Abstract
Long QT syndrome (LQTS) is an inherited cardiac disease characterized by a prolonged heart rate-corrected QT (QTc) interval. We investigated the genetic causes in patients with prolonged QTc intervals who were negative for pathogenic variants in three major LQTS-related genes (KCNQ1, KCNH2, and SCN5A). Molecular genetic testing was performed using a panel including 13 LQTS-related genes and 67 additional genes implicated in other cardiac diseases. Overall, putative genetic causes of prolonged QTc interval were identified in three of the 30 patients (10%). Among the LQTS-related genes, we detected a previously reported pathogenic variant, CACNA1C c.1552C>T, responsible for cardiac-only Timothy syndrome. Among the genes related to other cardiac diseases, a likely pathogenic variant, RYR2 c.11995A>G, was identified in a patient with catecholaminergic polymorphic ventricular tachycardia. Another patient who developed dilated cardiomyopathy with prolonged QTc interval was found to carry a likely pathogenic variant, TAZ c.718G>A, associated with infantile dilated cardiomyopathy. Comprehensive screening of genetic variants using multigene panel sequencing enables detection of genetic variants with a possible involvement in QTc interval prolongation, thus uncovering unknown molecular mechanisms underlying LQTS.
Long QT syndrome (LQTS) is an inherited cardiac disease characterized by a prolonged heart rate-corrected QT (QTc) interval on the electrocardiogram (ECG). Besides genetic factors, several other conditions can lengthen the QTc interval such as electrolyte imbalance, use of QTc-prolonging drugs, and structural heart diseases.
To date, 15 genes (KCNQ1, KCNH2, SCN5A, ANK2, KCNE1, KCNE2, KCNJ2, CACNA1C, CAV3, SCN4B, AKAP9, SNTA1, KCNJ5, CALM1, and CALM2) have been implicated in LQTS. KCNQ1, KCNH2, and SCN5A are known to be responsible for 60–75% of genotype-positive LQTS cases [12], while the other 12 genes linked to LQTS susceptibility collectively account for <5%. Because genetic predisposition often factors in cases of acquired LQTS, genetic profiling is important, even for patients with mild QTc interval prolongation [3].
Here, we applied targeted sequencing based on a multigene panel containing known LQTS-associated genes to investigate the genetic background of patients with prolonged QTc interval, but negative for mutations in KCNQ1, KCNH2, and SCN5A by Sanger sequencing. The multigene panel also included 67 genes related to other cardiac diseases, which were used to target patients without pathogenic variants of the LQTS-associated genes. For this study, 30 participants were selected among patients with prolonged QTc interval who underwent genetic testing at the Seoul National University Hospital, Korea, between 2005 and 2011. DNA samples had been archived after the routing screening of KCNQ1, KCNH2, and SCN5A variants for the purpose of this research. Most participants exhibited prolonged QTc interval on the resting ECG (>450 ms) and/or positive results on the provocative tests; a few patients with borderline QTc intervals, but suspected LQTS, were also included. The study was approved by the institutional review board of Seoul National University Hospital.
The multigene test panel included 13 known LQTS-associated genes (AKAP9, ANK2, CACNA1C, CAV3, KCNE1, KCNE2, KCNH2, KCNJ2, KCNJ5, KCNQ1, SCN4B, SCN5A, and SNTA1) and 67 genes related to other cardiac diseases (see Supplemental Table S1). DNA samples were enriched using the TruSeq Custom Enrichment Kit and sequenced with MiSeq (Illumina, Inc., San Diego, CA, USA); sequencing data were analyzed using the NextGENe® Software (Softgenetics, State College, PA, USA). Pathogenic/likely pathogenic variants or variants of uncertain significance were further confirmed by Sanger sequencing. Copy number variation was not analyzed. Exonic variants with non-synonymous changes and intronic variants in 10-bp exon-flanking regions were analyzed. Each variant was assessed by considering allele frequencies in normal controls from the 1,000 Genomes database, Exome Aggregation Consortium (ExAC), and Korean Reference Genome DB (KRGDB) and in silico prediction results (Align GVGD, SIFT, and MutationTaster). The highest minor allele frequency (MAF) reported in the databases for any population was taken into account, and variants with MAF >0.1% were filtered out. Each retained variant was classified according to the American College of Medical Genetics (ACMG) guidelines [4]. For previously reported variants, segregation and functional test results were reviewed. When no pathogenic or likely pathogenic variants were found among the LQTS-linked genes, the genes related to other cardiac diseases were sequentially analyzed.
The average coverage depth in target regions of the multigene panel was 235×, representing 1,831 exons in total; 99.77% and 99.95 % of the bases had ≥30× and ≥5× coverage, respectively, the latter was the minimal level of acceptable coverage considered in this study. Regions with coverage <5× were not subjected to Sanger sequencing. The median patient age at examination was 10 years (1 month–30 years), and the average QTc interval was 485 ms (468–502 ms; 95% confidence interval). Of the study participants, only one (patient No. 8) was confirmed to have a pathogenic variant of an LQTS-related gene, CACNA1C c.1552C>T (p.Arg518Cys), which was reported to be associated with Timothy syndrome (Table 1) [5]. This patient showed a phenotype similar to that of an originally reported proband: he had a ventricular septal defect (VSD) detected at birth and QTc interval prolongation of 480 ms accompanied by notched T-wave detected on his ECG at age five. The patient did not have any other extracardiac symptoms frequently found in Timothy syndrome, which could have been caused by the pathogenic variant in the CACNA1C gene. He had a family history of sudden cardiac death, but none of the other family members were tested (Table 2).
Next, genes related to other cardiac diseases were examined in the rest of the patients. One patient (No. 7), who had baseline QTc interval prolongation of 500 ms, exhibited polymorphic ventricular tachyarrhythmia, suggesting a diagnosis of catecholaminergic polymorphic ventricular tachycardia (CPVT). He was found to carry the ANK2 c.10322G>A (p.Arg3441Gln) variant of uncertain significance; however, an examination of the CPVT-related genes revealed that he also harbored a likely pathogenic variant, RYR2 c.11995A>G, (p.Met3999Val), which could have caused CPVT.
Patient No. 13, who developed dilated cardiomyopathy with QTc interval prolongation of 520 ms, carried a likely pathogenic variant, TAZ c.718G>A (p.Gly240Arg), previously reported as a causative mutation in infantile dilated cardiomyopathy [6]. The patient's deceased brother had been similarly diagnosed.
Prior to this study, we tested 57 patients with prolonged QTc interval for pathogenic point mutations and large deletions/duplications in KCNQ1, KCNH2, and SCN5A. Twenty-six patients (45.6%) were identified with pathogenic variants associated with QTc interval prolongation: 14 carried pathogenic mutations in KCNQ1 (24.6%), six in KCNH2 (10.5%), and six in KCNH2 (10.5%) (unpublished data). The detected proportion of pathogenic variants (45.6%) was relatively low compared with that in a previous study demonstrating that pathogenic variants in KCNQ1, KCNH2, and SCN5A accounted for nearly 75% of clinically defined LQTS cases and up to 80%, if copy number variant or genomic rearrangement data were included [7]. Another study tested a panel of 12 LQTS-related genes and reported a molecular diagnostic level of 30%, which was probably due to the inclusion of patients with borderline QTc intervals without other clinical symptoms [8]. More stringent criteria considering longer QTc intervals would increase the detection rate; however, in clinical laboratory settings, physicians may request diagnostic testing for patients with prolonged QTc interval and an intermediate probability of LQTS. Therefore, mutation analysis of genes related to a broader spectrum of cardiac diseases that can cause QTc interval prolongation should improve the diagnostic rate.
In this study, we detected pathogenic or likely pathogenic variants relevant to QTc interval prolongation in three out of 30 patients (10%) negative for KCNQ1, KCNH2, and SCN5A pathogenic variants. Only one patient was confirmed to have a pathogenic variant in an LQTS-related gene. When we expanded the targets to other cardiac disease-related genes, we detected likely pathogenic variants in two patients who showed cardiac manifestations other than prolonged QTc interval. Similar to the patient No. 7, who carried RYR2 c.11995A>G, several other cases with RYR2 mutations suspected of LQTS were diagnosed as CPVT in a previous study [9]. It should be noted that RYR2 has been proposed as a candidate gene involved in LQTS pathogenesis as it exhibits interactions with several genes with an established role in LQTS pathogenesis [1011].
In addition, the SLMAP c.805G>A variant, originally reported in a Japanese patient with Brugada syndrome [12], was detected in one of our participants who exhibited an ECG with a complete AV block, but did not exhibit Brugada syndrome features. A previous functional analysis study showed that SLMAP c.805G>A affects the membrane expression of cardiac sodium channel hNav1.5 and reduces hNav1.5-dependent current [12]. However, we found that this variant had a MAF of 0.16% by KRGDB, which was higher than expected for the disorder; therefore, it was recategorized as a variant of uncertain significance.
Because multigene panel sequencing has become widely available, mutation screening of cardiac disease-related genes that may cause QTc interval prolongation can be conducted in parallel, providing more comprehensive molecular analysis and improving the diagnosis rate in patients negative for mutations in LQTS-related genes. Incidental findings of well-described genes included in the expanded gene panel should be taken into consideration, especially if they are present in the minimum list recommended by the ACMG [13].
In conclusion, the multigene panel sequencing performed in this study enabled comprehensive screening of genetic variants with possible involvement in prolonged QTc interval and helped identify additional patients with genotypes that may lead to QTc interval prolongation. Thus, this approach expands the spectrum of genetic causes underlying QTc interval prolongation and can help prevent adverse outcomes, such as sudden cardiac death, in patients and their relatives [14].
References
1. Alders M, Christiaans I. Long QT Syndrome. In : Pagon RA, Adam MP, editors. GeneReviews(R). Seattle (WA): niversity of Washington;1993. p. 1993–2017.
2. Giudicessi JR, Ackerman MJ. Genotype- and phenotype-guided management of congenital long QT syndrome. Curr Probl Cardiol. 2013; 38:417–455. PMID: 24093767.
3. Itoh H, Crotti L, Aiba T, Spazzolini C, Denjoy I, Fressart V, et al. The genetics underlying acquired long QT syndrome: impact for genetic screening. Eur Heart J. 2016; 37:1456–1464. PMID: 26715165.
4. Richards S, Aziz N, Bale S, Bick D, Das S, Gastier-Foster J, et al. Standards and guidelines for the interpretation of sequence variants: a joint consensus recommendation of the American College of Medical Genetics and Genomics and the Association for Molecular Pathology. Genet Med. 2015; 17:405–424. PMID: 25741868.
5. Boczek NJ, Ye D, Jin F, Tester DJ, Huseby A, Bos JM, et al. Identification and Functional Characterization of a Novel CACNA1C-Mediated Cardiac Disorder Characterized by Prolonged QT Intervals With Hypertrophic Cardiomyopathy, Congenital Heart Defects, and Sudden Cardiac Death. Circ Arrhythm Electrophysiol. 2015; 8:1122–1132. PMID: 26253506.
6. D'Adamo P, Fassone L, Gedeon A, Janssen EA, Bione S, Bolhuis PA, et al. The X-linked gene G4.5 is responsible for different infantile dilated cardiomyopathies. Am J Hum Genet. 1997; 61:862–867. PMID: 9382096.
7. Ackerman MJ, Priori SG, Willems S, Berul C, Brugada R, Calkins H, et al. HRS/EHRA expert consensus statement on the state of genetic testing for the channelopathies and cardiomyopathies: this document was developed as a partnership between the Heart Rhythm Society (HRS) and the European Heart Rhythm Association (EHRA). Europace. 2011; 13:1077–1109. PMID: 21810866.
8. Lieve KV, Williams L, Daly A, Richard G, Bale S, Macaya D, et al. Results of genetic testing in 855 consecutive unrelated patients referred for long QT syndrome in a clinical laboratory. Genet Test Mol Biomarkers. 2013; 17:553–561. PMID: 23631430.
9. Stattin EL, Bostrom IM, Winbo A, Cederquist K, Jonasson J, Jonsson BA, et al. Founder mutations characterise the mutation panorama in 200 Swedish index cases referred for Long QT syndrome genetic testing. BMC Cardiovasc Disord. 2012; 12:95. PMID: 23098067.
10. Kauferstein S, Kiehne N, Erkapic D, Schmidt J, Hamm CW, Bratzke H, et al. A novel mutation in the cardiac ryanodine receptor gene (RyR2) in a patient with an unequivocal LQTS. Int J Cardiol. 2011; 146:249–250. PMID: 21126784.
11. Shigemizu D, Aiba T, Nakagawa H, Ozaki K, Miya F, Satake W, et al. Exome Analyses of Long QT Syndrome Reveal Candidate Pathogenic Mutations in Calmodulin-Interacting Genes. PLoS One. 2015; 10:e0130329. PMID: 26132555.
12. Ishikawa T, Sato A, Marcou CA, Tester DJ, Ackerman MJ, Crotti L, et al. A novel disease gene for Brugada syndrome: sarcolemmal membrane-associated protein gene mutations impair intracellular trafficking of hNav1.5. Circ Arrhythm Electrophysiol. 2012; 5:1098–1107. PMID: 23064965.
13. Kalia SS, Adelman K, Bale SJ, Chung WK, Eng C, Evans JP, et al. Recommendations for reporting of secondary findings in clinical exome and genome sequencing, 2016 update (ACMG SF v2.0): a policy statement of the American College of Medical Genetics and Genomics. Genet Med. 2017; 19:249–255. PMID: 27854360.
14. Nakano Y, Shimizu W. Genetics of long-QT syndrome. J Hum Genet. 2016; 61:51–55. PMID: 26108145.
Table 1
Pathogenic, likely pathogenic variants and variants of uncertain significance detected in this study
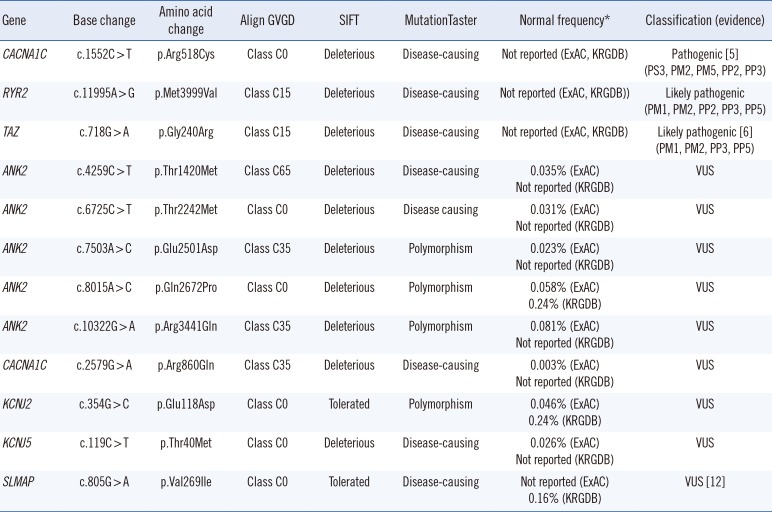
Gene | Base change | Amino acid change | Align GVGD | SIFT | MutationTaster | Normal frequency* | Classification (evidence) |
---|---|---|---|---|---|---|---|
CACNA1C | c.1552C > T | p.Arg518Cys | Class C0 | Deleterious | Disease-causing | Not reported (ExAC, KRGDB) | Pathogenic [5] (PS3, PM2, PM5, PP2, PP3) |
RYR2 | c.11995A > G | p.Met3999Val | Class C15 | Deleterious | Disease-causing | Not reported (ExAC, KRGDB)) | Likely pathogenic (PM1, PM2, PP2, PP3, PP5) |
TAZ | c.718G > A | p.Gly240Arg | Class C15 | Deleterious | Disease-causing | Not reported (ExAC, KRGDB) | Likely pathogenic [6] (PM1, PM2, PP3, PP5) |
ANK2 | c.4259C > T | p.Thr1420Met | Class C65 | Deleterious | Disease-causing |
0.035% (ExAC) Not reported (KRGDB) |
VUS |
ANK2 | c.6725C > T | p.Thr2242Met | Class C0 | Deleterious | Disease causing |
0.031% (ExAC) Not reported (KRGDB) |
VUS |
ANK2 | c.7503A > C | p.Glu2501Asp | Class C35 | Deleterious | Polymorphism |
0.023% (ExAC) Not reported (KRGDB) |
VUS |
ANK2 | c.8015A > C | p.Gln2672Pro | Class C0 | Deleterious | Polymorphism |
0.058% (ExAC) 0.24% (KRGDB) |
VUS |
ANK2 | c.10322G > A | p.Arg3441Gln | Class C35 | Deleterious | Polymorphism |
0.081% (ExAC) Not reported (KRGDB) |
VUS |
CACNA1C | c.2579G > A | p.Arg860Gln | Class C35 | Deleterious | Disease-causing |
0.003% (ExAC) Not reported (KRGDB) |
VUS |
KCNJ2 | c.354G > C | p.Glu118Asp | Class C0 | Tolerated | Polymorphism |
0.046% (ExAC) 0.24% (KRGDB) |
VUS |
KCNJ5 | c.119C > T | p.Thr40Met | Class C0 | Deleterious | Disease-causing |
0.026% (ExAC) Not reported (KRGDB) |
VUS |
SLMAP | c.805G > A | p.Val269Ile | Class C0 | Tolerated | Disease-causing | Not reported (ExAC) 0.16% (KRGDB) | VUS [12] |
Table 2
Clinical characteristics of the patients included in this study
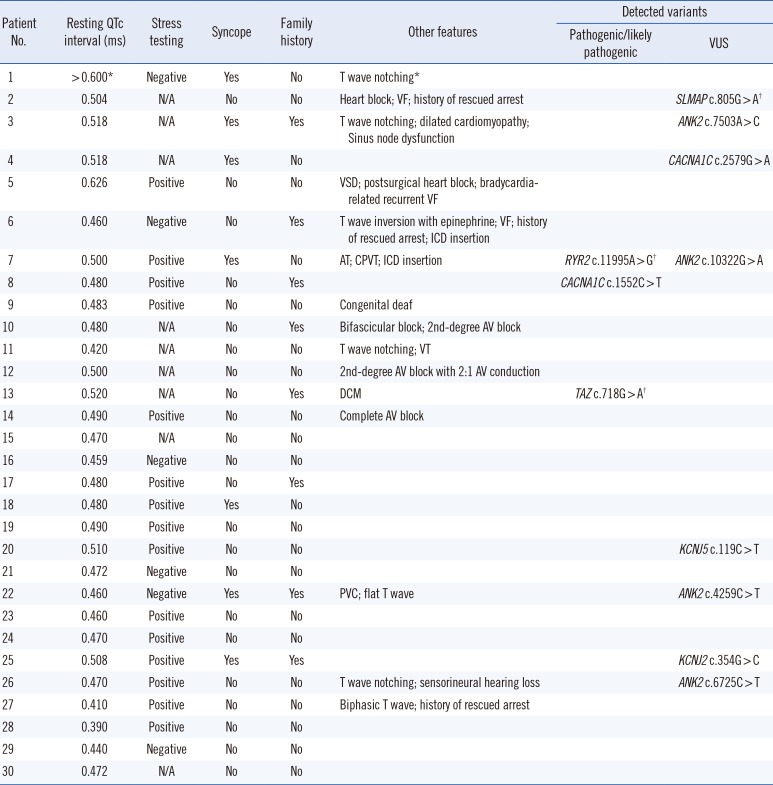
*Prolonged QTc interval > 0.600 ms with T wave notching was described in a medical record transferred from another healthcare center; †Variants detected in the expanded panel of other cardiac disease-related genes.
Abbreviations: AT, atrial tachycardia; AV, atrioventricular; CPVT, catecholaminergic polymorphic ventricular tachycardia; DCM, dilated cardiomyopathy; ICD, implantable cardioverter defibrillator; PVC, premature ventricular contraction; VF, ventricular fibrillation; VSD, ventricular septal defect; VT, ventricular tachycardia; and VUS, variant of uncertain significance.