Abstract
Measurement of thiopurine metabolites is helpful to monitor adverse effects and assess compliance in patients on thiopurine treatment. The purpose of this study was to develop and validate an analytical method for measurement of thiopurine metabolites, thioguanine nucleotides (6-TGN) and 6-methylmercaptopurine nucleotide (6-MMPN), in RBCs. We developed and validated a liquid chromatography–tandem mass spectrometry (LC-MS/MS) method for the quantification of 6-TGN and 6-MMPN and evaluated the stability of the thiopurine metabolites in RBC and whole blood states without any preprocessing at various storage conditions. The linear range was 0.1–10 µmol/L and 0.5–100 µmol/L for 6-TGN and 6-MMPN, respectively. The mean extraction recovery at the two concentrations was 71.0% and 75.0% for 6-TGN, and 102.2% and 96.4% for 6-MMPN. Thiopurine metabolites in preprocessed RBC samples were stable at 25℃ and 4℃ after storage for 4 hours and at −70℃ for up to 6 months. However, 6-TGN decreased by 30% compared with the initial concentration when stored at −20℃ for 180 days. In whole blood states, 6-TGN decreased by about 20% at four days after storage at 4℃. We validated a reliable LC-MS/MS method and recommend that the patient's whole blood sample be preprocessed as soon as possible.
The thiopurines, 6-mercaptopurine (6-MP), azathioprine (AZA), and 6-thioguanine (6-TG), are immunosuppressive drugs used in the treatment of acute leukemia, inflammatory bowel disease (IBD), and other autoimmune diseases. Thiopurines are prodrugs that require activation by several drug-metabolizing enzymes, including thiopurine S-methyltransferase (TPMT) [12]. 6-Thioguanine nucleotides (6-TGN) are formed from 6-MP by several enzymes, and 6-MP is inactivated by the action of TPMT, producing 6-methylmercaptopurine nucleotides (6-MMPN) [3]. Genetic polymorphisms and activities of enzymes involved in thiopurine metabolic pathways might determine the relative concentrations of 6-TGN and 6-MMPN related to thiopurine efficacy and various adverse effects, respectively [4]. Additionally, the monitoring of thiopurine metabolites can be useful for adjusting thiopurine dosage to improve treatment outcome and avoid adverse effects [3]. Two thiopurine metabolites are currently measured in washed red blood cells (RBCs) as a surrogate to determine the intracellular levels of these metabolites [56]. The therapeutic thresholds associated with high treatment efficacy (6-TGNs>235 pmol/8×108 RBCs), increased risk for leukopenia (6-TGNs>450 pmol/8×108 RBCs), and increased risk for hepatotoxicity (6-MMPNs>5,700 pmol/8×108 RBCs) can be used to determine an optimum dosage of thiopurines [78910].
HPLC and liquid chromatography-tandem mass spectrometry (LC-MS/MS) have been used to measure the concentrations of 6-TGN and 6-MMPN in RBCs [51112]. As the metabolites are unstable and can be altered during sample preparation and storage, control of every pre-analytical step is important to ensure reliable assay results. However, to our knowledge, stability of thiopurine metabolites has rarely been studied [1314]. For the first time in Korea, we developed and validated an LC-MS/MS method for the quantification of 6-TGN and 6-MMPN in RBCs. Additionally, we investigated the stability of thiopurine metabolites under various storage conditions to identify appropriate sample processing and storage conditions.
We prepared stock solutions of 3.0 mM 6-TGN and 30 mM 6-MMPN using standard materials (Sigma-Aldrich, St. Louis, MO, USA) and a stock solution of internal standards (IS) containing 1 mg/mL of each of 6-TG-13C215N and 6-MMP-d3 (Toronto Research Chemicals, Toronto, Canada) in methanol. These solutions were stored at −70℃. We diluted the stock solutions in 50 mM NaOH to prepare calibrators. We prepared the calibrators and control samples using whole blood (matrix-matched) spiked with the prepared drug standard solutions. Then, we validated the accuracy of our method for both metabolites. Six concentrations of calibrators (0.5, 2, 4, 6, 8, 10 µmol/L for 6-TGN and 5, 20, 40, 60, 80, 100 µmol/L for 6-MMPN) and quality-control materials at two concentrations for each analyte (2.5 µmol/L and 8.0 µmol/L for 6-TGN, and 25.0 µmol/L and 80.0 µmol/L for 6-MMPN) were measured during each analysis.
Residual EDTA-treated whole blood samples from patients under thiopurine treatment (N=4) were centrifuged (4,000 rpm, 10 minutes), and the plasma and buffy coat were discarded. This study was approved by the Institutional Review Board of Samsung Medical center, Seoul, Korea (IRB No. SMC-04-106-014). Two washing steps were performed, and the RBC count was determined using an automatic hematological cell counting device, XE-2100 (Sysmex Corp. Kobe, Japan), after diluting 100 µL of RBC three times in 200 µL of saline. The isolated RBCs were stored at −70℃ until further analysis. After thawing the stored samples, 50 µL of each sample was transferred into a tube containing 200 µL of 0.2 M D,L-dithiothreitol (DTT) and 30 µL of working internal standards (0.5 mg/L for 6-MMPN and 0.125 mg/L for 6-TGN). After vortexing twice, the sample was rapidly deproteinized by the addition of 40 µL of 70% perchloric acid (GFS Chemicals, Columbus, Ohio, USA). After vortexing at the highest frequency, the deproteinized samples were centrifuged at 15,000 rpm for 10 minutes. The supernatant (100 µL) was transferred to a new tube that was sealed well and heated for one hour at 100℃ to hydrolyze thiopurine nucleotides into their individual bases. After cooling, a 5-µL sample aliquot in 200 µL of mobile phase A of the chromatograph (water containing 2 mM ammonium acetate and 0.1% formic acid) was injected into the column as described below.
Chemical analyses were performed on an API 4000 tandem mass spectrometer (AB Sciex, Foster City, CA, USA) equipped with an Agilent 1200 HPLC system (Agilent Technologies Inc., Santa Clara, CA, USA) and an electrospray ionization (ESI) source operated in positive-ion detection mode. Chromatographic separation of 6-TGN and 6-MMPN was conducted on an X-bridge Shield RP18 (Imtackt, Portland, OR, USA; 1.7 µm, 2.1×150 mm). The flow rate was 0.5 mL/min. Quantitative analysis was performed in multiple reaction-monitoring mode (m/z 168.0→150.9 for 6-TGN, 158.0→110.0 for 6-MMP, 171.0→154.0 for 6-TG-13C215N, 161.1→110.1 for 6-MMP-d3), with a total running time of 3 minutes for each sample. Quantification was performed using the ratio of the integrated peak areas of 6-TGN and 6-MMPN to that of the IS, and calculations were performed using Analyst MD software (AB Sciex, Foster City, CA, USA) and a calibration curve with a weighting factor of 1/x.
The chromatogram showed no interfering peaks for 6-TGN and 6-MMPN. For the IS, retention times were 0.94 minutes for 6-TG-13C215N and 1.19 minutes for 6-MMP-d3 (Fig. 1). The within-run (N=5) and between-run (N=10) precision and accuracy were determined at different concentrations of 6-TGN and 6-MMPN (2.5 µmol/L and 8.0 µmol/L for 6-TGN and 25.0 µmol/L and 80.0 µmol/L for 6-MMPN). Within- and between-run imprecisions evaluated by using quality control materials demonstrated coefficient of variation (CV) values less than 10% (see Supplemental Data Table S1). The linear assay range was 0.1–10 µmol/L and 0.5–100 µmol/L for 6-TGN and 6-MMPN, respectively (R2>0.999 for both 6-TGN and 6-MMPN). The lower limit of quantitation (LLOQ) was defined as the lowest concentration with a signal to noise ratio of >10% and <20% of the CV. The LLOQ was 0.1 µmol/L for 6-TGN and 0.5 µmol/L for 6-MMPN. Matrix effects were evaluated by comparing the average peak areas of post-extraction blank samples spiked with 6-TGN and 6-MMPN to those of pure standard solutions of the corresponding concentrations. Samples were analyzed in quadruplicate at each concentration. The matrix effect ranged from 67.7% to 70.0% for 6-TGN and from 111.0% to 114.1% for 6-MMPN. The extraction recovery of 6-TGN and 6-MMPN was determined. The mean values of extraction recovery at two concentrations were 71.0% and 75.0% for 6-TGN, and 102.2% and 96.4% for 6-MMPN. Low extraction recovery of 6-TGN (~60%) was reported in a previous study [15].
Freeze-and-thaw stability was tested at two concentrations of 6-TGN and 6-MMPN (2.5 µmol/L and 8.0 µmol/L for 6-TGN and 25.0 µmol/L and 80.0 µmol/L for 6-MMPN) four times repeatedly. The materials were subjected to three freeze-and-thaw cycles (−70℃) with none of the materials showing significant changes in concentration; the decrease in concentration materials was less than 5% for both materials.
Short-term stability was investigated by analyzing two concentrations of each of 6-TGN and 6-MMPN (2.5 µmol/L and 8.0 µmol/L for 6-TGN and 25.0 µmol/L and 80.0 µmol/L for 6-MMPN) stored at 25℃ and 4℃ for 0.5, 1, 2, and 4 hours. There was no significant concentration change in either 6-TGN or 6-MMPN at either temperature after storage for 4 hours (Fig. 2). Long-term stability was investigated by analyzing samples stored at −70℃ and −20℃ until assayed (7, 14, 21, 28, 90, and 180 days). When stored at −70℃ for 180 days, 6-TGN decreased to approximately 5% of the initial concentrations (2.5 µmol/L and 8.0 µmol/L), but when stored at −20℃ for 180 days, 6-TGN decreased by 30% compared with the initial concentrations (2.5 µmol/L and 8.0 µmol/L). 6-MMPN decreased by approximately 10% of the initial concentration after 180 days at both temperatures and was rather stable (Fig. 2). Previously, Pike et al [14] reported that 6-TGN concentration decreased by about 12% after six months of storage at −80℃ and that 6-MMPN showed almost no change in concentration after six months. Therefore, samples for long-term storage should be kept at a temperature lower than −70℃ to prevent loss of 6-TGN.
Because whole blood samples cannot be pretreated immediately in a clinical laboratory, it is necessary to evaluate the stability of the thiopurine metabolites in the whole blood. To this end, we stored pooled patient blood samples (N=4) at room temperature (25℃) in the whole blood state and measured 6-TGN and 6-MMPN concentrations for up to seven days. For each concentration, four repeated measurements were conducted. Compared with the initial concentrations, 6-TGN decreased by more than 20% on day 2, and 6-MMPN decreased more than 40% on day 4 (Fig. 3). Although the degree of degradation is somewhat different, Pike et al [14] also found that the 6-TGN concentration decreased by 2–4% per day at room temperature in the whole blood state. Additionally, they found that the loss of 6-TGN was 1% after one week and 12% after 24 weeks at 80℃, indicating that 6-TGN is reasonably stable in whole blood, and 6-MMPN did not show a significant change when stored in the whole blood state for 24 weeks [14]. 6-TGN and 6-MMPN were relatively stable at 4℃ without pretreatment. However, the 6-TGN concentration decreased by about 20% after four days of storage at 4℃. Therefore, pretreatment should be carried out within four days when samples are stored at 4℃.
In conclusion, we developed and validated a reliable LC-MS/MS method for quantification of RBC 6-TGN and 6-MMPN and comprehensively analyzed their stability. Based on the stability test results, we recommend −70℃ for long-term storage of pretreatment samples. The whole blood sample should be preprocessed as soon as possible.
Acknowledgments
This study was supported by a grant of Korea Health Technology R&D Project through the Korea Health Industry Development Institute (KHIDI), funded by the Ministry of Health and Welfare, Republic of Korea (grant number: HI13C2098) and a grant funded in 2017 by the Korean Foundation for Cancer Research (KFCR-2017-D-1).
References
1. Karran P, Attard N. Thiopurines in current medical practice: molecular mechanisms and contributions to therapy-related cancer. Nat Rev Cancer. 2008; 8:24–36. PMID: 18097462.
2. Sahasranaman S, Howard D, Roy S. Clinical pharmacology and pharmacogenetics of thiopurines. Eur J Clin Pharmacol. 2008; 64:753–767. PMID: 18506437.
3. Gearry RB, Barclay ML. Azathioprine and 6-mercaptopurine pharmacogenetics and metabolite monitoring in inflammatory bowel disease. J Gastroenterol Hepatol. 2005; 20:1149–1157. PMID: 16048561.
4. Lee MN, Kang B, Choi SY, Kim MJ, Woo SY, Kim JW, et al. Impact of genetic polymorphisms on 6-thioguanine nucleotide levels and toxicity in pediatric patients with IBD treated with azathioprine. Inflamm Bowel Dis. 2015; 21:2897–2908. PMID: 26332308.
5. Dervieux T, Boulieu R. Simultaneous determination of 6-thioguanine and methyl 6-mercaptopurine nucleotides of azathioprine in red blood cells by HPLC. Clin Chem. 1998; 44:551–555. PMID: 9510860.
6. Vikingsson S, Almer S, Peterson C, Carlsson B, Josefsson M. Monitoring of thiopurine metabolites – a high-performance liquid chromatography method for clinical use. J Pharm Biomed Anal. 2013; 75:145–152. PMID: 23261807.
7. Nygaard U, Toft N, Schmiegelow K. Methylated metabolites of 6-mercaptopurine are associated with hepatotoxicity. Clin Pharmacol Ther. 2004; 75:274–281. PMID: 15060506.
8. Dubinsky MC, Yang H, Hassard PV, Seidman EG, Kam LY, Abreu MT, et al. 6-MP metabolite profiles provide a biochemical explanation for 6-MP resistance in patients with inflammatory bowel disease. Gastroenterology. 2002; 122:904–915. PMID: 11910342.
9. Cuffari C, Dassopoulos T, Turnbough L, Thompson RE, Bayless TM. Thiopurine methyltransferase activity influences clinical response to azathioprine in inflammatory bowel disease. Clin Gastroenterol Hepatol. 2004; 2:410–417. PMID: 15118980.
10. Dervieux T, Meyer G, Barham R, Matsutani M, Barry M, Boulieu R, et al. Liquid chromatography-tandem mass spectrometry analysis of erythrocyte thiopurine nucleotides and effect of thiopurine methyltransferase gene variants on these metabolites in patients receiving azathioprine/6-mercaptopurine therapy. Clin Chem. 2005; 51:2074–2084. PMID: 16166171.
11. Cangemi G, Barabino A, Barco S, Parodi A, Arrigo S, Melioli G. A validated HPLC method for the monitoring of thiopurine metabolites in whole blood in paediatric patients with inflammatory bowel disease. Int J Immunopathol Pharmacol. 2012; 25:435–444. PMID: 22697075.
12. Neurath MF, Kiesslich R, Teichgraber U, Fischer C, Hofmann U, Eichelbaum M, et al. 6-thioguanosine diphosphate and triphosphate levels in red blood cells and response to azathioprine therapy in Crohn’s disease. Clin Gastroenterol Hepatol. 2005; 3:1007–1014. PMID: 16234047.
13. de Graaf P, de Boer NK, Jharap B, Mulder CJ, van Bodegraven AA, Veldkamp AI. Stability of thiopurine metabolites: a potential analytical bias. Clin Chem. 2008; 54:216–218. PMID: 18160731.
14. Pike MG, Franklin CL, Mays DC, Lipsky JJ, Lowry PW, Sandborn WJ. Improved methods for determining the concentration of 6-thioguanine nucleotides and 6-methylmercaptopurine nucleotides in blood. J Chromatogr B Biomed Sci Appl. 2001; 757:1–9. PMID: 11419732.
15. Kirchherr H, Shipkova M, von Ahsen N. Improved method for therapeutic drug monitoring of 6-thioguanine nucleotides and 6-methylmercaptopurine in whole-blood by LC/MSMS using isotope-labeled internal standards. Ther Drug Monit. 2013; 35:313–321. PMID: 23666567.
SUPPLEMENTARY MATERIAL
Supplemental Data Table S1
Precision and accuracy of the LC-MS/MS method for the measurement of 6-TGN and 6-MMPN
Fig. 1
Representative chromatograms of (A) 6-TGN, (B) 6-TG-13C215N (IS for 6-TG), (C) 6-MMPN, and (D) 6-MMP-d3 (IS for 6-MMP).
Abbreviations: IS, internal standard; MRM, multiple-reaction monitoring; cps, counts per second.
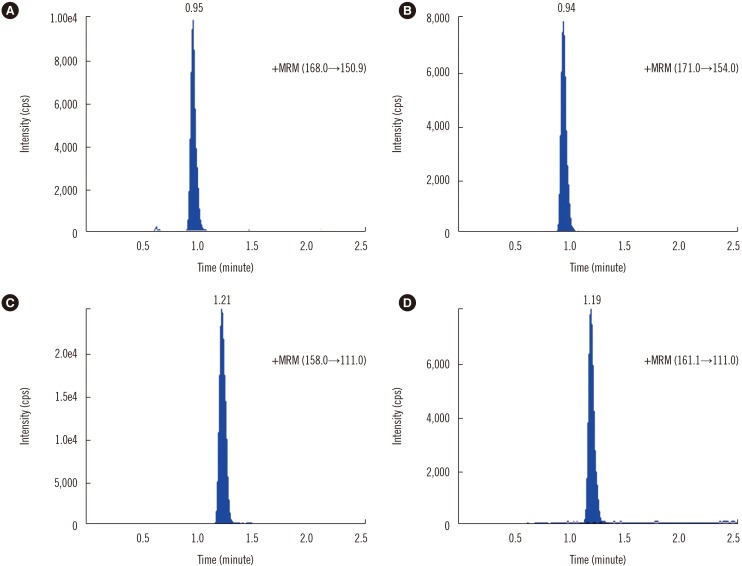