, elongated for 1 minute at 72℃, and then annealed and elongated repeatedly 30 times and incubated for 5 minutes at 72℃.(Table 1)
Sinus mucosa-derived stem cells were washed with PBS 72 hours after treatment on normal media and simvastatin (0.1, 0.2, 0.5, 1.0, 2.0 µM)-treated media. The cells were then collected with cell scraper (Sarsted) and spun at 12,000 rpm for 20 minutes at 4℃ after being rinsed with RIPA-B buffer (0.5% Nonidet P-40, 20 mM Tris, pH 8.0, 50 mM NaCl, 50 mM NaF, 100 µM Na3VO4, 1 mM DTT, 50 µg/mL PMSF) for 1 hour at 4℃. The supernatant was transferred to the nitrocellulose membrane, blocked with TTBS (1 M Tris, 1.5 M NaCl, 500 µL Tween 20) containing 5% skim milk, and treated by BMP-2 primary antibody and secondary antibody. Afterward, the results were detected by the radioactive system.
Sinus mucosa-derived stem cells were cultured 3, 6, 9, and 12 days after treatment on normal media and determined concentrated simvastatin-treated media. After the total RNA was separated with QIAGEN RNA extraction kit, it underwent reverse transcription reaction with the separated RNA, OC sense primer, antisense primer, and RT-PCR premix for 60 minutes at 42℃ and 5 minutes at 94℃ and denatured for 5 minutes at 94℃ and 1 minute at 94℃; genes were annealed for 90 seconds at 52℃, elongated for 1 minute at 72℃, and then annealed and elongated repeatedly 30 times and incubated for 5 minutes at 72℃.(Table 1)
The control and simvastatin-treated group were each washed 3 times with sterile triple-distilled water 3, 6, 9, and 12 days after the treatment date, fixed with citrate-acetone-formaldehyde fixer solution (Sigma-Aldrich), and washed 3 times with sterile triple-distilled water. They were stained with alkaline-dye mix (Nitrite, FRV-alkaline, Naphthol ASBI alkaline solution; Sigma-Aldrich) with light isolated for 15 minutes at normal temperature, washed 3 times with sterile triple-distilled water, counterstained with hematoxylin, washed again 3 times with sterile triple-distilled water, and observed with inverted microscope. The specimens were observed by two separate observers.
All groups were washed 3 times with sterile triple-distilled water 3, 6, 9, and 12 days after the treatment date. All groups were fixed with 4% paraformaldehyde (Merck & Co., Inc., Darmstadt, Germany) at normal temperature for 15 minutes and washed again 3 times with sterile triple-distilled water. They were stained for 30 minutes with light isolated with 1% silver nitrate (Merck) solution, washed 3 times with sterile triple-distilled water, left for 1 hour under ultraviolet, counterstained with 0.1% eosin (Sigma-Aldrich), and observed with inverted microscope. The specimens were observed by two separate observers.
The subjects of this study were five adults (mean age: 20 years old). There were no special events during surgery and at all experimental periods.
BMP-2 mRNA was expressed on the simvastatin group after 72 hours, with the highest expression on the 1.0 and 2.0 µM groups.(Fig. 1) BMP-2 protein was also expressed on the 0.5, 1.0, and 2.0 µM simvastatin groups.(Fig. 2) BMP-2 mRNA and protein were not expressed on the control and SMSCs only group. The adequate concentration of simvastatin for this experiment was determined to be 1.0 µM.
Dental implant is one of the most effective treatment methods for oral rehabilitation. Still, it has to be modified because of unfavorable environments such as excessive alveolar bone loss and sinus pneumatization. To improve such unfavorable environment, bone graft is considered to be the treatment of choice. Especially, maxillary sinus grafting is a standard procedure with dental implant in the posterior maxilla22, and autogenous and various artificial bone substitutes are used in this procedure. Autogenous bone has many disadvantages, e.g., insufficient bone volume, donor site morbidity, bone resorption, and discomfort, even as it is an ideal graft material. Artificial bone graft materials are cell-free, requiring more time for bone healing despite being more convenient to use than autogenous bone22.
Recent studies show that multi-potent stem cells can replace bone graft for the treatment of bony defects23-26. Embryonic stem cells may have greater potential27,28, but these cells have some limitations such as bioethical consideration and uncontrolled differentiation. MSCs are adult stem cells with self-renewal capacity and multi-potentiality of being differentiated into multiple mesenchymal cell lineages29-31. It also overcomes any bioethical hindrance of the embryonic stem cell. Different types of MSCs and combination methods with various osteoconductive scaffolds have been examined for their potential application in bone regeneration22. Most of MSCs are generally isolated from bone marrow aspirates harvested from the iliac bone, periosteum of the mandible, or maxillary tuberosity and adipose tissue22. MSCs are also isolated from the maxillary sinus mucosal membrane11,13. MSCs from hMSM showed calcified nodule 14 days after being cultured in osteogenic media13. Note, however, that MSCs in normal media did not show any calcified nodule and OC mRNA. These results suggest that MSCs itself may have not osteogenic potential, and that combination methods with MSCs and osteoconductive scaffold induce new bone formation.
Several studies suggested the beneficial effects of simvastatin on bone formation. Song et al.32 investigated the effect of simvastatin, a widely used statin, on osteoblastic and adipocytic differentiation in primary cultured mouse bone marrow stromal cells. According to them, simvastatin enhanced the expression level of mRNA for OC and protein for OC and osteopontin, increased alkaline phosphatase activity significantly, and inhibited adipocytic differentiation. It was suggested that statin induced the apoptosis of osteoclast and enhanced the differentiation of osteoblast through the inhibition of the mevalonate pathway in cholesterol metabolism and blockade of guanosine-5'-triphosphatase and prenylation32. BMP-2 mRNA in human osteoblast-like cells was stimulated by simvastatin14. The OC mRNA and ALP activity of human alveolar osteoblasts and periodontal ligament cells was also increased by simvastatin33. Rat mandibular defects were regenerated, increasing bone density after graft with simvastatin34. Some clinical studies reported that the long-term administration of simvastatin is correlated with increasing bone mineral density and with decreasing bone fracture35,36.
This study has some limitations since quantitative experiments were not performed because of the preliminary study; hence the need for quantitative analysis and evaluation of relative osteogenic potential of simvastatin.
In this study, the authors tested the effect of simvastatin on maxillary sinus mucosal stem cells. MSCs were treated with simvastatin. The osteogenic potential of stem cells and simvastatin was evaluated with RT-PCR, and the mineralization of extra cellular matrix was verified by ALP expression and von Kossa staining. We found that BMP-2 was much more expressed in 1 µM simvastatin than the other concentrations. 1 µM simvastatin enhanced the expression of OC mRNA and mineralization. OC mRNA was expressed on the 3rd day in this study. The authors' previous study showed that MSCs and osteogenic media expressed OC mRNA after 1 week, and that mineralization was detected after 2 weeks13. Similarly, MSCs did not express OC mRNA and mineralization in this study. These results suggest that the combination of stem cells and simvastatin enhance new bone formation, and that this method may be a useful treatment of bony defects.
References
1. Ferrari G, Cusella-De Angelis G, Coletta M, Paolucci E, Stornaiuolo A, Cossu G, et al. Muscle regeneration by bone marrow-derived myogenic progenitors. Science. 1998; 279:1528–1530. PMID: 9488650.


2. Gussoni E, Soneoka Y, Strickland CD, Buzney EA, Khan MK, Flint AF, et al. Dystrophin expression in the mdx mouse restored by stem cell transplantation. Nature. 1999; 401:390–394. PMID: 10517639.


3. Kocher AA, Schuster MD, Szabolcs MJ, Takuma S, Burkhoff D, Wang J, et al. Neovascularization of ischemic myocardium by human bone-marrow-derived angioblasts prevents cardiomyocyte apoptosis, reduces remodeling and improves cardiac function. Nat Med. 2001; 7:430–436. PMID: 11283669.


4. Orlic D, Kajstura J, Chimenti S, Jakoniuk I, Anderson SM, Li B, et al. Bone marrow cells regenerate infarcted myocardium. Nature. 2001; 410:701–705. PMID: 11287958.


5. Alison MR, Poulsom R, Jeffery R, Dhillon AP, Quaglia A, Jacob J, et al. Hepatocytes from non-hepatic adult stem cells. Nature. 2000; 406:257. PMID: 10917519.


7. Johnstone B, Hering TM, Caplan AI, Goldberg VM, Yoo JU. In vitro chondrogenesis of bone marrow-derived mesenchymal progenitor cells. Exp Cell Res. 1998; 238:265–272. PMID: 9457080.
8. Wakitani S, Saito T, Caplan AI. Myogenic cells derived from rat bone marrow mesenchymal stem cells exposed to 5-azacytidine. Muscle Nerve. 1995; 18:1417–1426. PMID: 7477065.


9. Pittenger MF, Mackay AM, Beck SC, Jaiswal RK, Douglas R, Mosca JD, et al. Multilineage potential of adult human mesenchymal stem cells. Science. 1999; 284:143–147. PMID: 10102814.


10. Bruder SP, Jaiswal N, Haynesworth SE. Growth kinetics, self-renewal, and the osteogenic potential of purified human mesenchymal stem cells during extensive subcultivation and following cryopreservation. J Cell Biochem. 1997; 64:278–294. PMID: 9027588.


11. Gruber R, Kandler B, Fuerst G, Fischer MB, Watzek G. Porcine sinus mucosa holds cells that respond to bone morphogenetic protein (BMP)-6 and BMP-7 with increased osteogenic differentiation in vitro. Clin Oral Implants Res. 2004; 15:575–580. PMID: 15355400.


12. Haas R, Donath K, Födinger M, Watzek G. Bovine hydroxyapatite for maxillary sinus grafting: comparative histomorphometric findings in sheep. Clin Oral Implants Res. 1998; 9:107–116. PMID: 9663038.


13. Kim SW, Lee IK, Yun KI, Kim CH, Park JU. Adult stem cells derived from human maxillary sinus membrane and their osteogenic differentiation. Int J Oral Maxillofac Implants. 2009; 24:991–998. PMID: 20162102.
14. Mundy G, Garrett R, Harris S, Chan J, Chen D, Rossini G, et al. Stimulation of bone formation in vitro and in rodents by statins. Science. 1999; 286:1946–1949. PMID: 10583956.


15. Oxlund H, Dalstra M, Andreassen TT. Statin given perorally to adult rats increases cancellous bone mass and compressive strength. Calcif Tissue Int. 2001; 69:299–304. PMID: 11768201.


16. Oxlund H, Andreassen TT. Simvastatin treatment partially prevents ovariectomy-induced bone loss while increasing cortical bone formation. Bone. 2004; 34:609–618. PMID: 15050891.


17. Takahashi Y, Yamamoto M, Tabata Y. Enhanced osteoinduction by controlled release of bone morphogenetic protein-2 from biodegradable sponge composed of gelatin and beta-tricalcium phosphate. Biomaterials. 2005; 26:4856–4865. PMID: 15763265.
18. Li H, Dai K, Tang T, Zhang X, Yan M, Lou J. Bone regeneration by implantation of adipose-derived stromal cells expressing BMP-2. Biochem Biophys Res Commun. 2007; 356:836–842. PMID: 17391646.


19. Song I, Kim BS, Kim CS, Im GI. Effects of BMP-2 and vitamin D3 on the osteogenic differentiation of adipose stem cells. Biochem Biophys Res Commun. 2011; 408:126–131. PMID: 21463608.


20. Sumanasinghe RD, Bernacki SH, Loboa EG. Osteogenic differentiation of human mesenchymal stem cells in collagen matrices: effect of uniaxial cyclic tensile strain on bone morphogenetic protein (BMP-2) mRNA expression. Tissue Eng. 2006; 12:3459–3465. PMID: 17518682.


21. Cho HH, Park HT, Kim YJ, Bae YC, Suh KT, Jung JS. Induction of osteogenic differentiation of human mesenchymal stem cells by histone deacetylase inhibitors. J Cell Biochem. 2005; 96:533–542. PMID: 16088945.


22. Park JB. Use of cell-based approaches in maxillary sinus augmentation procedures. J Craniofac Surg. 2010; 21:557–560. PMID: 20216438.


23. Kimelman N, Pelled G, Helm GA, Huard J, Schwarz EM, Gazit D. Review: gene- and stem cell-based therapeutics for bone regeneration and repair. Tissue Eng. 2007; 13:1135–1150. PMID: 17516852.


24. Moioli EK, Clark PA, Xin X, Lal S, Mao JJ. Matrices and scaffolds for drug delivery in dental, oral and craniofacial tissue engineering. Adv Drug Deliv Rev. 2007; 59:308–324. PMID: 17499385.


25. Donzelli E, Salvadè A, Mimo P, Viganò M, Morrone M, Papagna R, et al. Mesenchymal stem cells cultured on a collagen scaffold: In vitro osteogenic differentiation. Arch Oral Biol. 2007; 52:64–73. PMID: 17049335.


26. Thesleff I, Tummers M. Stem cells and tissue engineering: prospects for regenerating tissues in dental practice. Med Princ Pract. 2003; 12(Suppl 1):43–50. PMID: 12707500.


27. Woll NL, Heaney JD, Bronson SK. Osteogenic nodule formation from single embryonic stem cell-derived progenitors. Stem Cells Dev. 2006; 15:865–879. PMID: 17253949.


28. Kärner E, Unger C, Sloan AJ, Ahrlund-Richter L, Sugars RV, Wendel M. Bone matrix formation in osteogenic cultures derived from human embryonic stem cells in vitro. Stem Cells Dev. 2007; 16:39–52. PMID: 17233553.


29. Jackson L, Jones DR, Scotting P, Sottile V. Adult mesenchymal stem cells: differentiation potential and therapeutic applications. J Postgrad Med. 2007; 53:121–127. PMID: 17495381.


30. Weiss ML, Troyer DL. Stem cells in the umbilical cord. Stem Cell Rev. 2006; 2:155–162. PMID: 17237554.


31. Gimble J, Guilak F. Adipose-derived adult stem cells: isolation, characterization, and differentiation potential. Cytotherapy. 2003; 5:362–369. PMID: 14578098.


32. Song C, Guo Z, Ma Q, Chen Z, Liu Z, Jia H, et al. Simvastatin induces osteoblastic differentiation and inhibits adipocytic differentiation in mouse bone marrow stromal cells. Biochem Biophys Res Commun. 2003; 308:458–462. PMID: 12914771.


33. Liu S, Bertl K, Sun H, Liu ZH, Andrukhov O, Rausch-Fan X. Effect of simvastatin on the osteogenetic behavior of alveolar osteoblasts and periodontal ligament cells. Hum Cell. 2012; 25:29–35. PMID: 22399266.


34. Ozeç I, Kiliç E, Gümüş C, Göze F. Effect of local simvastatin application on mandibular defects. J Craniofac Surg. 2007; 18:546–550. PMID: 17538316.
35. Edwards CJ, Hart DJ, Spector TD. Oral statins and increased bone-mineral density in postmenopausal women. Lancet. 2000; 355:2218–2219. PMID: 10881898.


36. Meier CR, Schlienger RG, Kraenzlin ME, Schlegel B, Jick H. HMG-CoA reductase inhibitors and the risk of fractures. JAMA. 2000; 283:3205–3210. PMID: 10866867.


Fig. 1
Effect of simvastatin on bone morphogenetic protein (BMP)-2 mRNA expression in human sinus membrane stem cells using reverse transcriptase-polymerase chain reaction. BMP-2 mRNA levels were measured after cells were stimulated with simvastatin for 72 hours.
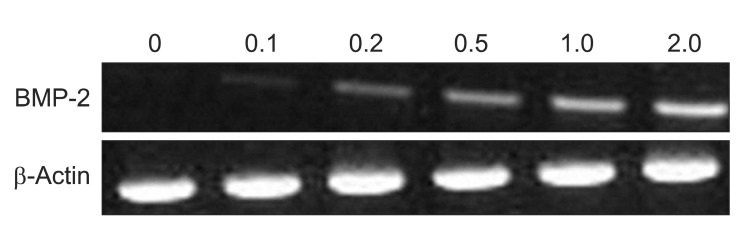
Fig. 2
Effect of simvastatin on bone morphogenetic protein (BMP)-2 protein expressions in human sinus membrane stem cells using Westen blot analysis. BMP-2 expression was measured after cells were stimulated with simvastatin for 72 hours.
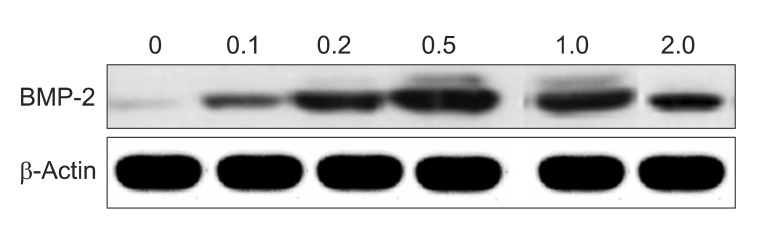
Fig. 3
Effect of simvastatin on osteocalcin mRNA expression in human sinus membrane stem cells using reverse transcriptase-polymerase chain reaction. Osteocalcin mRNA levels were measured after cells were stimulated with simvastatin for 72 hours.
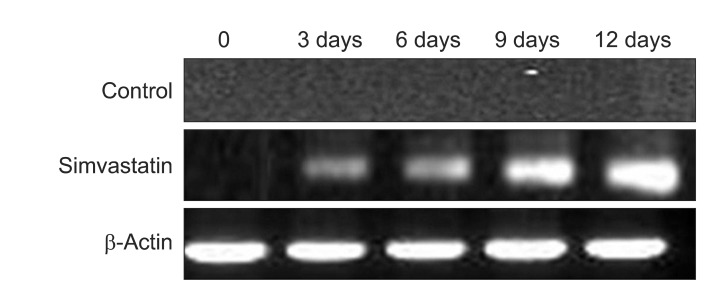