Abstract
Background
Macrophage migration inhibitory factor (MIF), a pro-inflammatory cytokine with chemokine-like functions, has been shown to play a central role in several acute and chronic inflammatory diseases. However, limited information is available regarding the use of MIF as an inflammatory pathway marker in patients with tuberculosis. This study aimed to investigate the association of MIF with IFN-γ and TNF-α in active pulmonary tuberculosis (APTB) following anti-tuberculosis treatment.
Methods
The MIF, TNF-α, and IFN-γ serum levels were determined in 47 patients with APTB by cytokine-specific ELISA at four phases: prior to anti-tuberculosis drug treatment (baseline), and following 2, 4, and 6 months of treatment. In addition, we measured the MIF, TNF-α, and IFN-γ serum levels in 50 health controls.
Results
MIF serum levels were significantly elevated (P<0.05) in patients with APTB prior to treatment compared with that in control subjects, and TNF-α ≥449.7 pg/mL was associated with high MIF levels (≥13.1 ng/mL). MIF levels were significantly reduced (P<0.01) following 2, 4, and 6 months of treatment, with variations in TNF-α and IFN-γ serum levels. MIF levels were positively correlated with the paired TNF-α level at baseline (r=0.1103, P=0.0316) and following 6 months of treatment (r=0.09569, P=0.0364).
Conclusions
A reduction in the MIF serum levels in patients with APTB following anti-tuberculosis treatment may positively affect host immune protection against Mycobacterium tuberculosis infection. Thus, serum MIF levels may constitute a useful marker for assessing therapy effectiveness in patients with APTB.
Macrophage migration inhibitory factor (MIF) was first described as a soluble factor released by activated T-lymphocytes in 1966 [1]. MIF, a pro-inflammatory cytokine with chemokine-like functions, has been shown to play a central role in several acute and chronic inflammatory diseases by promoting leukocyte recruitment [12345]. As an important pro-inflammatory cytokine, MIF may counter-regulate glucocorticoid effects by activating immune/inflammatory cells and promoting the expression of matrix metalloproteinases and the release of nitric oxide, prostaglandin E2 [6789], or pro-inflammatory and inflammatory cytokines, such as TNF-α, IL-1β, IL-2, IL-6, IL-8, and IFN-γ [10]. In addition, several studies have shown a correlation between the high expression of MIF alleles and improved outcomes of pneumonia and meningococcal meningitis and more severe outcomes of malaria [111213].
Host immune cells secrete a number of cytokines involved in the defense against Mycobacterium tuberculosis (M.tb) infection [14]; these cytokines play important roles in the initiation and regulation of the immune response during the different stages of tuberculosis (TB) development [15]. The interaction between infected macrophages and T lymphocytes is critical for protective immunity against M.tb and is mediated by a number of inflammatory cytokines produced by several cell types [16]. Following standard anti-tuberculosis drug (ATD) therapy, it is likely that cytokine-mediated cell signaling is altered, which ultimately leads to recovery of TB infection. Thus, assessing the association of the major cytokines at different stages of disease progression is necessary to elucidate the host response mechanism against M.tb. The major cytokines produced by immune cells during the effective phases of the immune response include IFN-γ, TNF-α, and MIF [17], which activate macrophages by potentiating their microbicidal mechanisms [18], including autophagy mechanisms involved in M.tb clearance [19], and maintaining the structural integrity of granulomas [20].
A number of groups have attempted to identify cytokines and other clinical characteristics in patients with TB in order to determine which of these could be used as biomarkers for TB development. Limited information is available regarding the use of MIF as an inflammatory pathway marker in patients with TB undergoing ATD therapy. Thus, the objective of this study was to assess the role of MIF associated with IFN-γ and TNF-α in active pulmonary tuberculosis (APTB) following ATD therapy.
A case-control study, including two study groups, was conducted from January 2015 to June 2016. The first group consisted of 47 patients with APTB enrolled from the Respiration Department of the Wuxi Fifth People's Hospital, China. The patients were diagnosed as having TB based on positive results for acid-fast bacilli (AFB) and a chest X-ray examination. For the control group, 50 healthy subjects were matched for analysis; they were identified by self-report and recruited from the general population in the same geographic area with negative T-SPOT.TB assays. The age between patients with APTB (median 44.6 years, range 28.7–72.3 years) and healthy controls (median 43.5 years, range 26.2–73.1 years) was not statistically different. The same result was the gender ratio of the two groups.
The clinical characteristics of 47 patients with APTB and 50 healthy controls are shown in Table 1. The median age of patients was 48 years; 57.4% of the patients were male. All patients enrolled in our study were receiving treatment for the first time. The control group included 50 healthy subjects (60% female and 40% male) with a median age of 43.5 (26.2–73.1) years. Eighteen (38.3%) patients had a history of smoking, 12 patients (17.6%) were HBV/HCV-positive patients, six (12.8%) patients had been diagnosed as having diabetes mellitus (DM), and four (8.5%) patients had cancer. Eleven patients with APTB had coexisting extra-pulmonary lesions, 29 patients were bacteriologically confirmed, 20 patients exhibited cavitation on initial chest X-ray (CXR), and only six patients presented with drug resistance.
Ethical approval and informed consent were obtained from all individuals prior to enrollment in the study, according to the ethical guidelines approved by the ethical committee of Wuxi 5th People's Hospital affiliated with Jiangnan University.
Serum samples were obtained from healthy controls and patients with APTB. Patient samples were obtained at four time-points: at first admission prior to any treatment, and following two, four, and six months of standard anti–TB treatment. The sera were collected following centrifugation and frozen immediately at -20℃ until analysis. MIF, IFN-γ, and TNF-α serum levels were determined using an ELISA Kit for humans (R&D Systems, Minneapolis, MN, USA), according to the manufacturer's instructions. Assays were repeated twice to obtain an average. Absorbance at 492 nm was measured using a spectrophotometer.
All data were statistically analyzed using SPSS 17.0 (SPSS, Inc., Chicago, IL, USA). The Pearson's chi-square test and Mann–Whitney U test were used to identify significant differences between groups; P<0.05 (two-sided) was considered as a statistically significant difference. The odds ratios (ORs) were estimated with 95% confidence intervals (95% CIs) from the bivariate analysis; P<0.05 was considered statistically significant. ROC curves were analyzed to determine the best cut-off value of each parameter. Spearman's correlation analysis was constructed to assess the relationship between MIF and other clinical characteristics; P<0.05 was considered statistically significant.
Prior to treatment, the average level of MIF (17.19 ng/mL vs 9.36 ng/mL, P<0.01), TNF-α (583.6 pg/mL vs 343.3 pg/mL, P<0.05), and IFN-γ (559.5 pg/mL vs 396.7 pg/mL, P<0.01) were significantly different between patients with APTB and healthy controls (Fig. 1). The best cut-off value was 13.1 ng/mL for MIF, 449.7 pg/mL for TNF-α, and 548.9 pg/mL for IFN-γ. The cutoff value MIF=13.1 ng/mL was used to divide the 47 patients with APTB into two groups (MIF level ≥13.1 ng/mL and MIF level <13.1 ng/mL). The cut-off values TNF-α=449.7 pg/mL and IFN-γ=548.9 pg/mL were used to examine whether different levels of TNF-α and IFN-γ were associated with high MIF levels. Thirty-nine (83%) of the patients with APTB had elevated pre-treatment MIF levels (≥13.1 ng/mL), and eight (17%) patients had lower MIF levels (<13.1 ng/mL) (Table 2). The 47 patients with APTB were divided into two groups based on MIF level to analyze the factors associated with MIF level. The results indicated that only levels of TNF-α≥449.7 pg/mL were associated with high MIF levels (OR=12.78, 95% CI, 1.326–123.1).
In patients with APTB, the serum levels of MIF, TNF-α, and IFN-γ significantly decreased (P<0.01) over the six months of ATD treatment (Fig. 2), reaching or approaching values close to those of the healthy controls. The median serum baseline MIF level was 17.19 (12.71–21.67) ng/mL, and MIF level decreased to 14.57 (11.98–16.98) ng/mL following two months of ATD therapy. MIF levels further decreased to 9.79 (8.17–12.35) ng/mL and 7.84 (6.34–8.70) ng/mL following four and six months of ATD therapy, respectively. Taken together, these findings indicate that there was a rapid decrease in serum MIF levels between zero to six months of ATD therapy; similar changes were observed for the serum levels of TNF-α and IFN-γ. Interestingly, six patients presented with drug resistance (one or more of isoniazid, rifampicin, pyrazinamide, and ethambutol) following six months of ATD therapy; their average MIF levels (12.53 ng/mL) remained significantly higher (P<0.0001) than that of the other patients (7.16 ng/mL).
We next analyzed the correlation between MIF and IFN-γ serum levels in the patient groups at baseline and following two, four, and six months of ATD therapy. All correlations for the serum levels of MIF and IFN-γ showed P values higher than 0.05 (Fig. 3). However, a positive correlation was observed between MIF and TNF-α serum levels at baseline and following six months of therapy in the APTB group (P<0.05) (Fig. 3).
Understanding the immune mechanisms responsible for the outcomes of M.tb infection is crucial for developing improved preventive and therapeutic strategies. To date, MIF-related macrophage responses and the role of pro-inflammatory activation in TB has not been studied.
In this study, we compared two groups: 47 patients with APTB and 50 healthy controls from Eastern China. The MIF, TNF-α, and IFN-γ serum levels in the 47 patients with APTB were assessed during six-month period of ATD treatment. We also determined the MIF, TNF-α, and IFN-γ serum levels in 50 healthy controls. At baseline, the patients with APTB had highly elevated serum levels of MIF, TNF-α, and IFN-γ, and following ATD therapy, their levels rapidly decreased to near normal values. As previously reported, a number of pro-inflammatory cytokines including MIF, TNF-α, and IFN-γ are excessively secreted following M.tb infection from host macrophages [21], and elevated circulating MIF can activate peripheral blood mononuclear cells to promote the production of inflammatory mediators, thereby enhancing the inflammatory response [22]. The dramatic response of serum MIF during ATD treatment was evident, indicating that MIF can be used as a marker (similar to TNF-α and IFN-γ) for assessing therapy effectiveness in patients with APTB. Moreover, patients with severe TB who did not respond to ATD therapy maintained high MIF levels; this finding further supports the use of MIF as a marker.
We attempted to further elucidate the role of MIF in patients with APTB by studying the relationship between MIF and other inflammatory biomarkers. Interestingly, we observed a positive correlation between serum MIF and TNF-α levels in patients with APTB at baseline and following six months of ATD therapy; TNF-α ≥449.7 pg/mL was associated with high MIF levels (≥13.1 ng/mL). However, a similar association was not observed for MIF and IFN-γ levels. MIF is functionally distinguished from other cytokines by its upstream immune regulatory action on immune cells [23]. The relationship between MIF and TNF-α suggests a possible role for MIF in the activation of TNF-α inflammation. TNF-α can be secreted by multiple immune cells, including dendritic cells, T cells, macrophages, and neutrophils [24], and has multiple functions [2526]. Several studies have reported that TNF-α is required for the formation and maintenance of granuloma integrity [27282930]. This role has been suggested as an explanation for the reactivation of TB against TNF-α [293132] and is consistent with the theory that granulomas benefit the host by containing and controlling M.tb [26]. In fact, previous studies have demonstrated decreased production of TNF-α in patients with multidrug-resistant TB [3334].
Several limitations in our study merit consideration. First, the patients in our study were hospital-based and were recruited from the region of Jiangsu Province, China; thus, selection bias cannot be eliminated. Second, the quantification of cytokines in serum by ELISA may not accurately reflect the increased expression of MIF in inflammation sites; therefore, these findings must be interpreted with caution. Third, TB severity, including symptoms, course of APTB, and sputum bacteriological factor, might influence the levels of some cytokines. Thus, further investigation of the immune function in patients with APTB is required to clarify immune pathogenesis and provide the rationale for immune-based interventions.
In conclusion, our findings indicate that a reduction in the MIF serum levels in patients with APTB following anti-TB treatment might play a positive role in host immune protection against M.tb infection. Moreover, serum MIF levels may constitute a useful marker for assessing therapy effectiveness in patients with APTB.
Acknowledgements
This study was supported by research grants from the key Program of Wuxi Administration of Science & Technology, China (No. CSE31N1510) and the research foundation of Wuxi Healthy Bureau, China (No. ML201310).
Notes
References
1. Calandra T, Roger T. Macrophage migration inhibitory factor: a regulator of innate immunity. Nat Rev Immunol. 2003; 3:791–800. PMID: 14502271.
2. Gregory JL, Morand EF, Mckeown SJ, Ralph JA, Hall P, Yang YH, et al. Macrophage migration inhibitory factor induces macrophage recruitment via CC chemokine ligand 2. J Immunol. 2006; 177:8072–8079. PMID: 17114481.
3. Tillmann S, Bernhagen J, Noels H. Arrest functions of the MIF ligand/receptor axes in atherogenesis. Front Immunol. 2013; 4:115. PMID: 23720662.
4. Santos LL, Morand EF. Macrophage migration inhibitory factor: a key cytokine in RA, SLE and atherosclerosis. Clin Chim Acta. 2013; 399:1–7.
5. Morand EF, Leech M, Bemhagen J. MIF: a new cytokine link between rheumatoid arthritis and atherosclerosis. Nat Rev Drug Discov. 2006; 5:399–410. PMID: 16628200.
6. Baugh JA, Bucala R. Macrophage migration inhibitory factor. Crit Care Med. 2002; 30:S27–S35.
7. Lolis E, Bucala R. Macrophage migration inhibitory factor. Expert Opin Ther Targets. 2003; 7:153–164. PMID: 12667094.
8. Leng L, Bucala R. Macrophage migration inhibitory factor. Critical Care Med. 2005; 33:S475–S477. PMID: 16340426.
9. Coban N, Onat A, Yildirim O, Can G, Erginelunaltuna N. Oxidative stress-mediated (sex-specific) loss of protection against type-2 diabetes by macrophage migration inhibitory factor (MIF)-173G/C polymorphism. Clin Chim Acta. 2015; 438:1–6. PMID: 25108206.
10. Calandra T, Bernhagen J, Metz CN, Spiegel LA, Bacher M, Donnelly T, et al. MIF as a glucocorticoid-induced modulator of cytokine production. Nature. 1995; 377:68–71. PMID: 7659164.
11. Awandare GA, Martinson JJ, Were T, Ouma C, Davenport GC, Ong'echa JM, et al. MIF (macrophage migration inhibitory factor) promoter polymorphism and susceptibility to severe malarial anemia. J Infect Dis. 2009; 200:629–637. PMID: 19591577.
12. Yende S, Angus DC, Kong L, Kellum JA, Weissfeld L, Ferrell R, et al. The influence of macrophage migration inhibitory factor gene polymorphism on outcome from community-acquired pneumonia. FASEB J. 2009; 23:2403–2411. PMID: 19346297.
13. Renner P, Roger T, Bochud PY, Sprong T, Sweep FC, Bochud M, et al. A functional microsatellite of the macrophage migration inhibitory factor gene associated with meningococcal disease. FASEB J. 2012; 26:907–916. PMID: 21990375.
14. Cooper AM, Khader SA. The role of cytokines in the initiation, expansion, and control of cellular immunity to tuberculosis. Immunol Rev. 2008; 226:191–204. PMID: 19161425.
15. Ameglio F, Casarini M, Capoluongo E, Mattia P, Puglisi G, Giosue S. Post-treatment changes of six cytokines in active pulmonary tuberculosis: differences between patients with stable or increased fibrosis. Int J Tuberc Lung Dis. 2005; 9:98–104. PMID: 15675558.
16. Munk ME, Emoto M. Functions of T-cell subsets and cytokines in mycobacterial infections. Eur Respir J. 1995; 20:668–675.
17. Boom WH, Canaday DH, Fulton SA, Gehring AJ, Rojas RE, Torres M. Human immunity to M. tuberculosis: T cell subsets and antigen processing. Tuberculosis. 2003; 83:98–106. PMID: 12758197.
18. Denis M. Interferon-gamma-treated murine macrophages inhibit growth of tubercle bacilli via the generation of reactive nitrogen intermediates. Cell Immunol. 1991; 132:150–157. PMID: 1905984.
19. Gutierrez MG, Master SS, Singh SB, Taylor GA, Colombo MI, Deretic V. Autophagy is a defense mechanism inhibiting BCG and Mycobacterium tuberculosis survival in infected macrophages. Cell. 2004; 119:753–766. PMID: 15607973.
20. Jacobs M, Brown N, Allie N, Rvffel B. Fatal Mycobacterium bovis BCG infection in TNF-LT-alpha –deficient mice. Clin Immunol. 2000; 94:192–199. PMID: 10692238.
21. Van CR, Ottenhoff TH, Vander Meer JW. Innate immunity to Mycobacterium tuberculosis. Clin Microbiol Rev. 2002; 15:294–309. PMID: 11932234.
22. White DA, Fang L, Chan W, Morand EF, Kiriazis H, Duffy SJ, et al. Proinflammatory action of MIF in acute myocardial infarction via activation of peripheral blood mononuclear cells. PloS One. 2013; 8:e76206. PMID: 24098445.
23. Llamas-Covarrubias MA, Valle Y, Bucala R, Navarro-Hernandez RE, Palafox-Sanchez CA, Padilla-Gutierrez JR, et al. Macrophage migration inhibitory factor (MIF): genetic evidence for participation in early onset and early stage rheumatoid arthritis. Cytokine. 2013; 61:759–765. PMID: 23402792.
24. Vassalli P. The pathophysiology of tumor necrosis factors. Annu Rev Immunol. 1992; 10:411–452. PMID: 1590993.
25. Pasparakis M, Alexopoulou L, Douni E, Kollias G. Tumour necrosis factors in immune regulation: everything that's interesting is. . .new! Cytokine Growth Factor Rev. 1996; 7:223–229. PMID: 8971477.
26. Flynn JL, Chan J. Immunology of tuberculosis. Annu Rev Immunol. 2001; 19:93–129. PMID: 11244032.
27. Berry MP, Graham CM, McNab FW, Bioch SA, Oni T, Wilkinson KA, et al. An interferon-inducible neutrophil-driven blood transcriptional signature in human tuberculosis. Nature. 2010; 466:973–977. PMID: 20725040.
28. Bean AG, Roach DR, Briscoe H, France MP, Korner H, SedqwicK JD, et al. Structural deficiencies in granuloma formation in TNF gene-targeted mice underlie the heightened susceptibility to aerosol Mycobacterium tuberculosis infection, which is not compensated for by lymphotoxin. J Immunol. 1999; 162:3504–3511. PMID: 10092807.
29. Chakravarty SD, Zhu G, Tsai MC, Mohan VP, Marino S, Kirschner DE, et al. Tumor necrosis factor blockade in chronic murine tuberculosis enhances granulomatous inflammation and disorganizes granulomas in the lungs. Infect Immun. 2008; 76:916–926. PMID: 18212087.
30. Roach DR, Briscoe H, Saunders B, France MP, Riminton S, Britton WJ. Secreted lymphotoxin-α is essential for the control of an intracellular bacterial infection. J Exp Med. 2001; 193:239–246. PMID: 11208864.
31. Botha T, Ryffel B. Reactivation of latent tuberculosis infection in TNF-deficient mice. J Immunol. 2003; 171:3110–3118. PMID: 12960337.
32. Mohan VP, Scanga CA, Yu K, Scottd HM, Tanaka KE, Tsanq E, et al. Effects of tumor necrosis factor α on host immune response in chronic persistent tuberculosis: possible role for limiting pathology. Infect Immun. 2001; 69:1847–1855. PMID: 11179363.
33. Smith SM, Klein MR, Malin AS, Sillah J, Mcadam KP, Dockrell HM, et al. Decreased IFN-gamma and increased IL-4 production by human CD8(+) T cells in response to Mycobacterium tuberculosis in tuberculosis patients. Tuberculosis. 2002; 82:7–13. PMID: 11914057.
34. Sharma S, Sharma M, Roy S, Kumar P, Bose M. Mycobacterium tuberculosis induces high production of nitric oxide in coordination with production of tumor necrosis factor-alpha in patients with fresh active tuberculosis but not in MDR tuberculosis. Immunol Cell Biol. 2004; 82:377–382. PMID: 15283847.
Fig. 1
Serum levels of MIF, TNF-α, and IFN-γ in 47 patients with active pulmonary TB and 50 control subjects. (A) MIF levels in patients with TB (17.19 ng/mL [12.71–21.67] and in control subjects (9.36 ng/mL [7.29–11.43]. (B) TNF-α levels in patients with TB (583.6 pg/mL [453.1–844.5] and in control subjects (343.3 pg/mL [298.6–434.2]. (C) IFN-γ levels in patients with TB (559.5 pg/mL [255.0–864.0] and in control subjects (396.7 pg/mL [189.5–603.9]. Data are presented as median values (interquartile range).
Abbreviation: MIF, macrophage migration inhibitory factor.
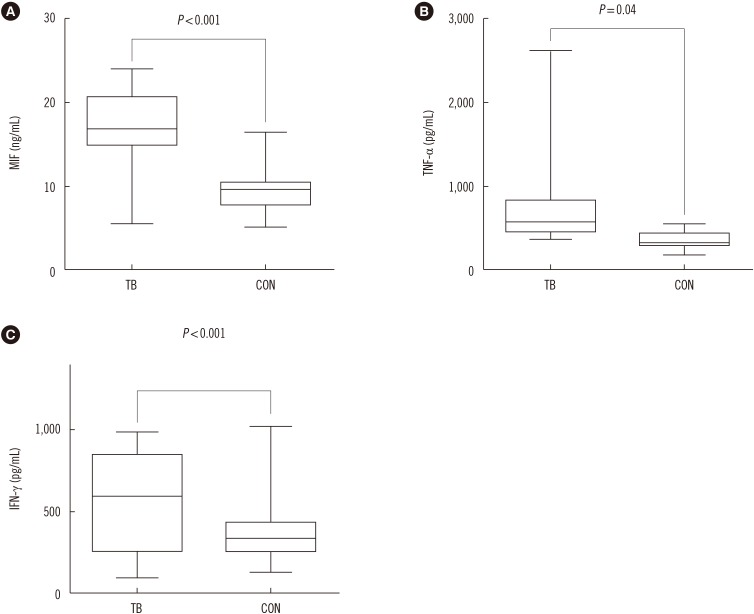
Fig. 2
Serum levels of inflammatory cytokines (MIF, TNF-α, and IFN-γ) in active pulmonary tuberculosis (APTB). Patients with APTB (n= 47) at baseline and following 2, 4, and 6 months of anti-tuberculosis drug (ATD) treatment are indicated with solid lines. Serum levels of inflammatory cytokines in healthy controls at baseline (n=50) are shown with dotted lines. (A) The MIF level in patients with APTB was 17.19 (12.71–21.67) ng/mL at baseline and decreased to 14.57 (11.98–16.98) ng/mL, 9.79 (8.17–12.35) ng/mL, and 7.84 (6.34–8.70) ng/mL following 2, 4, and 6 months of ATD therapy, respectively. (B) The TNF-α level in patients with APTB was 583.6 (453.1–844.5) pg/mL at baseline and decreased to 481.6 (398.7–504.0) pg/mL, 415.5 (346.7–454.9) pg/mL, and 374.5 (312.0–415.0) pg/mL following 2, 4, and 6 months of ATD therapy, respectively. (C) The IFN-γ level in patients with APTB was 559.5 (255.0–864.0) pg/mL at baseline and decreased to 535.2 (341.2–794.0) pg/mL, 465.7 (258.3–649.9) pg/mL, and 401.2 (227.2–574.6) pg/mL following 2, 4, and 6 months of ATD therapy, respectively. Data are presented as median values (interquartile range); error bars indicate standard error of means.
Abbreviation: MIF, macrophage migration inhibitory factor.

Fig. 3
Relationship between serum MIF levels and TNF-α and IFN-γ levels. Levels at baseline (A) and following 2 months (B), 4 months (C), and 6 months (D) of ATD therapy. Only the inflammatory cytokines MIF and TNF-α exhibited a statistically significant (P<0.05) Spearman correlation coefficient value in patients with APTB at baseline and following 6 months of ATD treatment (A and D).
Abbreviation: MIF, macrophage migration inhibitory factor.
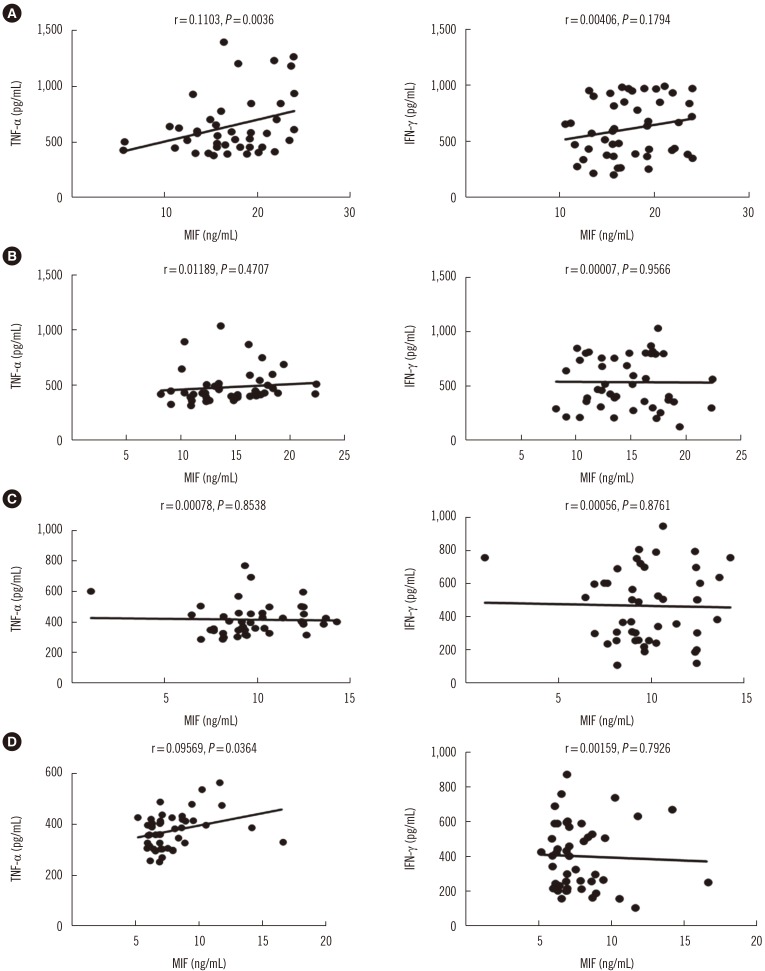
Table 1
Clinical features of patients with tuberculosis and healthy controls
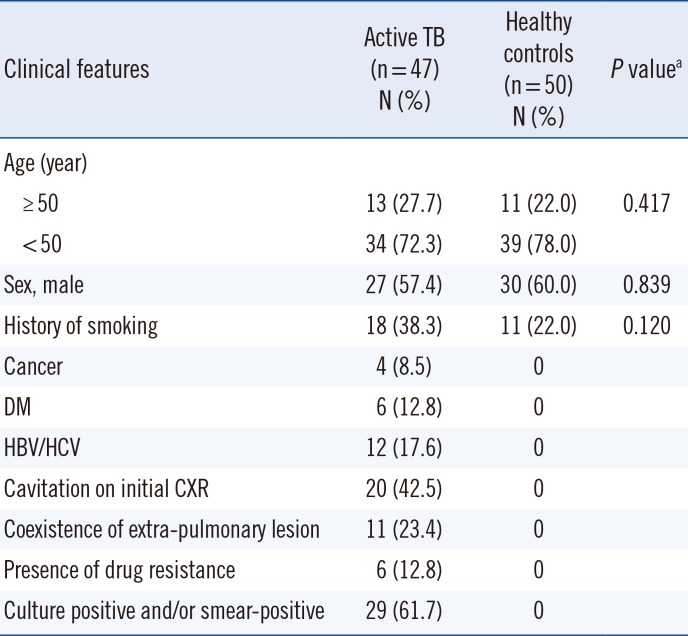
Table 2
Odds ratio (OR) for the associations between potential risk factors and high MIF level (≥13.1 ng/mL)
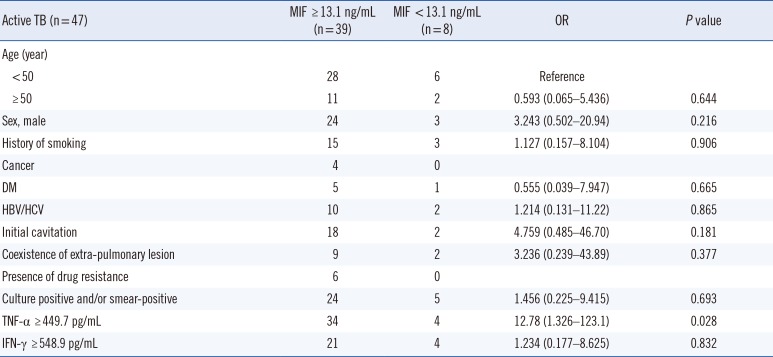