Abstract
In the past several years, interest in the clinical utility of cell-free DNA as a noninvasive cancer biomarker has grown rapidly. Success in the development of plasma genotyping assays and other liquid biopsy assays has widened the scope of cell-free DNA use in research and the clinic. Already approved by the US Food and Drug Administration in the narrow context of epidermal growth factor receptor-mutated non-small cell lung cancer, plasma genotyping assays are currently being investigated in a wide array of clinical settings and modalities. These include plasma genotyping as a tool for early diagnosis, the detection of minimal residual disease, and the evaluation of treatment response/progression. In this review, we assess the clinical landscape of plasma genotyping assays and propose strategies for their further expansion into routine clinical care.
Nearly 70 years after the discovery of cell-free DNA (cfDNA) by Mandel and Metais [1], plasma genotyping of cfDNA is on the brink of transforming cancer care. cfDNA is free-floating DNA present in the blood and other bodily fluids [234]. In addition to its applications in oncology, cfDNA analysis is used extensively for prenatal genetic testing [567]. Because of our already expansive and ever-accelerating understanding of cancer genetics, the cfDNA component of liquid biopsy assays is particularly intriguing in oncology, in which plasma genotyping assays can noninvasively detect and quantify clinically relevant point mutations, insertions/deletions, amplifications, rearrangements, and aneuploidy within cfDNA [89]. Plasma genotyping has potential clinical utility in early diagnosis, the detection of minimal residual disease (MRD), and the evaluation of treatment response and resistance (Fig. 1). In 2016, Roche's Cobas plasma epidermal growth factor receptor (EGFR) mutation test V2 (US-IVD) was approved by the US Food and Drug Administration (FDA) to guide treatment decisions in patients with lung cancer who are unable to undergo tissue biopsy; this is still the only FDA-approved plasma genotyping assay [10]. At present, clinical assessment of cancer primarily consists of invasive tissue biopsies and radiation-emitting scans [111213]. In addition to its noninvasive nature, plasma genotyping has several advantages, including longitudinal monitoring, short turnaround time, low cost, and the ability to capture heterogeneity, which is sometimes missed by tissue biopsy, within tumors and between metastatic lesions [141516]. The realization of these benefits, however, is neither indubitable nor possible without significant further work.
In this review, we detail the clinical landscape of cfDNA analysis in oncology and the necessary next steps for further clinical adoption of this promising technology. Over the past five years, strong concordance has been shown between plasma- and tissue-based genomic assays, encouraging “observational” trials that explore the potential clinical utility of plasma genotyping. Success in completed observational trials has spurred the recent initiation of several “interventional” trials to test the value of incorporating cfDNA results into routine clinical care (Fig. 2).
Clinical use of cfDNA analysis requires exceedingly accurate assays for the genetic characterization of DNA fragments within the fluid of interest, often blood. These assays must have high analytical sensitivity to detect clinically relevant genetic alterations in a high background of wild-type DNA shed by nonmalignant cells. Low allelic frequencies (AF, <0.5% mutant AF) are commonly seen in patients, particularly in the context of early detection or MRD. In addition, exquisite specificity is required because false positives can lead to further unnecessary, invasive testing or inappropriate treatment adjustment. Various groups have developed valuable tools to increase the analytic specificity of assays. The use of molecular tags and improved bioinformatics (e.g. polishing [17]) has dramatically improved the specificity of next-generation sequencing (NGS) assays [17181920]. Specificity considerations are even more important in the context of early detection.
Several highly sensitive and specific platforms exist for the detection and quantification of genetic alterations within cfDNA. More targeted approaches that investigate focused alterations, often point mutations, in single alleles include digital PCR [21], BEAMing [22], and Scorpion ARMS PCR [23]. NGS permits broader inquiries, allowing assessment of the mutation status of thousands/millions of bases [17181920]. At present, ongoing clinical trials are evaluating the concordance of cfDNA alterations in plasma, using digital PCR or NGS platforms, with DNA alterations identified in tumor tissue. Notably, Sacher and colleagues have published a prospective clinical trial demonstrating perfect specificity and 70–80% sensitivity for detection of EGFR driver mutations in non-small cell lung cancer (NSCLC) using allele-specific PCR [24]. In addition, prospective clinical trials testing NGS of plasma cfDNA in other malignancies are ongoing [252627].
Cancer outcomes vary greatly based on the stage at detection; late-stage diagnoses are associated with increased mortality rates and treatment inefficacy [28293031]. Widespread clinical adoption of screening tools has diminished cancer morbidity and mortality in many malignancies; however, high rates of late-stage diagnoses underscore the desperate need for better screening capabilities. The development of accurate plasma genotyping assays described above has generated interest in the potential use of cfDNA analysis as a diagnostic tool.
Early detection demands biomarkers specific to disease that are detectable at an early stage. To assess the qualifications of cfDNA as such a biomarker, Bettegowda and colleagues performed digital PCR on cfDNA from 640 patients with different types and stages of cancer [32]. While rates of tumor DNA present in plasma differed by tissue of origin, 47% of stage I cancers and 55% of stage II cancers had detectable circulating tumor DNA (ctDNA). Plasma genotyping assays with greater sensitivity (<0.2% AF) and breadth (as the genetic alterations promoting tumorigenesis are not known prior to the screen) could raise the detection rates of early-stage cancers [32]. Indeed, several biotechnology companies are pursuing the development of NGS assays that sequence deeper and broader, requiring larger cfDNA input and greater sequencing power [3334]. The ultimate goal of these assays is to use them for early detection in high-risk populations. Although interest in population screening is growing, current assay specificity is not amenable to such screens, where the prior probability of any patient having cancer is very low. Bettegowda's extensive work provides confidence that many early-stage cancers shed DNA into the blood, but the presence of mutated DNA fragments in the blood does not necessarily indicate the presence of a malignancy [32].
cfDNA analysis of healthy volunteers has provided insights into specificity concerns with the use of plasma genotyping as a diagnostic tool. In a longitudinal study that followed healthy volunteers, 33 of more than 1,000 volunteers were shown to have either a KRAS or TP53 mutation in their blood. Interestingly, 16 of these 33 developed cancer after an average of 18.3 months, showing the potential for early detection with plasma genotyping [35]. The potential origin of these mutations may be the hematopoietic compartment in the context of clonal hematopoiesis of indeterminate potential [36]. However, these data also highlight the challenges surrounding population-wide screens, particularly the rate of false positives due to rare and unexplained cancer-related mutations in the blood of healthy volunteers. These findings necessitate a reformulation of how we think about cfDNA as a biomarker. In particular, the presence of cancer-related mutations in the blood does not axiomatically prove the presence of an underlying malignancy. This complicates all cfDNA analysis, but is particularly important to the future of diagnostic plasma genotyping.
There are several methods to limit false positives and to investigate the common, but not absolute, link between cancer-related mutations within the blood and underlying malignancies. First, if a mutant DNA molecule has found its way into the blood from a cancerous cell, then broad sequencing of cfDNA should detect other cancer-related mutations, such as activating mutations in oncogenes or inactivating mutations in tumor suppressors. Thus, deep and broad sequencing could provide the sensitivity needed to detect low levels of cfDNA alterations in patients with early-stage disease. Indeed, Abbosh et al [37] performed multiregion whole-exome sequencing of early-stage NSCLC tumors to show an abundance of clonal mutations in these tumors. Assaying plasma for a broad panel of patient-specific clonal single nucleotide variants (SNVs) provides greater sensitivity. However, broad panels are associated with specificity issues. Abbosh et al [37] provided an intriguing solution to this issue by requiring the detection of two or more SNVs for the determination of the presence of cancer [37]. Therefore, optimal cfDNA assay development for cancer detection may require a combination of broader panels (to increase sensitivity) and stricter calling methods (to increase specificity). Second, studies investigating methylation patterns of cfDNA suggested the possibility of understanding the tissue-of-origin makeup of cfDNA [383940]. Lehmann-Werman and colleagues analyzed methylation patterns to distinguish the relative proportions of cfDNA shed by each organ [38]. An early diagnosis tool should provide information on both the genetics and location of the cancer. A tissue-of-origin assay could potentially locate the malignancy. Upon discovering a KRAS mutation in the cfDNA, for example, one may see an increase in cfDNA from the pancreas by methylation analysis, potentially indicating a pancreatic adenocarcinoma driven by mutant KRAS. Third, waiting and re-assaying the plasma weeks or months later is perhaps the best way to distinguish signal from noise. Any false positive would most likely not be seen in a re-assay. Malignancies will likely be detected again, potentially at a higher AF, indicating a growing tumor. These three ideas will help clinicians and researchers discard false positives (or true mutations that have arisen from hematopoietic events) and solidify the link between plasma genotyping results, and clinically relevant malignancies.
Ultimately, clinical adoption of cfDNA assays will only occur if their use improves patient outcomes. Because many malignancies are detected too late, the clinical utility of a highly sensitive and specific early diagnostic test is obvious. However, it remains unclear whether plasma genotyping assays can achieve the necessary sensitivity and specificity of such a diagnostic test. Furthermore, clinical care in this setting is ill-defined. Interventional clinical trials that assess clinical decision-making using results from such assays are needed to prove clinical efficacy. Monitoring high-risk populations with diagnostic cfDNA assays is an obvious next step and is being pursued [2526]. Observational trials in early detection investigate whether cancer variants found by plasma genotyping will lead to eventual cancer diagnosis. Large-scale observational trials funded by private ventures have been announced [252627]. Upon obtaining successful results in observational trials, treating patients based on plasma results in interventional trials will need to show improved outcomes over the current process of diagnosing and treating cancer. Larger consortiums with immense resources and vast clinical infrastructure should play a key role in carrying out these large-scale studies [41].
Patients that have undergone curative surgery or are in complete remission frequently show recurrence due to the presence of previously undetected MRD [424344]. Early detection of MRD can inform decisions regarding adjuvant therapy and improve outcomes while preventing overtreatment [4546]. However, detection of such recurrence is currently limited to radiation-emitting scans, the development of clinically relevant symptoms, or protein biomarkers with poor sensitivity and specificity [4748]. Thus, new biomarkers to predict MRD before radiologic or clinical detection are urgently needed. Recently, researchers have investigated the presence of plasma tumor DNA post-surgery or other curative treatments and the ability to predict eventual recurrence.
Extensive studies of plasma tumor DNA as a prognostic biomarker for MRD in breast cancer [495051], colorectal cancer [525354] diffuse large B-cell lymphoma (DLBCL) [55], gastric cancer [56], lung cancer [37], and pancreatic cancer [57] have established the high predictive power of plasma tumor DNA and the early detection of recurrence using this marker. In perhaps the largest prospective clinical trial of MRD to date, Tie and colleagues performed NGS of 1,046 plasma samples from 230 patients with resected stage II colorectal cancer. They showed impressive positive (80%) and negative (97%) predictive values. Furthermore, they found that plasma tumor DNA is a better marker for recurrence than carcinoembryonic antigen (CEA), which is currently used in the clinical setting [52]. In the same setting, Reinert and colleagues showed perfect sensitivity and specificity of their NGS- and digital PCR-based assays in predicting recurrence after curative surgery. In addition, plasma genotyping-based recurrence detection preceded detection by conventional follow-up by 10 months [53]. Roschewski et al [55] followed 107 patients with DLBCL after complete remission and confirmed the strong positive (88%) and negative (98%) predictive values of plasma tumor DNA for assessing recurrence. Additionally, similar to Reinert's study, Roschewski et al [55] found that the presence of plasma tumor DNA often occurs before evidence of clinical disease (3–5 months). In breast cancer, Olsson et al [49] and Garcia-Murillas et al [50] showed the high predictive power of plasma tumor DNA and its lead time of several months over clinical detection of recurrence. Abbosh and colleagues performed a prospective clinical trial in NSCLC using patient-specific NGS assays to predict MRD with high sensitivity (92.3%) and specificity (100%), with a median lead time of 70 days over computed tomography (CT) imaging [37]. Notably, Sausen and colleagues followed patients with pancreatic cancer after curative surgery and showed that plasma tumor DNA predicts recurrence accurately and with a median lead time of 6.5 months over imaging [57].
While this list does not include ongoing MRD studies using plasma tumor DNA as a prognostic biomarker, it confirms the importance of plasma genotyping in MRD detection. We believe that these studies provide ample evidence to begin interventional trials assigning adjuvant therapy, or the resumption of therapy, upon plasma genotyping-based recurrence determination after either curative surgery or complete remission. However, standardization of cfDNA analysis in MRD is lagging. The studies listed above use varying platforms including targeted NGS, digital PCR, and low-coverage whole-genome sequencing. Clinical adoption of the most sensitive and specific assays and assays that are most amenable to longitudinal monitoring is needed. In MRD, as in diagnosis, specificity takes precedence over sensitivity because overtreatment of false positives introduces unnecessary harm. We envision clinical monitoring for recurrence in addition to plasma genotyping because the sensitivity of these assays is imperfect. Overall, current data suggest that plasma tumor DNA is a highly sensitive and specific biomarker of disease recurrence and justify the initiation of interventional trials using cfDNA analysis to guide clinical decision-making.
In addition to its capacity to determine the presence of a malignancy in diagnosis and MRD, plasma genotyping can also be used to guide the choice of therapeutic agent and to monitor dynamic tumor responses throughout treatment. Genotype-directed personalized therapy in oncology has been shown to be successful due to our deep understanding of cancer genetics and advances in drug development of targeted agents. Tissue biopsy genotyping is the current gold standard for collecting tumor genotype data. Due to the many advantages discussed above, plasma genotyping has been extensively studied for the determination of progression and for the characterization of genetic alterations that drive resistance. Plasma progression, i.e., the reemergence or significant increase in plasma tumor DNA during drug treatment, is strongly correlated with radiographic/clinical progression [172058]. Several studies have shown that plasma progression can predict progression with a lead-time of months over radiographic progression [165960]. This has led to the initiation of an interventional clinical trial seeking to examine outcomes of switching treatment at the time of plasma progression versus waiting for radiographic or clinical progression, as is standard of care [61]. The outcome of this trial will determine the clinical future of cfDNA as a biomarker for the determination of progression.
In addition to identifying progression, cfDNA analysis can provide further details of the potential genetic causes of progression; plasma genotyping often illuminates the genetic mechanisms of resistance seen in the tumor [125962636465666768]. A major success of plasma genotyping has been the ubiquitous clinical use of cfDNA analysis at progression to the first-generation EGFR tyrosine kinase inhibitors (TKIs) erlotinib and gefitinib in patients with NSCLC. Over 50% of patients with lung cancer taking these agents will develop the EGFR T790M mutation, which confers acquired resistance to erlotinib/gefitinib [69]. The recent approval of osimertinib [70], a drug that targets the EGFR T790M resistance mechanism, makes this an optimal setting for potential displacement of the current standard of care (tissue rebiopsy) with cfDNA analysis. Our group has shown the high positive predictive value of EGFR T790M mutations identified in plasma, thus redefining the paradigm of optimal care for erlotinib/gefitinib-resistant patients at progression [2460]. Whereas tissue rebiopsy is currently recommended for these patients at progression, our data suggest that assaying cfDNA for the EGFR T790M mutation will lead to equivalent outcomes and save many patients from invasive tissue rebiopsy [7172]. Thus, plasma genotyping at the time of progression to erlotinib/gefitinib should be performed. If T790M is detected, osimertinib should be administered. If not detected, a tissue rebiopsy is justified, as sensitivity for EGFR T790M mutation in the plasma is imperfect.
Further work characterizing genetic mechanisms of resistance to the growing list of FDA-approved targeted agents is necessary. Clinical adoption of plasma genotyping at progression requires well-defined and actionable mechanisms of resistance. Additional approvals of these types of targeted therapies will only increase the potential clinical use of plasma genotyping at progression. As with lung cancer resistant to first and second-generation EGFR TKIs, routine clinical use requires further clinical trials proving equivalent or improved outcomes in patients switching treatment based on cfDNA analysis versus tissue rebiopsy.
In addition to evaluating progression, cfDNA has been increasingly studied as a biomarker of response. Plasma response, a significant or complete decline in ctDNA during drug treatment, is highly concordant with successful response to therapy [1516172073747576]. At present, radiographic imaging and clinical assessment are the primary tools for evaluation of response. A major advantage of cfDNA as a biomarker of response is its non-invasive collection and ease of longitudinal monitoring. Due to its strong concordance with tissue response and the aforementioned logistical benefits, many recently initiated early-phase clinical trials have included cfDNA analysis as a biomarker of response. Our group showed the value of plasma tumor DNA as a pharmacodynamic biomarker in a phase 1 clinical trial [77]. Although imaging and clinical assessment will continue to be the foundation of response determination, cfDNA is an intriguing potential biomarker of response. We expect greater integration of cfDNA into early-phase clinical trials for noninvasive longitudinal monitoring of response, which has immense clinical utility.
A rapid expansion of research and clinical interest in cfDNA has occurred over the past five years. Academic and industry teams have developed assays to detect and quantify tumor DNA in the plasma with high sensitivity and specificity. The application of these assays has shown that plasma DNA and tumor tissue DNA are highly concordant. Potential areas of clinical adoption of cfDNA analysis include diagnosis, characterizing treatment response and progression, and identifying MRD. Widespread clinical use of plasma genotyping for these indications can only occur once the high threshold to displace the current standard of care is met. We believe that current evidence justifies the initiation of interventional clinical trials comparing outcomes of plasma genotyping-guided treatment against the standard of care. Such trials provide a crucial clinical test of plasma genotyping and will ultimately determine whether this approach can be used in the clinical setting. In no way should this deter further assay development of more sensitive and specific platforms for cfDNA analysis. The potential failure of such interventional trials may indicate flawed assays more than the flaws of cfDNA as a biomarker. Further studies are needed to elucidate whether cfDNA is indeed a clinically useful biomarker and whether current plasma genotyping assays are sufficient for detecting cfDNA. As plasma genotyping assays gear up for major clinical tests, these uncertainties still remain.
Figures and Tables
Fig. 1
Plasma genotyping assays can use cell-free DNA to longitudinally track dynamic cancer responses in diverse clinical situations. In particular, these assays can evaluate responses to targeted agents and the presence or recurrence of disease after curative surgery.
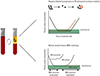
Fig. 2
The clinical trial landscape for cell-free DNA. (A) Three major clinical trial types conducted in the field of cell-free DNA analysis. Concordance trials assess the accuracy of plasma genotyping assays to capture genetic mutations found in tumor tissue. Observational trials explore the predictive capacity of cell-free DNA as a biomarker. Interventional trials compare treatment outcomes of clinical decision-making with plasma genotyping versus standard of care (imaging, tissue biopsy, etc.). (B) Graph of clinical trials registered to clinicaltrials.gov that have investigated some aspect of plasma genotyping in oncology. This highlights the rapid growth of interest in the clinical utility of cell-free DNA and the recent initiation of interventional trials.
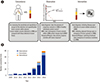
References
1. Mandel P, Metais P. Les acides nucleiques du plasma sanguin chez l'homme [in French]. C R Seances Soc Biol Fil. 1948; 142:241–243.
2. Jahr S, Hentze H, Englisch S, Hardt D, Fackelmayer FO, Hesch RD, et al. DNA fragments in the blood plasma of cancer patients: quantitations and evidence for their origin from apoptotic and necrotic cells. Cancer Res. 2001; 61:1659–1665.
3. Stroun M, Maurice P, Vasioukhin V, Lyautey J, Lederrey C, Lefort F, et al. The origin and mechanism of circulating DNA. Ann N Y Acad Sci. 2000; 906:161–168.


4. Stroun M, Lyautey J, Lederrey C, Olson-Sand A, Anker P. About the possible origin and mechanism of circulating DNA. Clin Chim Acta. 2001; 313:139–142.


5. Papageorgiou EA, Karagrigoriou A, Tsaliki E, Velissariou V, Carter NP, Patsalis PC. Fetal-specific DNA methylation ratio permits noninvasive prenatal diagnosis of trisomy 21. Nat Med. 2011; 17:510–513.


6. Lo YM, Corbetta N, Chamberlain PF, Rai V, Sargent IL, Redman CW, et al. Presence of fetal DNA in maternal plasma and serum. Lancet. 1997; 350:485–487.


7. Lo YM, Hjelm NM, Fidler C, Sargent IL, Murphy MF, Chamberlain PF, et al. Prenatal diagnosis of fetal RhD status by molecular analysis of maternal plasma. N Engl J Med. 1998; 339:1734–1738.


8. Leary RJ, Kinde I, Diehl F, Schmidt K, Clouser C, Duncan C, et al. Development of personalized tumor biomarkers using massively parallel sequencing. Sci Transl Med. 2010; 2:20ra14.


9. Leary RJ, Sausen M, Kinde I, Papadopoulos N, Carpten JD, Craig D, et al. Detection of chromosomal alterations in the circulation of cancer patients with whole-genome sequencing. Sci Transl Med. 2012; 4:162ra154.


10. Center for Drug Evaluation and Research. Approved Drugs - cobas EGFR Mutation Test v2 [Internet]. U S Food and Drug Administration Home Page. Center for Drug Evaluation and Research;Updated on Jun 2016. https://www.fda.gov/Drugs/InformationOnDrugs/ApprovedDrugs/ucm504540.htm.
11. Overman MJ, Modak J, Kopetz S, Murthy R, Yao JC, Hicks ME, et al. Use of research biopsies in clinical trials: are risks and benefits adequately discussed? J Clin Oncol. 2013; 31:17–22.


12. Murtaza M, Dawson S-J, Tsui DWY, Gale D, Forshew T, Piskorz AM, et al. Non-invasive analysis of acquired resistance to cancer therapy by sequencing of plasma DNA. Nature. 2013; 497:108–112.


13. Vanderlaan PA, Yamaguchi N, Folch E, Boucher DH, Kent MS, Gangadharan SP, et al. Success and failure rates of tumor genotyping techniques in routine pathological samples with non-small-cell lung cancer. Lung Cancer. 2014; 84:39–44.


14. Sundaresan TK, Sequist LV, Heymach JV, Riely GJ, Ja Nne PA, Koch WH, et al. Detection of T790M, the acquired resistance EGFR mutation, by tumor biopsy versus noninvasive blood-based analyses. Clin Cancer Res. 2016; 22:1103–1110.


15. Yanagita M, Redig AJ, Paweletz CP, Dahlberg SE, Oconnell A, Feeney N, et al. A prospective evaluation of circulating tumor cells and cell-free DNA in EGFR-mutant non-small cell lung cancer patients treated with erlotinib on a phase II trial. Clin Cancer Res. 2016; 22:6010–6020.


16. Oxnard GR, Paweletz CP, Kuang Y, Mach SL, Oconnell A, Messineo MM, et al. Noninvasive detection of response and resistance in EGFR-mutant lung cancer using quantitative next-generation genotyping of cell-free plasma DNA. Clin Cancer Res. 2014; 20:1698–1705.


17. Newman AM, Bratman SV, To J, Wynne JF, Eclov NCW, Modlin LA, et al. An ultrasensitive method for quantitating circulating tumor DNA with broad patient coverage. Nat Med. 2014; 20:548–554.


18. Kinde I, Wu J, Papadopoulos N, Kinzler KW, Vogelstein B. Detection and quantification of rare mutations with massively parallel sequencing. Proc Natl Acad Sci U S A. 2011; 108:9530–9535.


19. Schmitt MW, Kennedy SR, Salk JJ, Fox EJ, Hiatt JB, Loeb LA. Detection of ultra-rare mutations by next-generation sequencing. Proc Natl Acad Sci U S A. 2012; 109:14508–14513.


20. Forshew T, Murtaza M, Parkinson C, Gale D, Tsui DWY, Kaper F, et al. Noninvasive identification and monitoring of cancer mutations by targeted deep sequencing of plasma DNA. Sci Transl Med. 2012; 4:136ra68.


22. Dressman D, Yan H, Traverso G, Kinzler KW, Vogelstein B. Transforming single DNA molecules into fluorescent magnetic particles for detection and enumeration of genetic variations. Proc Natl Acad Sci U S A. 2003; 100:8817–8822.


23. Spindler KL, Pallisgaard N, Vogelius I, Jakobsen A. Quantitative cell-free DNA, KRAS, and BRAF mutations in plasma from patients with metastatic colorectal cancer during treatment with cetuximab and irinotecan. Clin Cancer Res. 2012; 18:1177–1185.


24. Sacher AG, Paweletz C, Dahlberg SE, Alden RS, O'Connell A, Feeney N, et al. Prospective validation of rapid plasma genotyping for the detection of EGFR and KRAS mutations in advanced lung cancer. JAMA Oncol. 2016; 2:1014–1022.
25. The STRIVE Study: Breast Cancer Screening Cohort [Internet]. The STRIVE Study: Breast Cancer Screening Cohort - Full Text View -. ClinicalTrials.gov;Updated on Aug 2017. https://clinicaltrials.gov/ct2/show/NCT03085888?term=NCT03085888&rank=1.
26. Utility of Plasma Circulating Tumor DNA (ctDNA) in Asymptomatic Subjects for the Detection of Neoplastic Disease (H1000) [Internet]. Utility of Plasma Circulating Tumor DNA (ctDNA) in Asymptomatic Subjects for the Detection of Neoplastic Disease - Full Text View -. ClinicalTrials;Updated on Apr 2017. https://clinicaltrials.gov/ct2/show/NCT02612350?term=NCT02612350&rank=1.
27. Spanish Lung Liquid vs. Invasive Biopsy Program (SLLIP) [Internet]. Spanish Lung Liquid vs. Invasive Biopsy Program (SLLIP) - Full Text View -. ClinicalTrials.gov;Updated on Sep 2017. https://clinicaltrials.gov/ct2/show/NCT03248089?spons=Guardant&rank=1.
28. Moyer VA. Screening for lung cancer: U.S. Preventive Services Task Force Recommendation Statement. Ann Intern Med. 2014; 160:330–338.


29. U.S. Preventive Services Task Force. Screening for colorectal cancer: U.S. Preventive Services Task Force Recommendation Statement. Ann Intern Med. 2008; 149:627.
30. U.S. Preventive Services Task Force. Screening for breast cancer: U.S. Preventive Services Task Force Recommendation Statement. Ann Intern Med. 2009; 151:716.
31. Moyer VA. U.S. Preventive Services Task Force. Screening for cervical cancer: U.S. Preventive Services Task Force Recommendation Statement. Ann Intern Med. 2012; 156:880.


32. Bettegowda C, Sausen M, Leary R, Kinde I, Agrawal N, Bartlett B, et al. Detection of circulating tumor DNA in early and late stage human malignancies. Sci Transl Med. 2014; 6:224ra24.


33. Science | GRAIL's Commitment to Scientific Rigor and Clinical Utility [Internet]. GRAIL;Update on 2017. https://grail.com/science/.
34. Reporter S. Guardant Health, Pharma Firms to Develop 500-Gene Liquid Biopsy Panel [Internet]. GenomeWeb;2017. https://www.genomeweb.com/drug-discovery-development/guardant-health-pharma-firms-develop-500-gene-liquid-biopsy-panel.
35. Gormally E. TP53 and KRAS2 mutations in plasma DNA of healthy subjects and subsequent cancer occurrence: a prospective study. Cancer Res. 2006; 66:6871–6876.


36. Zhang BM, Aleshin A, Lin CY, Ford J, Zehnder JL, Suarez CJ. IDH2 mutation in a patient with metastatic colon cancer. N Engl J Med. 2017; 376:1991–1992.


37. Abbosh C, Birkbak NJ, Wilson GA, Jamal-Hanjani M, Constantin T, Salari R, et al. Phylogenetic ctDNA analysis depicts early stage lung cancer evolution. Nature. 2017; 545:446–451.
38. Lehmann-Werman R, Neiman D, Zemmour H, Moss J, Magenheim J, Vaknin-Dembinsky A, et al. Identification of tissue-specific cell death using methylation patterns of circulating DNA. Proc Natl Acad Sci U S A. 2016; 113:E1826–E1834.


39. Sun K, Jiang P, Chan KC, Wong J, Cheng YK, Liang RH, et al. Plasma DNA tissue mapping by genome-wide methylation sequencing for non-invasive prenatal, cancer, and transplantation assessments. Proc Natl Acad Sci U S A. 2015; 112:E5503–E5512.


40. Kang S, Li Q, Chen Q, Zhou Y, Park S, Lee G, et al. CancerLocator: non-invasive cancer diagnosis and tissue-of-origin prediction using methylation profiles of cell-free DNA. Genome Biol. 2017; 18:53.


41. Alliance for Clinical Trials in Oncology [Internet]. Alliance Trials – Full Text View --. https://www.allianceforclinicaltrialsinoncology.org. https://www.allianceforclinicaltrialsinoncology.org/main/public/standard.xhtml?path=%2FPublic%2FAlliance-Trials.
42. Zimmermann FB, Molls M, Jeremic B. Treatment of recurrent disease in lung cancer. Semin Surg Oncol. 2003; 21:122–127.


43. Sadahiro S, Suzuki T, Ishikawa K, Nakamura T, Tanaka Y, Masuda T, et al. Recurrence patterns after curative resection of colorectal cancer in patients followed for a minimum of ten years. Hepatogastroenterology. 2003; 50:1362–1366.
44. Hourigan CS, Karp JE. Minimal residual disease in acute myeloid leukaemia. Nat Rev Clin Oncol. 2013; 10:460–471.


45. Figueredo A, Rumble RB, Maroun J, Earle CC, Cummings B, Mcleod R, et al. Follow-up of patients with curatively resected colorectal cancer: a practice guideline. BMC Cancer. 2003; 3:26.


46. Pita-Fernandez S, Alhayek-Ai M, Gonzalez-Martin C, Lopez-Calvino B, Seoane-Pillado T, Pertega-Diaz S. Intensive follow-up strategies improve outcomes in nonmetastatic colorectal cancer patients after curative surgery: a systematic review and meta-analysis. Ann Oncol. 2014; 26:644–656.
47. Chao M, Gibbs P. Caution is required before recommending routine carcinoembryonic antigen and imaging follow-up for patients with early-stage colon cancer. J Clin Oncol. 2009; 27:e279–e280.


48. Benson AB 3rd, Desch CE, Flynn PJ, Krause C, Loprinzi CL, Minsky BD, et al. 2000 Update of American Society of Clinical Oncology Colorectal Cancer Surveillance Guidelines. J Clin Oncol. 2000; 18:3586–3588.


49. Olsson E, Winter C, George A, Chen Y, Howlin J, Tang M-HE, et al. Serial monitoring of circulating tumor DNA in patients with primary breast cancer for detection of occult metastatic disease. EMBO Mol Med. 2015; 7:1034–1047.
50. Garcia-Murillas I, Schiavon G, Weigelt B, Ng C, Hrebien S, Cutts RJ, et al. Mutation tracking in circulating tumor DNA predicts relapse in early breast cancer. Sci Transl Med. 2015; 7:302ra133.


51. Beaver JA, Jelovac D, Balukrishna S, Cochran RL, Croessmann S, Zabransky DJ, et al. Detection of cancer DNA in plasma of patients with early-stage breast cancer. Clin Cancer Res. 2014; 20:2643–2650.


52. Tie J, Wang Y, Tomasetti C, Li L, Springer S, Kinde I, et al. Circulating tumor DNA analysis detects minimal residual disease and predicts recurrence in patients with stage II colon cancer. Sci Transl Med. 2016; 8:346ra92.


53. Reinert T, Schøler LV, Thomsen R, Tobiasen H, Vang S, Nordentoft I, et al. Analysis of circulating tumour DNA to monitor disease burden following colorectal cancer surgery. Gut. 2015; 65:625–634.


54. Frattini M, Gallino G, Signoroni S, Balestra D, Lusa L, Battaglia L, et al. Quantitative and qualitative characterization of plasma DNA identifies primary and recurrent colorectal cancer. Cancer Lett. 2008; 263:170–181.


55. Roschewski M, Dunleavy K, Pittaluga S, Moorhead M, Pepin F, Kong K, et al. Circulating tumour DNA and CT monitoring in patients with untreated diffuse large B-cell lymphoma: a correlative biomarker study. Lancet Oncol. 2015; 16:541–549.


56. Kim K, Shin DG, Park MK, Baik SH, Kim TH, Kim S, et al. Circulating cell-free DNA as a promising biomarker in patients with gastric cancer: diagnostic validity and significant reduction of cfDNA after surgical resection. Ann Surg Treat Res. 2014; 86:136.


57. Sausen M, Phallen J, Adleff V, Jones S, Leary RJ, Barrett MT, et al. Clinical implications of genomic alterations in the tumour and circulation of pancreatic cancer patients. Nat Commun. 2015; 6:7686.


58. Diehl F, Schmidt K, Choti MA, Romans K, Goodman S, Li M, et al. Circulating mutant DNA to assess tumor dynamics. Nat Med. 2007; 14:985–990.


59. Diaz LA Jr, Williams RT, Wu J, Kinde I, Hecht JR, Berlin J, et al. The molecular evolution of acquired resistance to targeted EGFR blockade in colorectal cancers. Nature. 2012; 486:537–540.


60. Oxnard GR, Thress KS, Alden RS, Lawrance R, Paweletz CP, Cantarini M, et al. Association between plasma genotyping and outcomes of treatment with osimertinib (AZD9291) in advanced non–small-cell lung cancer. J Clin Oncol. 2016; 34:3375–3382.


61. Blood Sample Monitoring of Patients With EGFR Mutated Lung Cancer [Internet]. Blood Sample Monitoring of Patients with EGFR Mutated Lung Cancer - Full Text View -. ClinicalTrials.gov;Update on Mar 2017. https://clinicaltrials.gov/ct2/show/NCT02284633?term=NCT02284633&rank=1.
62. Schiavon G, Hrebien S, Garcia-Murillas I, Cutts RJ, Pearson A, Tarazona N, et al. Analysis of ESR1 mutation in circulating tumor DNA demonstrates evolution during therapy for metastatic breast cancer. Sci Transl Med. 2015; 7:131ra182.


63. Lallous N, Volik SV, Awrey S, Leblanc E, Tse R, Murillo J, et al. Functional analysis of androgen receptor mutations that confer anti-androgen resistance identified in circulating cell-free DNA from prostate cancer patients. Genome Biol. 2016; 17:10.


64. Azad AA, Volik SV, Wyatt AW, Haegert A, Bihan SL, Bell RH, et al. Androgen receptor gene aberrations in circulating cell-free DNA: biomarkers of therapeutic resistance in castration-resistant prostate cancer. Clin Cancer Res. 2015; 21:2315–2324.


65. Chu D, Paoletti C, Gersch C, Vandenberg DA, Zabransky DJ, Cochran RL, et al. ESR1 mutations in circulating plasma tumor DNA from metastatic breast cancer patients. Clin Cancer Res. 2016; 22:993–999.


66. Siravegna G, Mussolin B, Buscarino M, Corti G, Cassingena A, Crisafulli G, et al. Clonal evolution and resistance to EGFR blockade in the blood of colorectal cancer patients. Nat Med. 2015; 21:795–801.


67. Morelli MP, Overman MJ, Dasari A, Kazmi SMA, Mazard T, Vilar E, et al. Characterizing the patterns of clonal selection in circulating tumor DNA from patients with colorectal cancer refractory to anti-EGFR treatment. Ann Oncol. 2015; 26:731–736.


68. Misale S, Yaeger R, Hobor S, Scala E, Janakiraman M, Liska D, et al. Emergence of KRAS mutations and acquired resistance to anti-EGFR therapy in colorectal cancer. Nature. 2012; 486:532–536.


69. Yu HA, Arcila ME, Rekhtman N, Sima CS, Zakowski MF, Pao W, et al. Analysis of tumor specimens at the time of acquired resistance to EGFR-TKI therapy in 155 patients with EGFR-mutant lung cancers. Clin Cancer Res. 2013; 19:2240–2247.


70. Press Announcements - FDA approves new pill to treat certain patients with non-small cell lung cancer [Internet]. U S Food and Drug Administration Home Page. Updated on Nov 2015. https://www.fda.gov/NewsEvents/Newsroom/PressAnnouncements/ucm472525.htm.
71. Jänne PA, Yang JC, Kim DW, Planchard D, Ohe Y, Ramalingam SS, et al. AZD9291 in EGFR inhibitor-resistant non-small-cell lung cancer. N Engl J Med. 2015; 372:1689–1699.


72. Sequist LV, Soria JC, Goldman JW, Wakelee HA, Gadgeel SM, Varga A, et al. Rociletinib in EGFR-mutated non–small-cell lung cancer. N Engl J Med. 2015; 372:1700–1709.


73. Guibert N, Pradines A, Farella M, Casanova A, Gouin S, Keller L, et al. Monitoring KRAS mutations in circulating DNA and tumor cells using digital droplet PCR during treatment of KRAS-mutated lung adenocarcinoma. Lung Cancer. 2016; 100:1–4.
74. Guibert N, Pradines A, Casanova A, Farella M, Keller L, Soria JC, et al. Detection and monitoring of the BRAF mutation in circulating tumor cells and circulating tumor DNA in BRAF-mutated lung adenocarcinoma. J Thorac Oncol. 2016; 11:e109–e112.
75. Gray ES, Rizos H, Reid AL, Boyd SC, Pereira MR, Lo J, et al. Circulating tumor DNA to monitor treatment response and detect acquired resistance in patients with metastatic melanoma. Oncotarget. 2015; 6:42008–42018.


76. Bidard FC, Madic J, Mariani P, Piperno-Neumann S, Rampanou A, Servois V, et al. Detection rate and prognostic value of circulating tumor cells and circulating tumor DNA in metastatic uveal melanoma. Int J Cancer. 2013; 134:1207–1213.


77. Paweletz CP, Oxnard GR, Feeney N, Hilton JF, Gandhi L, Do KT, et al. Serial droplet digital PCR (ddPCR) of plasma cell-free DNA (cfDNA) as pharmacodynamic (PD) biomarker in Phase 1 clinical trials for patients (pts) with KRAS mutant non-small cell lung cancer (NSCLC). In : American Association for Cancer Research Annual Meeting in 2016;