Abstract
Clostridium difficile is a major causative agent of antibiotic-associated diarrhea and has become the most common pathogen of healthcare-associated infection worldwide. The pathogenesis of C. difficile infection (CDI) is mediated by many factors such as colonization involving attachment to host intestinal epithelial cells, sporulation, germination, and toxin production. Bacterial cell surface components are crucial for the interaction between the bacterium and host cells. C. difficile has two distinct surface layer proteins (SLPs): a conserved high-molecular-weight SLP and a highly variable low-molecular-weight SLP. Recent studies have shown that C. difficile SLPs play roles not only in growth and survival, but also in adhesion to host epithelial cells and induction of cytokine production. Sequence typing of the variable region of the slpA gene, which encodes SLPs, is one of the methods currently used for typing C. difficile. SLPs have received much attention in recent years as vaccine candidates and new therapeutic agents in the treatment of C. difficile-associated diseases. Gaining mechanistic insights into the molecular functions of C. difficile SLPs will help advance our understanding of CDI pathogenesis and the development of vaccines and new therapeutic approaches. In this review, we summarize the characteristics and immunological roles of SLPs in C. difficile.
Clostridium difficile was recently shown to be phylogenetically distant from rRNA clostridial cluster I, located in cluster XI, within the family Peptostreptococcaceae [1]. Yutin et al [2] proposed the genus name Peptoclostridium to include all organisms in cluster XI. However, Lawson et al [3] have proposed that Clostridium difficile be named Clostridioides difficile gen. nov. comb. nov. based on phenotypic, chemotaxonomic, and phylogenetic analyses. Thus, although this species may be renamed following proper validation [4], in this review, we used the name Clostridium difficile to ensure visibility and readability, as this is the name conventionally used.
C. difficile is a gram-positive, spore-forming, obligate anaerobic bacterium that is the main causative pathogen of antimicrobial-associated colitis. C. difficile infection (CDI) is the leading cause of infectious diarrhea in hospitalized patients and is increasingly recognized as a common cause of diarrhea in the community [567]. The reported CDI-related mortality rate is 5.7–14%, and the all-cause mortality within 30 days of CDI onset is 11–38% [891011]. Although CDI surveillance in Asia remains limited compared with that in North America and Europe, existing evidence suggests that CDI occurs at similar rates in Asia and other regions in the world [12].
CDI pathogenesis involves the following multiple processes: C. difficile is acquired through ingestion of spores shed into the environment by infected individuals with or without disease symptoms. Once the vegetative cells reach the anaerobic environment of the cecum and colon, they proliferate and colonize the intestinal mucosa. Disruption of the intestinal microbiota, for example by antibiotic treatment, allows the vegetative cells to penetrate the mucus layer and adhere to the surface of the epithelial cells. It is through this intimate contact with host cells that a number of virulence factors produced by C. difficile promote intestinal damage and disease [13].
Many prokaryotes express a surface-exposed proteinaceous layer, termed the surface layer (S-layer), which forms a regular two-dimensional array visible by electron microscopy [14]. S-layers are found on both gram-positive and -negative bacteria and are highly prevalent in archaea; they comprise one or more types of S-layer proteins (SLPs), which are some of the most abundant bacterial cell proteins [15]. Our knowledge of SLPs has increased over the past decade; SLPs play important roles not only in growth and survival but also in the interaction with the host and its immune system. All C. difficile strains also express crystalline or paracrystalline SLPs on the outer cell surface [16]. Recent studies have shown that C. difficile SLPs are involved not only in adhesion to host intestinal cells but also in the induction of cytokine production and the recognition of C. difficile by the immune system [171819]. In this review, we highlight recent discoveries in the field of C. difficile SLPs and discuss their importance in CDI pathogenesis.
Although the S-layer of most bacteria is comprised of one major protein, which is modified by glycosylation in some species [20], C. difficile expresses two distinct SLPs that form two superimposed and structurally different S-layer lattices [142122], which are not glycosylated [23]. Of the two distinct C. difficile SLPs, one is a high-molecular-weight SLP (HMW-SLP; ~40 kDa), and the other is a low-molecular-weight SLP (LMW-SLP; ~35 kDa) (Fig. 1) [24]. Both SLPs are exposed at the cell surface, as determined by surface iodination and immunogold labeling and by indirect immunofluorescence [25]. A single gene, slpA, that has a conserved genomic location among all C. difficile strains, encodes both SLPs. slpA encodes a precursor protein, termed SlpA, that has three identifiable subdomains: an N-terminal secretion signal, followed by a highly variable LMW region, and a HMW region containing three tandem cell wall binding 2 (CWB2) motifs (Fig. 1A) [26]. The precursor protein is then cleaved by protease Cwp84 to generate the two mature proteins (HMW-SLP and LMW-SLP; Fig. 1B) [272829]. Both HMW- and LMW-SLPs are linked by non-covalent interactions to form a tightly associated complex [21]. Although SLPs have been identified in all C. difficile isolates, there is sequence variability between the PCR ribotype strains [3031]. HMW-SLP is highly conserved in C. difficile, with up to 97% sequence identity between the strains [14]; it exhibits strong and specific binding to gastrointestinal tissues and human epithelial cells and is most likely anchored to the cell wall [32]. LMW-SLP, in contrast, exhibits greater sequence variation between strains. It is not known how the sequence differences in the LMW region influence SLP-host interaction. The mechanism by which the HMW/LMW-SLP complex assembles to form the mature S-layer remain unknown.
The C. difficile genome encodes 29 SlpA paralogues that comprise the cell wall protein family. The slpA gene is located within a 36.6 kb cell wall protein (cwp) gene cluster; slpA is encoded within a genomic locus including 11 of these 29 paralogues [2227]. A previous study using whole-genome sequencing identified a 10-kb cassette within the S-layer locus, including the slpA, secA2, cep2, and cwp66 genes, that displays higher inter-strain diversity than the rest of the locus [33]. This suggests that frequent and independent horizontal transfer of the cwp cluster has occurred throughout the C. difficile population.
To initiate the C. difficile colonization process in the host, C. difficile must first adhere to the intestinal cells. Multiple adhesins have been implicated in the attachment of C. difficile to the mucus layer of the intestine: the flagellar cap protein, FliD; the flagellin FliC; the surface-associated heat-shock-induced adhesin, Cwp66; heat-shock protein, GroEL; fibronectin-binding proteins; and binary toxin [343536373839]. Of these, SLPs constitute a major contributor to bacterial adherence [40]. A study investigating animal and human C. difficile isolates showed that variation in the S-layer led to variable adherence to epithelial cells [41]. As demonstrated by in vitro studies, SLPs bind to Hep-2 cells, Vero cells, and human gastrointestinal tissues [3242]; furthermore, chemical removal of the SLPs or treatment of C. difficile bacterial cells with anti-SLP Fab fragments abolishes C. difficile adherence to mouse 929 and human HeLa cells [25]. Similarly, purified SLPs bind to intestinal tissues and several proteins of the extracellular matrix; antibodies against HMW-SLP inhibit this adherence [32]. Merrigan et al [40] have demonstrated that pre-treatment of host cells with purified SlpA or SlpA subunits abrogates C. difficile-attachment in a dose-dependent manner in vitro. Conversely, pre-treatment of viable C. difficile with anti-SlpA antibodies also abrogates adherence. Collectively, these observations suggest that C. difficile SLPs may contribute to colonization and infection persistence. However, the precise host receptor that interacts with the SLPs has yet to be identified.
SLPs have emerged as a second class of C. difficile virulence determinants, in addition to the large clostridial toxins (toxin A and toxin B) [42]. Recent studies have begun to characterize host innate and adaptive responses to C. difficile attachment (Fig. 2) [13]. SLPs have the ability to activate pro-inflammatory signaling through toll-like receptor 4 (TLR4) expressed on the surface of host cells [17]. Engagement of TLR4 results in the subsequent production of inflammatory cytokines by initiating downstream signaling of nuclear factor-kappa beta and interferon (IFN) regulatory factor 3 [40], leading to immune cell activation. In vitro studies using mouse bone marrow-derived dendritic cells and human monocyte-derived dendritic cells have shown that purified SLPs induce the production of both pro-inflammatory [tumor necrosis factor-alpha (TNF-α), interleukin (IL)-12, IL-23, and IL-1b] and anti-inflammatory (IL-10) cytokines [1718]. Furthermore, SLPs induce the maturation of dendritic cells characterized by the production of IL-12p70, TNF-α, IL-23, and IL-6 and increased expression of major histocompatibility complex (MHC) class II, cluster of differentiation (CD) 40, CD80, and CD86 [17]. SLP-activated dendritic cells, in turn, drive strong T helper (Th) 1 and Th17 responses characterized by the production of IFN-gamma and IL-17 [17]. Moreover, SLPs can also activate a clearance response in macrophages. SLPs activate macrophages to produce IL-12p40, TNF-α, monocyte chemotactic and activating factor (MCP), and macrophage inflammatory protein 1 (MIP-1); upregulate the expression of cell-surface markers (TLR2, CD40, CD14, and MHC class II); and enhance macrophage phagocytosis and migration [19]. As monocytes and dendritic cells play important roles in the immune response, these observations suggest that SLPs are strongly associated with the activation of host innate and adaptive immune defenses in response to CDI.
CDI due to hypervirulent strains, such as PCR ribotypes 027 and 078, causes severe and recurrent diseases [4344]. There have been conflicting reports regarding the predictability of infection severity based on C. difficile ribotypes [45]. A previous study has demonstrated that there is no difference in the immunomodulation of monocytes and monocyte-derived dendritic cells by SLPs between the hypervirulent (epidemic) or non-hypervirulent (non-epidemic) C. difficile strains [18]. However, a recent study has shown that sequence differences in C. difficile SLPs are tightly linked to disease susceptibility and severity [46]. SLPs from PCR ribotypes 027 and 078, which are hypervirulent strains, induce a more potent inflammatory response, exhibiting up to two-fold increases in IL-6, IL-12p40, and IL10 production; higher levels of CD80, CD40, and MHC class II; and increased chemokine production, relative to the SLPs from PCR ribotypes 001 and 014 [46].
Several typing systems are currently being utilized in epidemiological studies of C. difficile: restriction endonuclease analysis (REA), pulse-field gel electrophoresis (PFGE), PCR ribotyping, multiple locus sequence typing (MLST), and multilocus variable number tandem repeat analysis (MVLA) [47]. Focusing on the diversity of LMW-SLP among C. difficile strains, Karjalainen et al [48] investigated whether slpA genotyping could be used as an alternative to serotyping. They showed that the DNA and deduced amino acid sequences of the slpA variable region were 100% identical within a given serogroup, as confirmed by PCR-restriction fragment length polymorphism (RFLP) analysis and nucleotide sequencing, whereas the inter-serogroup identity was fairly low [48]. Kato et al [31] demonstrated that this typing method has reliable typability and discriminatory power in comparison with PCR ribotyping and applied it to strains isolated directly from stool specimens. Subsequent studies have evaluated this typing method and applied it to the analysis of various local outbreaks of C. difficile, especially in Japan and Germany [314849505152535455565758596061]. slpA typing is reproducible, and the results are easily disseminated. This methodology is relatively low-cost; the overall cost for generating one sequence is estimated to be less than 10 US dollars [48]. Therefore, slpA typing may be a useful method for outbreaks occurring in a hospital setting. However, a comparative study of seven C. difficile typing techniques showed that MLVA exhibits a significantly higher discriminatory power than slpA genotyping, PFGE, or PCR ribotyping [62]. The study performed by Huber et al [63], which included a large number of genomes, demonstrated that isolates of the same ribotype or MLST type may differ in slpA type. For these reasons, slpA genotyping has not become the global standard for C. difficile typing.
While SLPs have limited utility in C. difficile typing, active or passive immunization against SLPs might constitute a viable therapeutic strategy in the treatment of C. difficile-associated disease. Patients with recurrent CDI episodes have significantly lower anti-SLP IgM levels than patients with a single CDI episode [64]. Sera from CDI patients have been shown to have significantly higher anti-SLP IgG levels than sera from carriers or healthy controls, indicating that SLPs are targeted by the host immune responses [65]. Interestingly, the binding of recombinant antibodies to SLPs and their components exhibited strain specificity [66]. These reagents could potentially be useful for diagnosis. Passive immunization using anti-SLP antibodies significantly delays the progression of C. difficile-associated diarrhea and prolongs survival in C. difficile-infected hamsters [67]. SLPs have also been tested as vaccine components in hamsters, but did not fully protect the animals, and antibody production varied from modest to poor [68]. However, intra-rectal vaccination of mice using the recombinant SlpA protein resulted in significantly lower gut colonization by C. difficile in vaccinated mice than in the control group; vaccinated mice also had a higher level of SlpA-specific IgA in their feces than the control mice [69]. Although SlpA is being examined as a vaccine candidate, problems may still arise because of the high slpA sequence variability between strains.
As the use of conventional antibiotics is associated with the risk of developing antibiotic resistance, targeting essential bacterial virulence factors, such as SLPs, provides an alternative antimicrobial therapeutic strategy. Interestingly, a recent study has examined the potential of single-domain antibodies against specific targets as a strategy for enhancing immunity and reducing infection [70]. SLP-specific single-domain antibodies (VHHs) bind the LMW-SLP subunit of the C. difficile hypervirulent-strain with high affinity. Furthermore, VHHs inhibit the motility of the hypervirulent strain in vitro [29]. In contrast to conventional antibodies that are typically comprised of light and heavy chains, single-domain antibodies isolated from the variable domains of Camelidae species contain only the heavy chain. Single-domain antibodies have the advantage of being highly specific, robust, easy to produce, and flexible [71]. Thus, targeting SLPs with single-domain antibodies might have a potential therapeutic application.
Although the role of C. difficile toxins in CDI pathogenesis has been the subject of worldwide investigation for many decades, the role of SLPs in CDI pathogenesis and the mechanisms of attachment to host cells remain largely unexplored. Mechanistic insights into the molecular functions of C. difficile SLPs will further our understanding of CDI pathogenicity and aid in the development of vaccines and novel therapeutic approaches for the treatment of C. difficile-associated disease.
Acknowledgments
We wish to thank Science Graphics Co., Ltd. (www.s-graphics.co.jp) for illustrating Fig. 2 and Editage (www.editage.jp) for English language editing.
References
1. Collins MD, Lawson PA, Willems A, Cordoba JJ, Fernandez-Garayzabal J, Garcia P, et al. The phylogeny of the genus Clostridium: proposal of five new genera and eleven new species combinations. Int J Syst Bacteriol. 1994; 44:812–826. PMID: 7981107.
2. Yutin N, Galperin MY. A genomic update on clostridial phylogeny: Gram-negative spore formers and other misplaced clostridia. Environ Microbiol. 2013; 15:2631–2641. PMID: 23834245.
3. Lawson PA, Citron DM, Tyrrell KL, Finegold SM. Reclassification of Clostridium difficile as Clostridioides difficile (Hall and O'Toole 1935) Prévot 1938. Anaerobe. 2016; 40:95–99. PMID: 27370902.
4. Oren A, Garrity GM. List of new names and new combinations previously effectively, but not validly, published. Int J Syst Evol Microbiol. 2018; 68:1–2. PMID: 29292690.
5. Lessa FC, Mu Y, Bamberg WM, Beldavs ZG, Dumyati GK, Dunn JR, et al. Burden of Clostridium difficile infection in the United States. N Engl J Med. 2015; 372:825–834. PMID: 25714160.
6. Freeman J, Bauer MP, Baines SD, Corver J, Fawley WN, Goorhuis B, et al. The changing epidemiology of Clostridium difficile infections. Clin Microbiol Rev. 2010; 23:529–549. PMID: 20610822.
7. Mori N, Aoki Y. Clinical characteristics and risk factors for community-acquired Clostridium difficile infection: a retrospective, case-control study in a tertiary care hospital in Japan. J Infect Chemother. 2015; 21:864–867. PMID: 26482373.
8. Hensgens MP, Goorhuis A, Dekkers OM, van Benthem BHB, Kuijper EJ. All-cause and disease-specific mortality in hospitalized patients with Clostridium difficile infection: a multicenter cohort study. Clin Infect Dis. 2013; 56:1108–1116. PMID: 23300235.
9. Honda H, Yamazaki A, Sato Y, Dubberke ER. Incidence and mortality associated with Clostridium difficile infection at a Japanese tertiary care center. Anaerobe. 2014; 25:5–10. PMID: 24184291.
10. Takahashi M, Mori N, Bito S. Multi-institution case-control and cohort study of risk factors for the development and mortality of Clostridium difficile infections in Japan. BMJ Open. 2014; 4:e005665.
11. Bloomfield MG, Sherwin JC, Gkrania-Klotsas E. Risk factors for mortality in Clostridium difficile infection in the general hospital population: a systematic review. J Hosp Infect. 2012; 82:1–12. PMID: 22727824.
12. Collins DA, Hawkey PM, Riley TV. Epidemiology of Clostridium difficile infection in Asia. Antimicrob Resist Infect Control. 2013; 2:21. PMID: 23816346.
13. Peniche AG, Savidge TC, Dann SM. Recent insights into Clostridium difficile pathogenesis. Curr Opin Infect Dis. 2013; 26:447–451. PMID: 23982235.
14. Calabi E, Ward S, Wren B, Paxton T, Panico M, Morris H, et al. Molecular characterization of the surface layer proteins from Clostridium difficile. Mol Microbiol. 2001; 40:1187–1199. PMID: 11401722.
15. Fagan RP, Fairweather NF. Biogenesis and functions of bacterial Slayers. Nat Rev Microbiol. 2014; 12:211–222. PMID: 24509785.
16. Masuda K, Itoh M, Kawata T. Characterization and reassembly of a regular array in the cell wall of Clostridium difficile GAI 4131. Microbiol Immunol. 1989; 33:287–298. PMID: 2770560.
17. Ryan A, Lynch M, Smith SM, Amu S, Nel HJ, McCoy CE, et al. A role for TLR4 in Clostridium difficile infection and the recognition of surface layer proteins. PLoS Pathog. 2011; 7:e1002076. PMID: 21738466.
18. Bianco M, Fedele G, Quattrini A, Spigaglia P, Barbanti F, Mastrantonio P, et al. Immunomodulatory activities of surface-layer proteins obtained from epidemic and hypervirulent Clostridium difficile strains. J Med Microbiol. 2011; 60:1162–1167. PMID: 21349985.
19. Collins LE, Lynch M, Marszalowska I, Kristek M, Rochfort K, O'Connell M, et al. Surface layer proteins isolated from Clostridium difficile induce clearance responses in macrophages. Microbes Infect. 2014; 16:391–400. PMID: 24560642.
20. Sára M, Sleytr UB. S-layer proteins. J Bacteriol. 2000; 182:859–868. PMID: 10648507.
21. Fagan RP, Albesa-Jové D, Qazi O, Svergun DI, Brown KA, Fairweather NF. Structural insights into the molecular organization of the S-layer from Clostridium difficile. Mol Microbiol. 2009; 71:1308–1322. PMID: 19183279.
22. Kirk JA, Banerji O, Fagan RP. Characteristics of the Clostridium difficile cell envelope and its importance in therapeutics. Microb Biotechnol. 2017; 10:76–90. PMID: 27311697.
23. Qazi O, Hitchen P, Tissot B, Panico M, Morris HR, Dell A, et al. Mass spectrometric analysis of the S-layer proteins from Clostridium difficile demonstrates the absence of glycosylation. J Mass Spectrom. 2009; 44:368–374. PMID: 18932172.
24. Sarker MR, Paredes-Sabja D. Molecular basis of early stages of Clostridium difficile infection: germination and colonization. Future Microbiol. 2012; 7:933–943. PMID: 22913353.
25. Takeoka A, Takumi K, Koga T, Kawata T. Purification and characterization of S layer proteins from Clostridium difficile GAI 0714. J Gen Microbiol. 1991; 137:261–267. PMID: 1901902.
26. Fagan RP, Fairweather NF. Clostridium difficile has two parallel and essential Sec secretion systems. J Biol Chem. 2011; 286:27483–27493. PMID: 21659510.
27. Fagan RP, Janoir C, Collignon A, Mastrantonio P, Poxton IR, Fairweather NF. A proposed nomenclature for cell wall proteins of Clostridium difficile. J Med Microbiol. 2011; 60:1225–1228. PMID: 21252271.
28. de la Riva L, Willing SE, Tate EW, Fairweather NF. Roles of cysteine proteases Cwp84 and Cwp13 in biogenesis of the cell wall of Clostridium difficile. J Bacteriol. 2011; 193:3276–3285. PMID: 21531808.
29. Kandalaft H, Hussack G, Aubry A, van Faassen H, Guan Y, Arbabi-Ghahroudi M, et al. Targeting surface-layer proteins with single-domain antibodies: a potential therapeutic approach against Clostridium difficile-associated disease. Appl Microbiol Biotechnol. 2015; 99:8549–8562. PMID: 25936376.
30. Karjalainen T, Waligora-Dupriet AJ, Cerquetti M, Spigaglia P, Maggioni A, Mauri P, et al. Molecular and genomic analysis of genes encoding surface-anchored proteins from Clostridium difficile. Infect Immun. 2001; 69:3442–3446. PMID: 11292772.
31. Kato H, Yokoyama T, Arakawa Y. Typing by sequencing the slpA gene of Clostridium difficile strains causing multiple outbreaks in Japan. J Med Microbiol. 2005; 54:167–171. PMID: 15673512.
32. Calabi E, Calabi F, Phillips AD, Fairweather NF. Binding of Clostridium difficile surface layer proteins to gastrointestinal tissues. Infect Immun. 2002; 70:5770–5778. PMID: 12228307.
33. Dingle KE, Didelot X, Ansari MA, Eyre DW, Vaughan A, Griffiths D, et al. Recombinational switching of the Clostridium difficile S-layer and a novel glycosylation gene cluster revealed by large-scale whole-genome sequencing. J Infect Dis. 2013; 207:675–686. PMID: 23204167.
34. Tasteyre A, Barc MC, Collignon A, Boureau H, Karjalainen T. Role of FliC and FliD flagellar proteins of Clostridium difficile in adherence and gut colonization. Infect Immun. 2001; 69:7937–7940. PMID: 11705981.
35. Ternan NG, Jain S, Srivastava M, McMullan G. Comparative transcriptional analysis of clinically relevant heat stress response in Clostridium difficile strain 630. PLoS One. 2012; 7:e42410. PMID: 22860125.
36. Waligora AJ, Hennequin C, Mullany P, Bourlioux P, Collignon A, Karjalainen T. Characterization of a cell surface protein of Clostridium difficile with adhesive properties. Infect Immun. 2001; 69:2144–2153. PMID: 11254569.
37. Janoir C, Barc MC, Collignon A, Karjalainen T. Identification and characterization of a fibronectin-binding protein from Clostridium difficile. Microbiology. 2003; 149:2779–2787. PMID: 14523111.
38. Cerquetti M, Molinari A, Sebastianelli A, Diociaiuti M, Petruzzelli R, Capo C, et al. Characterization of surface layer proteins from different Clostridium difficile clinical isolates. Microb Pathog. 2000; 28:363–372. PMID: 10839973.
39. Gerding DN, Johnson S, Rupnik M, Aktories K. Clostridium difficile binary toxin CDT. Gut Microbes. 2014; 5:15–27. PMID: 24253566.
40. Merrigan MM, Venugopal A, Roxas JL, Anwar F, Mallozzi MJ, Roxas BA, et al. Surface-layer protein A (SlpA) is a major contributor to host-cell adherence of Clostridium difficile. PLoS One. 2013; 8:e78404. PMID: 24265687.
41. Spigaglia P, Barketi-Klai A, Collignon A, Mastrantonio P, Barbanti F, Rupnik M, et al. Surface-layer (S-layer) of human and animal Clostridium difficile strains and their behaviour in adherence to epithelial cells and intestinal colonization. J Med Microbiol. 2013; 62:1386–1393. PMID: 23518658.
42. Madan R, Petri WA Jr. Immune responses to Clostridium difficile infection. Trends Mol Med. 2012; 18:658–666. PMID: 23084763.
43. Marsh JW, Arora R, Schlackman JL, Shutt KA, Curry SR, Harrison LH. Association of relapse of Clostridium difficile disease with BI/NAP1/027. J Clin Microbiol. 2012; 50:4078–4082. PMID: 23052318.
44. Goorhuis A, Bakker D, Corver J, Debast SB, Harmanus C, Notermans DW, et al. Emergence of Clostridium difficile infection due to a new hypervirulent strain, polymerase chain reaction ribotype 078. Clin Infect Dis. 2008; 47:1162–1170. PMID: 18808358.
45. Walk ST, Micic D, Jain R, Lo ES, Trivedi I, Liu EW, et al. Clostridium difficile ribotype does not predict severe infection. Clin Infect Dis. 2012; 55:1661–1668. PMID: 22972866.
46. Lynch M, Walsh TA, Marszalowska I, Webb AE, MacAogain M, Rogers TR, et al. Surface layer proteins from virulent Clostridium difficile ribotypes exhibit signatures of positive selection with consequences for innate immune response. BMC Evol Biol. 2017; 17:135. PMID: 28606132.
47. Knight DR, Elliott B, Chang BJ, Perkins TT, Riley TV. Diversity and evolution in the genome of Clostridium difficile. Clin Microbiol Rev. 2015; 28:721–741. PMID: 26085550.
48. Karjalainen T, Saumier N, Barc MC, Delmée M, Collignon A. Clostridium difficile genotyping based on slpA variable region in S-layer gene sequence: an alternative to serotyping. J Clin Microbiol. 2002; 40:2452–2458. PMID: 12089261.
49. McCoubrey J, Starr J, Martin H, Poxton IR. Clostridium difficile in a geriatric unit: a prospective epidemiological study employing a novel S-layer typing method. J Med Microbiol. 2003; 52:573–578. PMID: 12808079.
50. Eidhin DN, Ryan AW, Doyle RM, Walsh JB, Kelleher D. Sequence and phylogenetic analysis of the gene for surface layer protein, slpA, from 14 PCR ribotypes of Clostridium difficile. J Med Microbiol. 2006; 55:69–83. PMID: 16388033.
51. Poilane I, Humeniuk-Ainouz C, Durand I, Janoir C, Cruaud P, Delmée M, et al. Molecular characterization of Clostridium difficile clinical isolates in a geriatric hospital. J Med Microbiol. 2007; 56:386–390. PMID: 17314371.
52. Kato H, Kato H, Nakamura M, Iwashima Y, Nakamura A, Ueda R, et al. Rapid analysis of Clostridium difficile strains recovered from hospitalized patients by using the slpA sequence typing system. J Infect Chemother. 2009; 15:199–202. PMID: 19554407.
53. Joost I, Speck K, Herrmann M, von Müller L. Characterization of Clostridium difficile isolates by slpA and tcdC gene sequencing. Int J Antimicrob Agents. 2009; 33(Suppl 1):S13–S18. PMID: 19303562.
54. Kato H, Kato H, Ito Y, Akahane T, Izumida S, Yokoyama T, et al. Typing of Clostridium difficile isolates endemic in Japan by sequencing of slpA and its application to direct typing. J Med Microbiol. 2010; 59:556–562. PMID: 20133413.
55. Tagashira Y, Kato H, Senoh M, Nakamura A. Two cases of fulminant colitis due to binary toxin-positive Clostridium difficile that are not PCR ribotype 027 or type 078. J Med Microbiol. 2013; 62:1486–1489. PMID: 23558137.
56. Xiao K, Kong F, Wang Q, Jin P, Thomas L, Xiong L, et al. Multiplex PCR targeting slpA: a rapid screening method to predict common Clostridium difficile ribotypes among fluoroquinolone resistant clinical strains. Pathology. 2013; 45:595–599. PMID: 24018815.
57. Niwa H, Kato H, Hobo S, Kinoshita Y, Ueno T, Katayama Y, et al. Postoperative Clostridium difficile infection with PCR ribotype 078 strain identified at necropsy in five Thoroughbred racehorses. Vet Rec. 2013; 173:607.
58. Stahlmann J, Schönberg M, Herrmann M, von Müller L. Detection of nosocomial Clostridium difficile infections with toxigenic strains despite negative toxin A and B testing on stool samples. Clin Microbiol Infect. 2014; 20:O590–O592. PMID: 24450741.
59. von Müller L, Mock M, Halfmann A, Stahlmann J, Simon A, Herrmann M. Epidemiology of Clostridium difficile in Germany based on a single center long-term surveillance and German-wide genotyping of recent isolates provided to the advisory laboratory for diagnostic reasons. Int J Med Microbiol. 2015; 305:807–813. PMID: 26341328.
60. Cheng JW, Xiao M, Kudinha T, Kong F, Xu ZP, Sun LY, et al. Molecular epidemiology and antimicrobial susceptibility of Clostridium difficile isolates from a university teaching hospital in China. Front Microbiol. 2016; 7:1621. PMID: 27799923.
61. Miller-Roll T, Na’amnih W, Cohen D, Carmeli Y, Adler A. Molecular types and antimicrobial susceptibility patterns of Clostridium difficile isolates in different epidemiological settings in a tertiary care center in Israel. Diagn Microbiol Infect Dis. 2016; 86:450–454. PMID: 27638350.
62. Killgore G, Thompson A, Johnson S, Brazier J, Kuijper E, Pepin J, et al. Comparison of seven techniques for typing international epidemic strains of Clostridium difficile: restriction endonuclease analysis, pulsed-field gel electrophoresis, PCR-ribotyping, multilocus sequence typing, multilocus variable-number tandem-repeat analysis, amplified fragment length polymorphism, and surface layer protein A gene sequence typing. J Clin Microbiol. 2008; 46:431–437. PMID: 18039796.
63. Huber CA, Foster NF, Riley TV, Paterson DL. Challenges for standardization of Clostridium difficile typing methods. J Clin Microbiol. 2013; 51:2810–2814. PMID: 23784128.
64. Drudy D, Calabi E, Kyne L, Sougioultzis S, Kelly E, Fairweather N, et al. Human antibody response to surface layer proteins in Clostridium difficile infection. FEMS Immunol Med Microbiol. 2004; 41:237–242. PMID: 15196573.
65. Sánchez-Hurtado K, Corretge M, Mutlu E, McIlhagger R, Starr JM, Poxton IR. Systemic antibody response to Clostridium difficile in colonized patients with and without symptoms and matched controls. J Med Microbiol. 2008; 57:717–724. PMID: 18480328.
66. Shirvan AN. Isolation of recombinant antibodies directed against surface proteins of Clostridium difficile. Braz J Microbiol. 2016; 47:394–402. PMID: 26991284.
67. O'Brien JB, McCabe MS, Athié-Morales V, McDonald GS, Ní Eidhin DB, Kelleher DP. Passive immunization of hamsters against Clostridium difficile infection using antibodies to surface layer proteins. FEMS Microbiol Lett. 2005; 246:199–205. PMID: 15899406.
68. Ní Eidhin DB, O'Brien JB, McCabe MS, Athié-Morales V, Kelleher DP. Active immunization of hamsters against Clostridium difficile infection using surface-layer protein. FEMS Immunol Med Microbiol. 2008; 52:207–218. PMID: 18093141.
69. Bruxelle JF, Mizrahi A, Hoys S, Collignon A, Janoir C, Péchiné S. Immunogenic properties of the surface layer precursor of Clostridium difficile and vaccination assays in animal models. Anaerobe. 2016; 37:78–84. PMID: 26505926.
70. Virdi V, Coddens A, De Buck S, Millet S, Goddeeris BM, Cox E, et al. Orally fed seeds producing designer IgAs protect weaned piglets against enterotoxigenic Escherichia coli infection. Proc Natl Acad Sci U S A. 2013; 110:11809–11814. PMID: 23801763.
71. Siontorou CG. Nanobodies as novel agents for disease diagnosis and therapy. Int J Nanomedicine. 2013; 8:4215–4227. PMID: 24204148.
Fig. 1
Amino acid sequence and schematic representation of the SlpA precursor protein. (A) Amino acid sequence of the SlpA precursor protein in Clostridium difficile 630 (GenBank: AJP12540.1). Amino acids highlighted in black indicate the signal peptide and those highlighted in gray denote residues involved in the interaction with the HMW-SLP. The amino acids comprising the LMW-SLP are underlined. The black triangle indicates the cleavage site that results in the generation of the two mature SLPs. (B) Schematic representation of the SlpA precursor protein. The black section represents the signal peptide, the light gray section denotes the conserved region within LMW-SLP, and the black triangle indicates the cleavage site of cysteine protease CWP84, which produces mature LMW-SLP and HMW-SLP.
Abbreviations: SLPs, surface layer proteins; HMW, high molecular weight; LMW, low molecular weight; CWP84, cell wall protein 84.
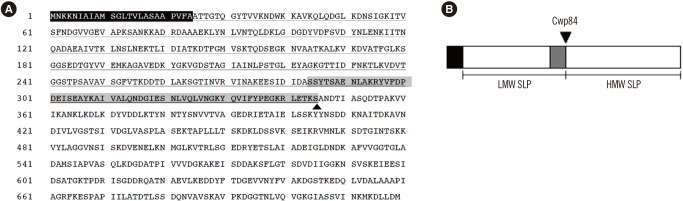
Fig. 2
The SLPs of Clostridium difficile activate dendritic cells and macrophages, which in turn produce various cytokines and chemokines.
Abbreviations: SLPs, surface layer proteins; TLR, toll-like receptor; IL, interleukin; IFN, interferon; MIP, macrophage inflammatory protein; MCP, monocyte chemotactic and activating factor; CD, cluster of differentiation; MHC, major histocompatibility complex; Th, T helper.
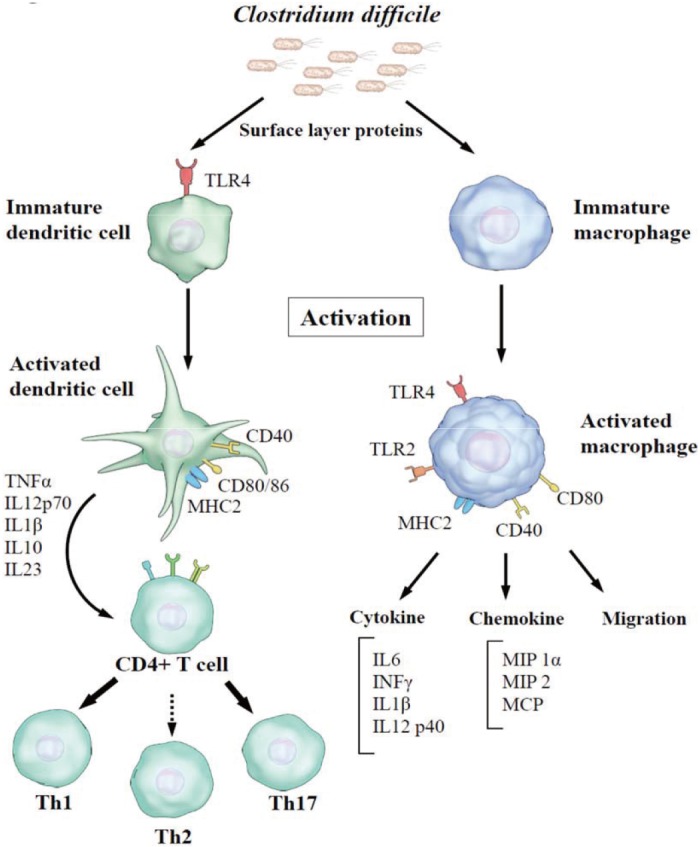