Abstract
Background
JAK2 V617F is the most common mutation in myeloproliferative neoplasms (MPNs) and is a major diagnostic criterion. Mutation quantification is useful for classifying patients with MPN into subgroups and for prognostic prediction. Droplet digital PCR (ddPCR) can provide accurate and reproducible quantitative analysis of DNA. This study was designed to verify the correlation of ddPCR with pyrosequencing results in the diagnosis of MPN and to investigate clinical implications of the mutational burden.
Methods
Peripheral blood or bone marrow samples were obtained from 56 patients newly diagnosed with MPN or previously diagnosed with MPN but not yet indicated for JAK2 inhibitor treatment between 2012 and 2016. The JAK2 V617F mutation was detected by pyrosequencing as a diagnostic work-up. The same samples were used for ddPCR to determine the correlation between assays and establish a detection sensitivity cut-off. Clinical and hematologic aspects were reviewed.
Results
Forty-two (75%) and 46 (82.1%) patients were positive for JAK2 V617F by pyrosequencing and ddPCR, respectively. The mean mutated allele frequency at diagnosis was 37.5±30.1% and was 40.7±31.2% with ddPCR, representing a strong correlation (r=0.9712, P<0.001). Follow-up samples were available for 12 patients, including eight that were JAK2 V617F-positive. Of these, mutational burden reduction after treatment was observed in six patients (75%), consistent with trends of hematologic improvement.
Myeloproliferative neoplasms (MPNs) are chronic neoplastic disorders defined as abnormal increases in mature peripheral blood cells, resulting in the aberrant clonal proliferation of hematopoietic progenitors [1]. With recent advances in the understanding of molecular pathogenesis, identifying genetic mutations has become the most important diagnostic and prognostic factor in hematologic malignancies. Similar to other hematologic disorders, several genetic mutations closely associated with MPNs have been discovered, and their significance in pathogenesis has been recognized. MPNs consist of chronic myeloid leukemia (CML), polycythemia vera (PV), essential thrombocytosis (ET), and myelofibrosis (MF). CML is regarded as having a discriminated clinical severity, with the BCR–ABL rearrangement as a hallmark.
Other BCR–ABL-negative MPNs are also associated with genetic aberrations. Janus kinase 2 (JAK2) is a protein tyrosine kinase gene that is associated with cellular growth and proliferation. The substitution of phenylalanine for valine in the JAK2 protein caused by a point mutation in codon 617 is one of the most frequent genetic mutations found in BCR–ABL-negative MPNs [2]. The JAK2 V617F mutation is detected in nearly 90% of PV patients, and in around a half of all ET and MF patients [34]. Mutations in CALR and MPL are the next most frequent genetic aberrations in MPNs after JAK2 V617F, in that order. Since their identification, a description of the relevant genetic mutations has become essential for diagnosing MPN. Since 2008, the WHO diagnostic criteria include the presence of JAK2, CALR, or MPL mutations among the major diagnostic criteria of MPN [56].
According to the WHO diagnostic criteria, a JAK2 V617F mutation test should be performed routinely as a diagnostic work-up of MPNs. Beyond determining the presence or absence of a mutation, the number of mutations is another important issue in diagnosis and prognosis. Besides the detection of a JAK2 mutation, quantification of a mutated allele burden provides useful information for classifying subgroups of MPNs and to predict disease-associated complications [78]. In previous researches, an increased JAK2 V617F allele burden at diagnosis correlated with a higher chance of venous thromboembolism and disease progression to myelofibrosis or leukemia [891011]. However, few groups have studied how a quantitative change of JAK2 V617F alleles affects the disease course and its potential use as a prognostic marker.
The most suitable method for detecting mutated JAK2 V617F alleles quantitatively remains controversial. The most commonly applied methods are real-time quantitative PCR and pyrosequencing. Such traditional techniques show high sensitivity in detecting mutated alleles. However, data reproducibility could be limited by the quality of samples and less experienced examiners [1213]. Droplet digital PCR (ddPCR) is a novel assay to measure very small amounts of DNA mutations [14]. The subject's DNA is fractionated into tens of thousands of droplets, each bearing a single nucleic acid, which is then amplified by PCR to measure the absolute number of DNA copies [15]. This method has an advantage over traditional techniques in yielding an accurate and reproducible quantitative analysis, even for a small amount of sample. The benefit of ddPCR has been demonstrated for several malignancies [141617].
The aim of this study was to verify the effectiveness of ddPCR in the diagnosis of JAK2 V617F-mutated MPN and to investigate how the mutated allele burden changes during the disease course. The primary objective was to verify a correlation between pyro-sequencing and ddPCR results. Secondary objectives were analysis of the JAK2 V617F mutational burden in patients who were treated for MPN and development of prognostic biomarkers.
Between 2012 and 2016, a total of 56 patients were diagnosed as having MPNs, with no cases of CML, at the National Cancer Center and National Health Insurance Service Ilsan Hospital, Korea, according to the WHO criteria. A JAK2 V617F mutation (GenBank accession number NT_008413.17) was detected by pyrosequencing as a part of the initial diagnostic work-up for MPN. The median age of the patients at diagnosis was 66 years (range, 39–85 years). The proportion of patients with PV was 41.1% (n=23), 35.7% (n=20) were diagnosed as having ET, and 21.4% (n=12) were diagnosed as having MF; 1.8% (n=1) of patients had an unclassifiable MPN but a positive JAK2 V617F mutated status.
Both peripheral blood (n=42) and/or bone marrow (n=14) samples were obtained at diagnosis and every three months after enrollment. The inclusion criteria were as follows: 1) patients older than 20 years, 2) newly diagnosed MPN after beginning the study, and 3) diagnosed as having MPN before the study but not yet meeting the indications for JAK2 inhibitor treatment. Residual clinical samples obtained before the start of the study were included, and post-enrollment follow-up samples after the start of treatment were available for 12 patients. All samples were negative for the BCR–ABL1 rearrangement. The JAK2 V617F mutation was detected by pyrosequencing as a part of the initial diagnostic work-up for MPN. Blood and bone marrow samples were treated by hypotonic lysis to remove contaminating red cells. DNA was extracted from samples using a QIAamp DNA blood mini kit (Qiagen GmbH, Hilden, Germany) and was quantified with a NanoDrop spectrophotometer (NanoDrop Technologies, Wilmington, DE, USA).
This study was approved by the Institutional Review Board (No. NCC2015-0267) of National Cancer Center, Korea. Informed consent was obtained from patients in accordance with the Declaration of Helsinki.
The sample preparation and pyrosequencing for JAK2 V617F genotyping and quantification were performed according to a previously published protocol [18]. After PCR, the biotinylated strand was captured on streptavidin Sepharose beads (Amersham Biosciences, Uppsala, Sweden) and annealed with sequencing primers for the strand. Pyrosequencing was performed separately for the strands using PSQ HS 96 Gold SNP Reagents and a PSQ HS 96 pyrosequencing machine (Biotage, Uppsala, Sweden).
Genomic DNA was extracted from the peripheral blood or bone marrow using Chemagic DNA blood kit (PerkinElmer, Baesweiler, Germany), according to the manufacturer's instructions. The JAK2 V617F mutation and allele burdens were determined by pyrosequencing. Extracted DNA was amplified using the following biotin-labeled primers: forward 5′-GAAGCAGCAAGTATGATGAGCA-3′; reverse 5′-TGCTCTGAGAAAGGCATTAGAA-3′. Single-stranded biotinylated templates were then isolated and sequenced using the sequencing primer 5′-TCTCGTCTCCACAGA-3′. Percentages of JAK2 V617F mutant alleles were determined using the Allele Frequency Quantification function in PyroMark Q24 Software 2.0, according to the manufacturer's specifications (Qiagen).
The ddPCR assay was performed with the same samples as used in diagnostic pyrosequencing. A PrimePCR™ ddPCR™ Mutation Detection Kit Assay (Bio-Rad Laboratories, Hercules, CA, USA) was used to detect a JAK2 V617F mutant allele ac-cording to the manufacturer's instructions. A QX200™ Droplet Generator (Bio-Rad Laboratories) was used to divide each DNA mixture into 20,000 droplets for each sample. Each mixture consisted of 10 µL of 2X ddPCR™ Super Mix for probes (Bio-Rad Laboratories, GmbH, Munich, Germany), which consisted of 1 µL of each target and reference amplification primer/probe mix (final concentration 450 nmol primers/250 nmol probe, respectively), 1 µL of the patient's genomic DNA template (20 ng/µL total genomic DNA), and 7 µL of sterile distilled water. The amplification cycle was as follows: 95℃ for 10 minutes, followed by 40 cycles at 94℃ for 30 seconds and 55℃ for 1 minute, and one final cycle at 98℃ for 10 minutes. The fluorescence signal for the wild type droplet was HEX, and the signal for the mutation droplet was FAM. A QX200™ Droplet Reader (Bio-Rad Laboratories) and QuantaSoft™ Software v.1.6 (Bio-Rad Laboratories, Pleasanton, CA, USA) were used in reading amplified droplets and analyzing data.
All statistical analyses were performed using Stata version 13.1 software (StataCorp LP, College Station, TX, USA). Since data from pyrosequencing and ddPCR were not normally distributed, to compare quantitative variables, median values and interquartile ranges were calculated. Correlation coefficients were calculated by a Spearman rank correlation coefficient analysis. Quantitative variables were analyzed with a Student's paired t-test or one-way ANOVA. All tests were two-sided, and P≤0.05 was accepted as indicating a statistically significant difference.
To validate the detection limit of ddPCR, the correlation between the expected and measured JAK2 V617F detection sensitivity was determined according to previously reported methods [1219]. A positive control sample was serially diluted to achieve concentrations of 0.0001%, 0.001%, 0.01%, 0.1%, 1%, 10%, 50%, and 100% (Fig. 1), and the JAK2 V617F allele was quantified in eight independent ddPCR experiments. The correlation coefficient between the expected and measured allele burden was 0.9458 (P=0.0004; Fig. 2A). The detection limit of ddPCR was reported to be as low as 0.001% in previous studies [15162021]. However, the lowest value of the JAK2 V617F allele as measured by ddPCR (except for negative samples) in our study was 0.01%, corresponding to approximately 0.07 copies/µL of the mutant allele. As several discrepancies were observed for concentrations between 0.0001% and 0.01% for expected and measured values in the ddPCR detection sensitivity assay, 0.1% was determined as the cut-off value.
Of the 56 patients, 42 (75%) and 46 (82.1%) were positive for the JAK2 V617F mutation by pyrosequencing and ddPCR, respectively. The mean±SD mutated allele burden at diagnosis was 37.5±30.1% with a range of 10–100%. With ddPCR, the mean mutated allele burden at diagnosis was 40.7±31.2% with a range of 0.02–96.87%. The quantification results of the mutant allele burden from pyrosequencing and ddPCR were highly correlated according to a Spearman correlation coefficient of 0.9712 (P<0.001; Fig. 2B). The ddPCR technique identified a JAK2 V617F mutant allele in all patients who were found to be positive according to pyrosequencing. Furthermore, the JAK2 V617F mutant allele was detected by ddPCR in four more patients who were determined to be negative for the mutation by pyrosequencing. Two of these patients had ET, and the other two had MF that had developed from ET. Including these latter two cases, the mutant allele amounts from the three patients were 0.02%, 0.06%, and 0.08%, respectively. These were classified as negative results, because they were less than the cut-off (0.1%); two patients with the lowest values had a CALR exon 9 mutation. The amount of the JAK2 mutant allele of the last patient with ET was 0.17% by ddPCR, which was accepted as a positive value, and then became negative after hydroxyurea treatment. A comparison of JAK2 wild-type and V617F mutant alleles with representative scatter plots is shown in Fig. 3.
We compared quantification of the JAK2 V617F mutant allele according to subgroups of MPN. The absolute JAK2 V617F burden measured by ddPCR was significantly different according to the subgroup (P=0.0012). In pairwise comparisons of the subgroups, patients with PV showed an increased amount of the mutant allele compared with those with ET (P=0.001); however, differences in the PV–MF and ET–MF comparisons were not statistically significant (P=0.848 and P=0.097). There was also a significant difference between PV and ET in absolute mutant DNA copy numbers (P=0.014). Detailed values are presented in Table 1.
Follow-up samples were available for 12 patients with a median duration of 12.2 months between treatment start and follow-up sampling, and eight out of 12 patients were JAK2 V617F positive. We reviewed the clinical course of eight of these patients, excluding three patients who had the wild-type JAK2 allele and one patient who was negative. Of these, a reduction in the mutant allele burden after treatment was observed in six patients (75%). The greatest decrease was observed in two PV patients; one received hydroxyurea cytoreductive therapy, and the other was treated with phlebotomy and low-dose aspirin. The noted decrease in the mutant allele burden showed a tendency to be synchronized with the degree of hematologic improvement. A patient with MF (No. 5) treated with a JAK2 inhibitor showed an initial reduction of the mutant allele burden; however, after administration of a reduced dose because of the development of drug toxicities, an increment in the JAK2 V617F mutation load was observed, which was accompanied by hematologic aggravation. One of the patients with PV (No. 6) was treated with interferon alpha and ceased treatment following hematologic improvement. The patient's follow-up sample was obtained right after treatment was restarted owing to disease aggravation, which showed an increase in the mutation allele amount. Detailed characteristics and values for these samples are described in Table 2.
In this study, we validated ddPCR as an effective diagnostic tool to detect JAK2 V617F mutations in MPN compared with pyrosequencing. Our results suggest that alterations in quantitative JAK2 mutations may have a tendency to become synchronized with clinical variations during the disease course.
Although the threshold of detection sensitivity is recommended to be at least 1–3% in order to identify the JAK2 V617F mutant allele, a standard assay to measure the exact amount of this allele has not yet been established [5]. Several methods such as Sanger sequencing and pyrosequencing are commonly employed to detect genetic aberrations because of the ease and convenience of these techniques. However, some assays are not quantitative, have low sensitivity, and/or display inconsistent results depending on the quality of samples. To overcome such limitations, several novel techniques have been developed, including ddPCR. In our study, the mutational load of JAK2 V617F as determined by ddPCR and pyrosequencing showed a strong positive correlation, which is consistent with the outcomes of previous studies [1019]. ddPCR was able to detect very small amounts of the mutant allele that were missed by pyrosequencing. Therefore, ddPCR might be applied to the diagnosis of MPN as a reliable and precise tool that is superior to pyrosequencing. In particular, the small amount of JAK2 mutation existing after an allogeneic hematopoietic stem cell transplantation could be detected by ddPCR and introduced as a prognostic biomarker [1922].
The DNA source used in our study was peripheral whole blood. To date, almost all studies evaluating the quantitative JAK2 allele burden have also used DNA extracted from whole blood. Several qualitative studies have used DNA from isolated granulocytes [2324], and a previous study on PV demonstrated that the DNA source might induce slight differences in the results [10]; however, this discrepancy does not appear to be significantly great (52.9% from isolated granulocytes [25] vs 54.3% in this study). As ddPCR measures the absolute copies of mutated DNA regardless of sample qualities, a variable source of DNA may be acceptable.
The JAK2 V617F allele burden has been known to differ according to the subgroup of MPN [26]. A significantly greater amount of mutant allele and absolute copy numbers were noted in patients with PV compared with those with ET. Recent reports have suggested that ET is associated with the lowest allele burden, followed by PV, primary MF, and post-PV MF [726]. In our study, the mutant allele burden tended to be slightly higher in patients with PV than in those with MF, but without a significant difference noted. Of the 12 patients with MF, two (16.7%) developed from ET, and only one (8.3%) developed from PV. The relatively small number of post-PV patients and the short duration of follow-up to produce a sufficient amount of the mutant allele could explain this discrepancy.
Several studies have suggested that the JAK2 V617F mutational load correlated with disease course and could therefore be a predictive marker [26]. The JAK2 mutational load decreased in almost 80% of patients whose samples were available after cytoreductive or target therapy in our study. Hematologic improvement correlated with a reduction in the JAK2 V617F mutational load, which was consistent with the previous results [27]. The degree of quantitative decline was more definite for PV than for ET and MF. Quantitative variation is a result of the allelic homozygosity reported in 25–30% of PV patients, whereas most ET patients have a heterozygous status [28]. Interestingly, patient No. 5, who was diagnosed as having MF and received 15 mg of the JAK2 inhibitor ruxolitinib twice daily (as the recommended starting dose), showed an initial decrement in the JAK2 burden. However, the patient's mutational load increased after a dose reduction to 5 mg twice daily due to gastrointestinal toxicities, which was accompanied by hematologic aggravation. This finding may indicate that the JAK2 mutational load measured by ddPCR changes according to the treatment response and thus may be a potential candidate prognostic predictor. Although the survival benefit is still in question, the clinical benefit of ruxolitinib has been well studied [293031]. Patient No. 6, who was diagnosed as having PV, received interferon alpha but ceased treatment with hematologic improvement. As the disease became aggravated during the drug rest, this patient started to receive the treatment again, and the follow-up sample was obtained right after treatment was restarted. This sample showed an increase in the mutation allele compared with that in the diagnostic sample because the effectiveness of treatment had not yet presented at that time. As the quantity of the JAK2 V617F mutation varies with treatment, the use of ddPCR shows promise for detecting treatment responders earlier in the disease course.
There are several limitations of our study. Because some patients with MPN show indolent progression over several decades, a 12-month term between the start of treatment and follow-up appears to be relatively short. However, this period was sufficient to show a reduction in the JAK2 mutational load with hematologic improvement. The use of ddPCR for measuring other molecular aberrations such as JAK2 exon 12, or CALR and MPL mutations was not investigated. Therefore, longer follow-up studies with survival analyses using ddPCR in a larger population to quantify JAK2 V617F and other mutations are needed. In conclusion, quantitative analysis of the JAK2 V617F mutation using ddPCR correlates highly with pyrosequencing and may reflect the response of MPN to clinical treatment.
Acknowledgements
This work was supported by grants from the National Cancer Center, Goyang, Korea (grant number 1531880).
References
1. Swerdlow S, Campo E, Harris NL, Jaffe ES, Pileri SA, Stein S, et al. WHO Classification of Tumours of Haematopoietic and Lymphoid Tissues. 4th ed. Lyon: IARC;2008.
2. Cankovic M, Whiteley L, Hawley RC, Zarbo RJ, Chitale D. Clinical performance of JAK2 V617F mutation detection assays in a molecular diagnostics laboratory: evaluation of screening and quantitation methods. Am J Clin Pathol. 2009; 132:713–721. PMID: 19846812.
3. Kralovics R, Passamonti F, Buser AS, Teo SS, Tiedt R, Passweg JR, et al. A gain-of-function mutation of JAK2 in myeloproliferative disorders. N Engl J Med. 2005; 352:1779–1790. PMID: 15858187.
4. Steensma DP, Dewald GW, Lasho TL, Powell HL, McClure RF, Levine RL, et al. The JAK2 V617F activating tyrosine kinase mutation is an infrequent event in both “atypical” myeloproliferative disorders and myelodysplastic syndromes. Blood. 2005; 106:1207–1209. PMID: 15860661.
5. Busque L, Porwit A, Day R, Olney HJ, Leber B, Ethier V, et al. Laboratory investigation of myeloproliferative neoplasms (MPNs): recommendations of the Canadian Mpn Group. Am J Clin Pathol. 2016; 146:408–422. PMID: 27686169.
6. Arber DA, Orazi A, Hasserjian R, Thiele J, Borowitz MJ, Le Beau MM, et al. The 2016 revision to the World Health Organization classification of myeloid neoplasms and acute leukemia. Blood. 2016; 127:2391–2405. PMID: 27069254.
7. Passamonti F, Rumi E. Clinical relevance of JAK2 (V617F) mutant allele burden. Haematologica. 2009; 94:7–10. PMID: 19118374.
8. Borowczyk M, Wojtaszewska M, Lewandowski K, Gil L, Lewandowska M, Lehmann-Kopydlowska A, et al. The JAK2 V617F mutational status and allele burden may be related with the risk of venous thromboembolic events in patients with Philadelphia-negative myeloproliferative neoplasms. Thromb Res. 2015; 135:272–280. PMID: 25559461.
9. Campbell PJ, Griesshammer M, Dohner K, Dohner H, Kusec R, Hasselbalch HC, et al. V617F mutation in JAK2 is associated with poorer survival in idiopathic myelofibrosis. Blood. 2006; 107:2098–2100. PMID: 16293597.
10. Koren-Michowitz M, Landman J, Cohen Y, Rahimi-Levene N, Salomon O, Michael M, et al. JAK2 V617F allele burden is associated with transformation to myelofibrosis. Leuk Lymphoma. 2012; 53:2210–2213. PMID: 22524513.
11. Tefferi A, Lasho TL, Huang J, Finke C, Mesa RA, Li CY, et al. Low JAK2 V617F allele burden in primary myelofibrosis, compared to either a higher allele burden or unmutated status, is associated with inferior overall and leukemia-free survival. Leukemia. 2008; 22:756–761. PMID: 18216871.
12. Kinz E, Leiherer A, Lang AH, Drexel H, Muendlein A. Accurate quantitation of JAK2 V617F allele burden by array-based digital PCR. Int J Lab Hematol. 2015; 37:217–224. PMID: 24963593.
13. Bench AJ, White HE, Foroni L, Godfrey AL, Gerrard G, Akiki S, et al. Molecular diagnosis of the myeloproliferative neoplasms: UK guidelines for the detection of JAK2 V617F and other relevant mutations. Br J Haematol. 2013; 160:25–34. PMID: 23057517.
14. Sanmamed MF, Fernandez-Landazuri S, Rodriguez C, Zarate R, Lozano MD, Zubiri L, et al. Quantitative cell-free circulating BRAF V600E mutation analysis by use of droplet digital PCR in the follow-up of patients with melanoma being treated with BRAF inhibitors. Clin Chem. 2015; 61:297–304. PMID: 25411185.
15. Hindson BJ, Ness KD, Masquelier DA, Belgrader P, Heredia NJ, Makarewicz AJ, et al. High-throughput droplet digital PCR system for absolute quantitation of DNA copy number. Anal Chem. 2011; 83:8604–8610. PMID: 22035192.
16. Zhang Y, Xu Y, Zhong W, Zhao J, Chen M, Zhang L, et al. Total DNA input is a crucial determinant of the sensitivity of plasma cell-free DNA EGFR mutation detection using droplet digital PCR. Oncotarget. 2017; 8:5861–5873. PMID: 28052016.
17. Otsuji K, Sasaki T, Tanaka A, Kunita A, Ikemura M, Matsusaka K, et al. Use of droplet digital PCR for quantitative and automatic analysis of the HER2 status in breast cancer patients. Breast Cancer Res Treat. 2017; 162:11–18. PMID: 28039535.
18. Kim HR, Choi HJ, Kim YK, Kim HJ, Shin JH, Suh SP, et al. Allelic expression imbalance of JAK2 V617F mutation in BCR-ABL negative myeloproliferative neoplasms. PLoS One. 2013; 8:e52518. PMID: 23349688.
19. Waterhouse M, Follo M, Pfeifer D, von Bubnoff N, Duyster J, Bertz H, et al. Sensitive and accurate quantification of JAK2 V617F mutation in chronic myeloproliferative neoplasms by droplet digital PCR. Ann Hematol. 2016; 95:739–744. PMID: 26931113.
20. Reid AL, Freeman JB, Millward M, Ziman M, Gray ES. Detection of BRAF-V600E and V600K in melanoma circulating tumour cells by droplet digital PCR. Clin Biochem. 2015; 48:999–1002. PMID: 25523300.
21. Watanabe M, Kawaguchi T, Isa S, Ando M, Tamiya A, Kubo A, et al. Ultra-sensitive detection of the pretreatment EGFR T790M mutation in non-small cell lung cancer patients with an EGFR-activating mutation using droplet digital PCR. Clin Cancer Res. 2015; 21:3552–3560. PMID: 25882755.
22. Lange T, Edelmann A, Siebolts U, Krahl R, Nehring C, Jakel N, et al. JAK2 V617F allele burden in myeloproliferative neoplasms one month after allogeneic stem cell transplantation significantly predicts outcome and risk of relapse. Haematologica. 2013; 98:722–728. PMID: 23300178.
23. Baxter EJ, Scott LM, Campbell PJ, East C, Fourouclas N, Swanton S, et al. Acquired mutation of the tyrosine kinase JAK2 in human myeloproliferative disorders. The Lancet. 2005; 365:1054–1061.
24. Benati M, Montagnana M, Danese E, De Matteis G, Veneri D, Paviati E, et al. Role of JAK2 V617F mutation and aberrant expression of microRNA-143 in myeloproliferative neoplasms. Clin Chem Lab Med. 2015; 53:1005–1011. PMID: 25527813.
25. Passamonti F, Rumi E, Pietra D, Elena C, Boveri E, Arcaini L, et al. A prospective study of 338 patients with polycythemia vera: the impact of JAK2 (V617F) allele burden and leukocytosis on fibrotic or leukemic disease transformation and vascular complications. Leukemia. 2010; 24:1574–1579. PMID: 20631743.
26. Alshemmari SH, Rajaan R, Ameen R, Al-Drees MA, Almosailleakh MR. JAK2 V617F allele burden in patients with myeloproliferative neoplasms. Ann Hematol. 2014; 93:791–796. PMID: 24362471.
27. Jones AV, Silver RT, Waghorn K, Curtis C, Kreil S, Zoi K, et al. Minimal molecular response in polycythemia vera patients treated with imatinib or interferon alpha. Blood. 2006; 107:3339–3341. PMID: 16352805.
28. Vannucchi AM, Antonioli E, Guglielmelli P, Rambaldi A, Barosi G, Marchioli R, et al. Clinical profile of homozygous JAK2 617V>F mutation in patients with polycythemia vera or essential thrombocythemia. Blood. 2007; 110:840–846. PMID: 17379742.
29. Cervantes F, Vannucchi AM, Kiladjian JJ, Al-Ali HK, Sirulnik A, Stalbovskaya V, et al. Three-year efficacy, safety, and survival findings from COMFORT-II, a phase 3 study comparing ruxolitinib with best available therapy for myelofibrosis. Blood. 2013; 122:4047–4053. PMID: 24174625.
30. Cervantes F, Pereira A. Does ruxolitinib prolong the survival of patients with myelofibrosis? Blood. 2017; 129:832–837. PMID: 28031182.
31. Vannucchi AM, Kiladjian JJ, Griesshammer M, Masszi T, Durrant S, Passamonti F, et al. Ruxolitinib versus standard therapy for the treatment of polycythemia vera. N Engl J Med. 2015; 372:426–435. PMID: 25629741.
Fig. 1
Consistency of the expected and measured JAK2 V617F mutation percentages in the range of 0.0001–100% with pilot samples. Eight dilutions were measured in eight independent droplet digital PCR experiments.

Fig. 2
Correlation and agreement between (A) the expected and measured JAK2 V617F mutation percentages in the range of 0.0001–100% with pilot samples. (B) pyrosequencing and ddPCR results for quantifiable JAK2 V617F mutation levels in clinical samples.
Abbreviation: ddPCR, droplet digital PCR.

Fig. 3
Representative two-dimensional scatter plots of (A) wild-type JAK2 V617F (green droplets) in a healthy person and (B) heterozygous JAK2 V617F mutation (blue droplets) in a patient with MPN detected by ddPCR and pyrosequencing. Orange droplets indicate a sample heterozygous for the JAK2 V617F mutation and JAK2 wild-type.
Abbreviations: MPN, myeloproliferative neoplasm; ddPCR, droplet digital PCR.

Table 1
Hematologic characteristics and JAK2 V617F load according to MPN subgroups*
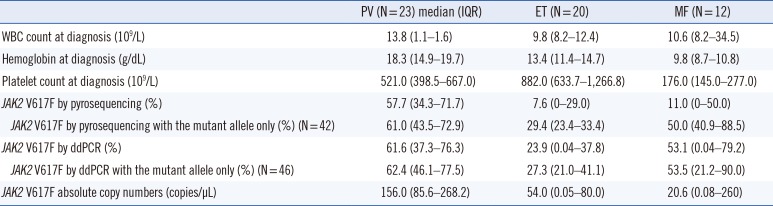
Table 2
Hematologic characteristics and JAK2 V617F load at initial diagnosis and follow-up after treatment
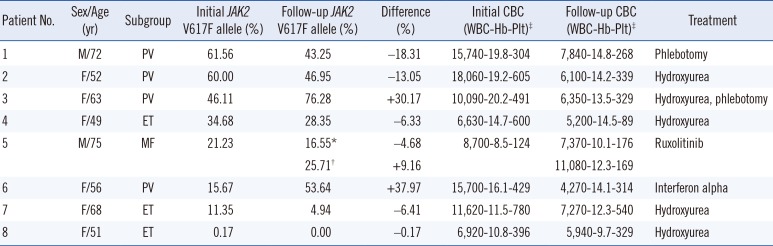
*Data from the first follow-up sample; †Data from the next follow-up sample for the same patient; ‡WBC, ×103/µL; Hb, g/dL; Plt, ×103/µL.
Abbreviations: M, male; F, female; PV, polycythemia vera; ET, essential thrombocytosis; MF, myelofibrosis; CBC, complete blood count; WBC, white blood cell; Plt, platelet.