Abstract
Matrix-assisted laser desorption ionization time-of-flight mass spectrometry (MALDI-TOF MS) has revolutionized the identification of microbial species in clinical microbiology laboratories. MALDI-TOF-MS has swiftly become the new gold-standard method owing to its key advantages of simplicity and robustness. However, as with all new methods, adoption of the MALDI-TOF MS approach is still not widespread. Optimal sample preparation has not yet been achieved for several applications, and there are continuing discussions on the need for improved database quality and the inclusion of additional microbial species. New applications such as in the field of antimicrobial susceptibility testing have been proposed but not yet translated to the level of ease and reproducibility that one should expect in routine diagnostic systems. Finally, during routine identification testing, unexpected results are regularly obtained, and the best methods for transmitting these results into clinical care are still evolving. We here discuss the success of MALDI-TOF MS in clinical microbiology and highlight fields of application that are still amenable to improvement.
The current position of matrix-assisted laser desorption ionization time-of-flight mass spectrometry (MALDI-TOF MS) in clinical microbiology is one of undisputed superiority over all other means of microbial species identification. At present, MALDI-TOF MS is considered the holy grail of rapid microbial identification despite only being introduced for use in routine laboratories less than 10 yr ago. The diagnostic applications cover gram-positive, gram-negative, aerobic, and anaerobic bacteria, as well as mycobacteria, yeasts, and molds. The specificity of MALDI-TOF MS-mediated microbial identification clearly makes this technique the current gold-standard diagnostic method. However, as has been claimed before, there is no single diagnostic method in microbiology that shows 100% accuracy [1]. In addition, even with the most efficient method, species identification is in the best case only as good as the current state of formal polyphasic microbial taxonomy [2]. Given this situation, it is important to consider aspects of the MALDI-TOF MS approach that can be further improved and to identify the issues that clinical microbiologists encounter when considering adopting this technology. The key remaining question is whether current discrepancies between results obtained through conventional methods and the outcomes of MALDI-TOF MS can be better explained when developing guidelines for new diagnostic and clinical protocols.
Here, we critically assess the success of MALDI-TOF MS in clinical microbiology. Whereas the important advantages of this technology have already been highlighted, we feel that it is equally important to define the current shortcomings and needs for improvement as well.
The primary application of MALDI-TOF MS in a clinical laboratory is species identification of cultured isolates of bacteria and fungi. Typically, isolated colonies of microbes grown on a solid agar medium are prepared by direct deposition of a small amount of cell material onto the target plate (e.g., for non acid-fast bacteria). For yeasts, and gram-positive cocci in some cases, on-target extraction with formic acid is applied to improve extraction. For molds and acid-fast bacteria, a multi-step extraction procedure in a reaction tube generally improves the spectrum quality and hence identification performance. Most importantly, the various extraction procedures are not competitive. That is, in the case where a direct deposit does not yield an identification result, an on-target or tube extraction method likely improves the spectrum quality without increasing the risk of obtaining false-positive results.
All extraction procedures ensure sufficient lysis of the cells and release of proteins that form a crystalline lattice with a chemical matrix, e.g., α-cyano-4-hydroxy-cinnamic acid (CHCA), enabling efficient ionization. [3]. Typical sample preparation methods for particular types of samples are outlined in Fig. 1. Other common methods involve growth in a liquid medium such as cultivation of mycoplasma in a broth from which the bacteria are subsequently concentrated and, if necessary, washed before mass spectrum acquisition and subsequent identification.
A particular sample preparation protocol is required for highly pathogenic species (e.g., tuberculous mycobacteria and other organisms requiring biological safety level [BSL] 3 conditions). Since MALDI-TOF MS systems are not typically found in BSL3 laboratories, any suspected BSL3 sample that is to be analyzed must be completely inactivated before being handled at a lower safety level [4]. The challenge for such an inactivation procedure is to eliminate viability, while retaining the proteins largely intact to allow for MALDI-TOF MS-based identification. Several procedures have been suggested to this end [567] and applied with success [8]. The most important steps for inactivation include the use of organic solvents (70% ethanol, trifluoroacetic acid, and various other mixtures), mechanical lysis, and washing steps. All procedures include multiple steps that are performed in a reaction tube that can be eventually transferred outside the BSL3 area. Although these procedures are technically straightforward, the most critical point is accurate handling by the laboratory staff to avoid the risk of contamination.
At present, MALDI-TOF MS cannot yet be performed directly on clinical samples in most cases, since the relatively low number of microorganisms present in the sample does not allow for accurate spectra acquisition. However, after enrichment during a liquid culture phase, identification becomes possible. For positive blood cultures, the microbial biomass is generally sufficient but needs to be concentrated and purified prior to mass spectral analysis. This can be achieved by a lysis/centrifugation or a lysis/filtration protocol yielding pure microbial pellets that can be treated like colonies grown on a solid medium [8910]. Other methods have been developed for this purpose, including quick-spin and wash protocols, density (gradient) centrifugation, among others. As an alternative to these concentration protocols, a so-called “short incubation” method has been applied with success by spreading an aliquot of a positive blood culture on an agar plate and incubating for 4–6 hr followed by sample preparation [1112]. Although this method extends the time of obtaining a result for individual samples, the overall efficiency is similar to that of more direct methods, mainly because the hands-on time is short [13]. Most importantly, with MALDI-TOF MS, a first tentative identification result can be reported at a significantly earlier time point, thereby considerably improving sepsis treatment [1415].
In general, and for most microbial species, approximately 104 cells per sample is the minimum biomass required to yield a spectrum of sufficient quality for identification [16]. Hence, only urine samples from urethritis patients are amenable to direct MALDI-TOF MS without an incubation step, as infected urine samples contain sufficient numbers of bacteria (>103 cells/mL) that can be concentrated from larger volumes (>10 mL). However, direct identification from urine is not always easy owing to the presence of potentially obscuring defensin peaks that can interfere with the identification [17]. Working directly with clinical samples can also be impaired by the fact that samples may be contaminated or contain more than one microorganism, which will compromise the identification results.
Without a doubt, MALDI-TOF MS provides a very strong methodology for microbial species identification with accuracies and specificities that range between 90% and close to 100% depending upon the microbial species involved or the spectrum of clinical samples analyzed. This level of performance often results in the reporting of rare species that may have not been previously reported at the species level [18]. For example, joint infections with Staphylococcus lugdunensis seem to be detected more often with MALDI-TOF MS than with conventional identification procedures [19].
Identification of isolates that are considered to be “difficult-to-identify microbes” with conventional procedures is generally not problematic with MALDI-TOF MS and depends primarily on the integrity of the database [20]. The reason is simply that all microorganisms produce housekeeping proteins such as ribosomal proteins, irrespective of their metabolic activity, that allow for mass spectral identification [21].
The question is then to determine the sort of activities carried out in a clinical laboratory that might generate unexpected findings, and the implications for laboratory workflow, clinical reporting, and patient treatment. In particular, it is important to establish whether a surprising result can be generally accepted or indicate the need for additional testing, e.g., ribosomal PCRs and sequencing of the PCR products. The problem is usually not that significant with the acceptance that a rare species or species not known to cause certain infections can be identified by MALDI-TOF MS; in general, a relatively short period of familiarization with the technology leads to a high acceptance level. The question rather is how to treat such infections. Obviously, this necessitates the development of new experimental and possibly clinically validated tools for antimicrobial susceptibility testing (AST), which requires time and research effort. A clear protocol for such cases has not yet been established, but could include a literature research (Has the species involved been described occasionally or never? Has treatment been proposed? Has treatment been documented and, most importantly, was such treatment successful?) and growth-based AST, including disc diffusion testing or antibiotic gradient testing with a broad spectrum of antibiotic compounds. Of course, such testing would initially be conducted for research purposes only, but could be used to at least guide clinical treatment. Such treatment should obviously be supervised clinically, and disease severity should be included in the decision tree. Although no standard method of dealing with unexpected species identifications has been presented to date, the phenomenon as such is expected to increase in frequency as databases become increasingly more exhaustive. Deciphering complexes into specific species will generate different perspectives of epidemiological behavior (e.g., the detection of Enterobacter hormaechei or individual members of the Acinetobacter baumannii complex will change the assessment of the spread of the respective species).
Another aspect of MALDI-TOF MS-based identification is the ease and relatively low cost of a single identification result, which permits the selection of multiple if not all colonies from agar plates. This will lead to a widened view of infectious and commensal microorganisms and, possibly, to the recognition of new pathogens or specific disease-related microbial consortia.
For the vast majority of species encountered in a routine clinical microbiology laboratory, MALDI-TOF MS databases are fairly comprehensive and robust. Therefore, on the one hand, it can surely be stated that available commercial databases are sufficiently complete to allow for efficient, routine identification in medical and, with some reservations, veterinary microbiology. This has been demonstrated in a large number of studies on various sample types. On the other hand, the number of known and well described microbial species only represents a tiny fraction of the global microbial diversity, and therefore available databases can never be complete in a strict sense. This is most evident for environmental microbiology and to a lesser degree for veterinary microbiology. In clinical microbiology, the knowledge of species associated with the human body has reached a high level but is nonetheless still far from being complete, as highlighted by molecular studies on the human microbiome [2223].
As we learn more about cryptic species or species that were previously confused with more common ones, it becomes increasingly important to delineate and expand databases, even if that would lead to the perseverance of some incorrect reference data. For example, the group previously thought of as the single species Candida haemulonii is now known to be a complex of multiple species, at least three of which (C. auris, C. duobushaemulonii, and C. haemulonii) are regularly detected in clinical settings [24].
Similar cases have been observed with species of Aspergillus [25], Burkholderia [26], Elizabethkingia [27], Klebsiella [28], and others. In these genera, new species have been described in recent years, and their identification is considered to be of medical relevance. For example, in the genus Acinetobacter, some species comprising the Acinetobacter-calcoaceticus-baumannii-complex (ACB) have been described only recently [29], but isolates belonging to these species were encountered prior to the valid description of the respective species, including A. pittii as “DNA group 3”, A. nosocomialis as “DNA group 13TU”, or A. seifertii as “close to DNA group 13TU” [30].
Naturally, any identification system can only report a species name when the corresponding species has been validly described at an undisputed taxonomic level. For an isolate that belongs to a species for which only an informal description has been accomplished, identification is ideally achieved at a higher taxonomic level, generally the genus or complex level. By gradual amendment of reference databases, new species may eventually be correctly identified; however, in addition to the proteomic data, this will require development of a well-defined “taxogenomic” framework in order to keep track of such new species. It is likely that microbial taxonomy will be primarily based on genome sequences in the future, a tendency that will also likely co-define the clinical utility of MALDI-TOF MS [3132].
The discovery of new species and taxonomic revisions is occurring at a much higher frequency today compared to a few decades ago, primarily owing to the increasing availability and wide application of molecular (genomic) methods. In consequence, reference databases need to be updated continuously to account for new species or new species names. For example, as of October 16, 2016, the genus Mycobacterium comprises 192 species and subspecies, 63 of which have only been described between 2000 and 2009 and 35 were noted from 2010 onwards. Of course, not all newly described species are of clinical relevance and many have thus far only been found in environmental samples. However, new species isolated from clinical samples are of potential medical importance. In Mycobacterium, this may be the case for the species M. alsense [33], M. celeriflavum [34], and M. saopaulense [35], all of which have been isolated from patient samples but have not yet been included in commercial databases.
Although the process of database amendment or revision is technically straightforward, similar to that for public nucleotide sequence databases, the procedure is much more complex on the clinical side. For providers of commercial in vitro diagnosis (IVD) databases, the first step is to decide which of the new validly described species are of sufficient clinical relevance to be included in the databases. In addition, it can be a challenge to find a sufficient number of strains available to build the database extension. Clinical validation requiring multiple unique isolates may be cumbersome if the frequency of occurrence of particular species is (very) low.
Important guidance is derived from scientific monitoring and user feedback, i.e., information on the occurrence of isolates in clinical samples that could not be identified or were misidentified by MALDI-TOF MS. Amended databases then need to be subjected to thorough testing, for example in clinical trials, before being released as a diagnostic tool. To date, the US Food and Drug Administration (FDA) has judged that a species can only be claimed usable for IVD in an approved database, if enough data are collected during the clinical trials, generally requiring tests on 30 unique isolates. The latter is true when there is an abundance of isolates; otherwise, the US FDA will agree to accept replicates of more limited numbers of isolates. If there is extreme rarity, the US FDA would agree to 30 replicates of one strain but their preference is to conduct tests with at least three strains along with respective replicate tests to achieve a minimum of 30 results.
Although identification of microorganisms can be efficiently achieved by MALDI-TOF MS, the method of choice for subsequent AST is under debate. Complementary to classical techniques for AST, several MS-based methods for the rapid determination of resistance or susceptibility have been suggested. These can be categorized in four major types.
Ideally, the spectrum used for identification is then used for typing. A prerequisite for this approach is that proteins conferring resistance or related proteins fall within the mass range used for WCMS. For example, in some methicillin-resistant Staphylococcus aureus (MRSA) strains, methicillin resistance is associated with the presence of a phenol-soluble modulin (Psm) at m/z 2,415 Da [36]. This peptide is encoded by some of the staphylococcal cassette chromosome mec (SCCmec) types and is easily detectable, whereas penicillin-binding protein 2a (PBP2A) that actually confers resistance has a molecular weight of about 76 kDa, and is therefore not detectable. Detection of an intense peak at m/z 2,415 indicates methicillin resistance, although its absence unfortunately does not allow for a conclusion of susceptibility to be reached [37]. If a particular resistance mechanism is associated with a particular phylotype, WCMS may be used to predict resistance. For example, Bacteroides fragilis strains can be distinguished according to their cfiA status without directly detecting the metallo-β-lactamase itself [3839].
In this method, bacteria are exposed to antibiotics and then spotted on the MALDI-TOF MS slide in the presence of an internal standard [40]. This allows for the interpretation of resulting mass spectra in a quantitative manner by relating protein peaks to the internal standard peak and reaching conclusions based on the patterns of growth inhibition [41]. In principle, this approach can be applied to all antibiotics after optimization of test conditions, but multiplexing is not possible and each antibiotic has to be tested separately. In a related approach, the (lack of) de novo biosynthesis of proteins in the presence of antibiotics is detected through the incorporation of amino acids labeled with stable isotopes with a resulting mass shift of protein peaks [42].
This approach uses MALDI-TOF MS as an analytical tool to detect antibiotics and their modifications through analysis of enzymatic activities [43]. Hence, enzymes such as carbapenemases or lactamases in general are not directly detected—although this has been suggested in some publications—but their presence in a particular strain can be concluded from identification of a modification of an antibiotic that supposedly occurs through enzymatic activity. This technique has been widely used for the detection of carbapenemase activity through the observation of a mass shift due to hydrolysis of the lactam moiety of carbapenems, sometimes followed by decarboxylation, resulting in mass shifts of +18 Da or −26 Da, respectively, compared to the native antibiotic's mass [444546] for a number of species as well as variants of lactamases [474849]. For the latter case, it is important to note that some variants show low enzymatic activity and hence require an extended incubation time (4 hr) to avoid false-negative results [4950]. Further, the assay has been applied to samples obtained from positive blood culture, potentially avoiding a cultivation step using solid growth media [5152]. Multiplexing, i.e., an assay with multiple antibiotics, may be feasible when lysates of bacteria are used [45]. Although MALDI-TOF MS has been used in most of the studies in this field, other mass spectrometric approaches such as liquid chromatography (LC)-MS/MS show comparable performances [5354].
Proteins that are not accessible for direct detection by WCMS can generally be detected by MS/MS approaches, often referred to as top-down proteomics. From a sample such as a bacterial colony, proteins are extracted and subjected to tryptic digestion. The resulting mixture of a large number of peptides is analyzed by LC-MS/MS to identify specific peptides based on their parent ion mass and fragmentation patterns. These so-called proteotypic peptides indicate the presence of resistance-conferring proteins such as PBP2a or extended-spectrum β-lactamase with high sensitivity and specificity [55565758].
While a considerable number of studies have demonstrated the promise of MS for AST or resistance testing, no method proposed to date has yet been validated by clinical trials and fully approved by regulatory agencies [59]. For full adaptation of the carbapenemase activity monitoring assay, for example, standardization is required to achieve reproducible and reliable results [6061]. More globally, it can be stated that MS assays targeting the detection of resistance cannot replace AST, simply because the results do not allow for the selection of a drug for treatment but can rather only provide information as to which drugs should not be used. Therefore, the value of MS resistance testing may be primarily for epidemiological analysis of selected isolates, while conventional AST must be applied for the bulk of isolates.
As mentioned above, the limited sensitivity of whole-cell MALDI-TOF MS makes the direct analysis of clinical samples difficult. Improving the sensitivity of MALDI-TOF MS by concentration and miniaturization steps is feasible to some extent and would be much appreciated by current users, but this can only be achieved at the price of increased handling time and equipment costs. In principle, the sensitivity can be increased for single bacterial cells, as has been shown for on-line aerosol MS [62]. However, for reliable identification, most likely a minimum of approximately 100 cells free of organic or inorganic matrix can be reasonably assumed. If this number of cells can be isolated from a clinical sample, for example through immuno-capture techniques [63], identification may indeed be feasible [64].
With the currently available commercial systems, a few but important limitations of species identification remain to be resolved. Although the differentiation of Shigella spp. and Escherichia coli has been suggested [6566], this test has not yet been validated or approved. The reason for this limitation is simply the fact that Shigella and E. coli are considered as a single species by taxonomists [67] and hence have highly similar proteomic patterns.
Species of the Mycobacterium tuberculosis complex (MTB) exhibit the same level of similarity. Although identification as a member of the MTB can generally be achieved with high sensitivity and specificity [6869], more precise identification to the species level is not feasible at present.
These and further limitations have to be accepted for now, and some problems may prove (very) hard to be solved with whole-cell MALDI-TOF MS. Consequently, for the time being, microbiological expertise as well as additional, specific test systems need to be conserved to deal with tests, in which MALDI-TOF MS results are inconclusive. Finally, it goes without saying that all microbiologists would like to see a smaller tool, available at a reduced price, with better qualifications and a universal database while simultaneously performing high-quality AST.
Within a few years, MALDI-TOF MS identification has been implemented in a large number of laboratories, replacing conventional automated identification systems. This process has been largely facilitated by the fact that much less sample material is needed for MALDI-TOF MS compared to identification with classical substrate galleries or cards [70].
The costs of MALDI-TOF MS-based identification in comparison to conventional methods for routine clinical diagnostics have been evaluated in a few studies [717273]. Expenses per sample as well as the time-to-result could be considerably reduced with MALDI-TOF MS although the numbers vary from study to study. Thus, MALDI-TOF MS identification systems can be considered as cheaper, faster, and more accurate than conventional identification systems.
How then are the identification and putative AST results ultimately translated into practice? Are there accepted protocols for streamlining this flow of information from the laboratory to the clinician who is treating patients with infectious disease? For routine microbial diagnostics, reliable identification of an isolate is only one step in the workflow. The most important result to be communicated to a clinician obviously relates to treatment; which are the antibiotics that can be applied, at which doses, and for what duration? Hence, identification of a microbial species facilitates the initiation of the most important AST. It is in this dynamic situation where MALDI-TOF MS allows for true acceleration. For example, whereas identification used to take at least 24 hr, this can now be achieved in only a very small fraction of that time. It must be emphasized, however, that there is still a clear disconnect between the current rapidity in the field of microbial identification and the time required for performing AST. While MALDI-TOF MS has solved one bottleneck, the AST-related issues remain very tangible.
MALDI-TOF MS-based identification is generally achieved at the species level or, for a small percentage of isolates, at the genus level. Identification at higher taxonomic levels is not possible, such as the classification of isolates as Bacteroidetes or Alphaproteobacteria as is frequently done for sequence-characterized isolates that do not belong to a known genus. This means that the primary result to be reported is a species name that is potentially familiar neither to clinicians nor to general practitioners. This applies to rare, new, or renamed species. Hence, a microbiological report containing an identification result for an unfamiliar species should include a ‘translation’ to more commonly used terminology to avoid confusion on the treatment side. This is true for rare or uncommon species as well as for species that have been subject to recent taxonomic revision, e.g., Lelliottia amnigena (syn. Enterobacter amnigenus), Saprochaete capitata (syn. Geotrichum capitatum, syn. Blastoschizomyces capitatus, syn. Trichosporon capitatum, etc.). For these and similar cases, the diagnostic result provided by the identification system is ideally complemented with taxonomic information for the treating physician. This could be simply the (earlier) synonym or a hint on the wider taxonomic affiliation of unusual species. For example, the latter could be a hint that species such as Staphylococcus auricularis, S. capitis, and S. pettenkoferi belong to the S. epidermidis-group of coagulase-negative staphylococci (CoNS). CoNS are part of the human skin microbiome and are generally of lower pathogenicity, but have been reported as etiological agents of infectious disease in particular cases [74].
Innovative MS methods are already being considered for introduction into clinical laboratories. All classical MALDI-TOF MS machines could be improved with respect to the need of calibration, size, maintenance levels, and cost. In addition, there are MS methods available that allow for the more detailed identification and characterization of microbial strains, including the detection of resistance genes and virulence factors as well as epidemiological markers [57]. To date, these methods require expensive equipment (three to four times more expensive than classical MALDI-TOF MS systems) and it is only possible to analyze one sample at a time. Since such analyses take longer to complete, at present, these methods are too slow for inclusion in the high-throughput workflow of a clinical microbiology laboratory. However, as with any technology, improvements are foreseen in the years to come.
In conclusion, MALDI-TOF MS dominates the field of microbial identification at this stage. New developments, both technological and practical (with respect to the clinical databases), are foreseen that will further broaden the acceptance and integration of the MS technology in clinical microbiology. Nevertheless, competing technologies are emerging in parallel. In particular, the “omics” technologies, including next-generation microbial genome sequencing, will be competing for prime positions in the clinical microbiological diagnostic workflow. Therefore, the issue as to whether MALDI-TOF MS-based methods or those more geared to other MS technologies will ultimately survive and predominate in the field of clinical microbiology will undoubtedly become increasingly clarified over the next five years.
Acknowledgments
We gratefully acknowledge all of our colleagues who participated in the wide variety of aspects of the work around MALDI TOF MS.
References
1. van Belkum A, Welker M, Dunne WM Jr, Girard V. The infallible microbial identification test: does it exist? J Clin Microbiol. 2015; 53:1786. PMID: 25883325.
2. Vandamme P, Peeters C. Time to revisit polyphasic taxonomy. Antonie Van Leeuwenhoek. 2014; 106:57–65. PMID: 24633913.
3. Šedo O, Sedláček I, Zdráhal Z. Sample preparation methods for MALDI-MS profiling of bacteria. Mass Spectrom Rev. 2011; 30:417–434. PMID: 21500244.
4. Lasch P, Jacob D, Grunow R, Schwecke T, Doellinger J. Matrix-assisted laser desorption/ionization time-of-flight (MALDI-TOF) mass spectrometry (MS) for the identification of highly pathogenic bacteria. Trends Analyt Chem. 2016; 85:103–111.
5. Dunne WM Jr, Doing K, Miller E, Miller E, Moreno E, Baghli M, et al. Rapid inactivation of Mycobacterium and Nocardia species before identification using matrix-assisted laser desorption ionization-time of flight mass spectrometry. J Clin Microbiol. 2014; 52:3654–3659. PMID: 25078917.
6. Mesureur J, Ranaldi S, Monnin V, Girard V, Arend S, Welker M, et al. A simple and safe protocol for preparing Brucella samples for matrix-assisted laser desorption/ionization-time of flight mass spectrometry analysis. J Clin Microbiol. 2016; 54:449–452. PMID: 26582837.
7. Lasch P, Nattermann H, Erhard M, Stämmler M, Grunow R, Bannert N, et al. MALDI-TOF mass spectrometry compatible inactivation method for highly pathogenic microbial cells and spores. Anal Chem. 2008; 80:2026–2034. PMID: 18290666.
8. Fothergill A, Kasinathan V, Hyman J, Walsh J, Drake T, Wang YF. Rapid identification of bacteria and yeasts from positive-blood-culture bottles by using a lysis-filtration method and matrix-assisted laser desorption ionization-time of flight mass spectrum analysis with the SARAMIS database. J Clin Microbiol. 2013; 51:805–809. PMID: 23254131.
9. Nonnemann B, Tvede M, Bjarnsholt T. Identification of pathogenic microorganisms directly from positive blood vials by matrix-assisted laser desorption/ionization time of flight mass spectrometry. Apmis. 2013; 121:871–877. PMID: 23331371.
10. Leli C, Cenci E, Cardaccia A, Moretti A, D'Alò F, Pagliochini R, et al. Rapid identification of bacterial and fungal pathogens from positive blood cultures by MALDI-TOF MS. Int J Med Microbiol. 2013; 303:205–209. PMID: 23602511.
11. Köck R, Wüllenweber J, Horn D, Lanckohr C, Becker K, Idelevich EA. Implementation of short incubation MALDI-TOF MS identification from positive blood cultures in routine diagnostics and effects on empiric antimicrobial therapy. Antimicrob Resist Infect Control. 2017; 6:12. PMID: 28101334.
12. Altun O, Botero-Kleiven S, Carlsson S, Ullberg M, Özenci V. Rapid identification of bacteria from positive blood culture bottles by MALDI-TOF MS following short-term incubation on solid media. J Med Microbiol. 2015; 64:1346–1352. PMID: 26361761.
13. Curtoni A, Cipriani R, Marra ES, Barbui AM, Cavallo R, Costa C. Rapid identification of microorganisms from positive blood culture by MALDI-TOF MS after short-term incubation on solid medium. Curr Microbiol. 2017; 74:97–102. PMID: 27858149.
14. Angeletti S, Dicuonzo G, D'Agostino A, Avola A, Crea F, Palazzo C, et al. Turnaround time of positive blood cultures after the introduction of matrix-assisted laser desorption-ionization time-of-flight mass spectrometry. New Microbiol. 2015; 38:379–386. PMID: 26147149.
15. Schneiderhan W, Grundt A, Wörner S, Findeisen P, Neumaier M. Work flow analysis of around-the-clock processing of blood culture samples and integrated MALDI-TOF mass spectrometry analysis for the diagnosis of bloodstream infections. Clin Chem. 2013; 59:1649–1656. PMID: 23881934.
16. Hsieh SY, Tseng CL, Lee YS, Kuo AJ, Sun CF, Lin YH, et al. Highly efficient classification and identification of human pathogenic bacteria by MALDI-TOF MS. Mol Cell Proteomics. 2008; 7:448–456. PMID: 18045801.
17. Köhling HL, Bittner A, Müller KD, Buer J, Becker M, Rübben H, et al. Direct identification of bacteria in urine samples by matrix-assisted laser desorption/ionization time-of-flight mass spectrometry and relevance of defensins as interfering factors. J Med Microbiol. 2012; 61:339–344. PMID: 22275503.
18. Seng P, Abat C, Rolain JM, Colson P, Lagier JC, Gouriet F, et al. Identification of rare pathogenic bacteria in a clinical microbiology laboratory: impact of matrix-assisted laser desorption ionization-time of flight mass spectrometry. J Clin Microbiol. 2013; 51:2182–2194. PMID: 23637301.
19. Szabados F, Anders A, Kaase M, Marlinghaus L, Gatermann SG, Teske W, et al. Late periprosthetic joint infection due to Staphylococcus lugdunensis identified by Matrix-Assisted Laser Desorption/Ionisation Time of Flight Mass Spectrometry: a case report and review of the literature. Case Rep Med. 2011; 2011:608919. PMID: 21776276.
20. Rodríguez-Sánchez B, Marín M, Sánchez-Carrillo C, Cercenado E, Ruiz A, Rodríguez-Créixems M, et al. Improvement of matrix-assisted laser desorption/ionization time-of-flight mass spectrometry identification of difficult-to-identify bacteria and its impact in the workflow of a clinical microbiology laboratory. Diagn Microbiol Infect Dis. 2014; 79:1–6. PMID: 24602850.
21. Welker M, Moore ER. Applications of whole-cell matrix-assisted laser-desorption/ionization time-of-flight mass spectrometry in systematic microbiology. Syst Appl Microbiol. 2011; 34:2–11. PMID: 21288677.
22. Lagier JC, Khelaifia S, Alou MT, Ndongo S, Dione N, Hugon P, et al. Culture of previously uncultured members of the human gut microbiota by culturomics. Nat Microbiol. 2016; 1:16203. PMID: 27819657.
23. Rajilić-Stojanović M, de Vos WM. The first 1000 cultured species of the human gastrointestinal microbiota. FEMS Microbiol Rev. 2014; 38:996–1047. PMID: 24861948.
24. Grenfell RC, da Silva Junior AR, Del Negro GM, Munhoz RB, Gimenes VM, Assis DM, et al. Identification of Candida haemulonii complex species: Use of ClinProTools(TM) to overcome limitations of the Bruker Biotyper(TM), VITEK MS(TM) IVD, and VITEK MS(TM) RUO Databases. Front Microbiol. 2016; 7:940. PMID: 27379069.
25. Lamoth F. Aspergillus fumigatus-related species in clinical practice. Front Microbiol. 2016; 7:683. PMID: 27242710.
26. Lambiase A, Del Pezzo M, Cerbone D, Raia V, Rossano F, Catania MR. Rapid identification of Burkholderia cepacia complex species recovered from cystic fibrosis patients using matrix-assisted laser desorption ionization time-of-flight mass spectrometry. J Microbiol Methods. 2013; 92:145–149. PMID: 23201483.
27. Han MS, Kim H, Lee Y, Kim M, Ku NS, Choi JY, et al. Relative prevalence and antimicrobial susceptibility of clinical isolates of Elizabethkingia species based on 16S rRNA gene sequencing. J Clin Microbiol. 2016; 55:274–280. PMID: 27847376.
28. Fonseca EL, Ramos ND, Andrade BG, Morais LL, Marin MF, Vicente AC. A one-step multiplex PCR to identify Klebsiella pneumoniae, Klebsiella variicola, and Klebsiella quasipneumoniae in the clinical routine. Diagn Microbiol Infect Dis. 2017; 87:315–317. PMID: 28139276.
29. Teixeira AB, Barin J, Hermes DM, Barth AL, Martins AF. PCR assay based on the gyrB gene for rapid identification of Acinetobacter baumannii-calcoaceticus complex at species level. J Clin Lab Anal. 2017; 31:e22046.
30. Marí-Almirall M, Cosgaya C, Higgins PG, Van Assche A, Telli M, Huys G, et al. MALDI-TOF/MS identification of species from the Acinetobacter baumannii (Ab) group revisited: inclusion of the novel A. seifertii and A. dijkshoorniae species. Clin Microbiol Infect. 2017; 23:210. PMID: 27919649.
31. Rosselló-Móra R, Amann R. Past and future species definitions for Bacteria and Archaea. Syst Appl Microbiol. 2015; 38:209–216. PMID: 25747618.
32. Whitman WB. Genome sequences as the type material for taxonomic descriptions of prokaryotes. Syst Appl Microbiol. 2015; 38:217–222. PMID: 25769508.
33. Tortoli E, Richter E, Borroni E, Cabibbe AM, Capitolo E, Cittaro D, et al. Mycobacterium alsense sp. nov., a scotochromogenic slow grower isolated from clinical respiratory specimens. Int J Syst Evol Microbiol. 2016; 66:450–456. PMID: 26545358.
34. Shahraki AH, Çavusoğlu C, Borroni E, Heidarieh P, Koksalan OK, Cabibbe AM, et al. Mycobacterium celeriflavum sp. nov., a rapidly growing scotochromogenic bacterium isolated from clinical specimens. Int J Syst Evol Microbiol. 2015; 65:510–515. PMID: 25389151.
35. Nogueira CL, Whipps CM, Matsumoto CK, Chimara E, Droz S, Tortoli E, et al. Mycobacterium saopaulense sp. nov., a rapidly growing mycobacterium closely related to members of the Mycobacterium chelonae--Mycobacterium abscessus group. Int J Syst Evol Microbiol. 2015; 65:4403–4409. PMID: 26358475.
36. Josten M, Dischinger J, Szekat C, Reif M, Al-Sabti N, Sahl HG, et al. Identification of agr-positive methicillin-resistant Staphylococcus aureus harbouring the class A mec complex by MALDI-TOF mass spectrometry. Int J Med Microbiol. 2014; 304:1018–1023. PMID: 25116838.
37. Rhoads DD, Wang H, Karichu J, Richter SS. The presence of a single MALDI-TOF mass spectral peak predicts methicillin resistance in staphylococci. Diagn Microbiol Infect Dis. 2016; 86:257–261. PMID: 27568365.
38. Fenyvesi VS, Urbán E, Bartha N, Abrók M, Kostrzewa M, Nagy E, et al. Use of MALDI-TOF/MS for routine detection of cfiA gene-positive Bacteroides fragilis strains. Int J Antimicrob Agents. 2014; 44:474–475. PMID: 25216544.
39. Nagy E, Becker S, Sóki J, Urbán E, Kostrzewa M. Differentiation of division I (cfiA-negative) and division II (cfiA-positive) Bacteroides fragilis strains by matrix-assisted laser desorption/ionization time-of-flight mass spectrometry. J Med Microbiol. 2011; 60:1584–1590. PMID: 21680764.
40. Sparbier K, Schubert S, Kostrzewa M. MBT-ASTRA: A suitable tool for fast antibiotic susceptibility testing? Methods. 2016; 104:48–54. PMID: 26804565.
41. Jung JS, Hamacher C, Gross B, Sparbier K, Lange C, Kostrzewa M, et al. Evaluation of a semiquantitative matrix-assisted laser desorption ionization-time of flight mass spectrometry method for rapid antimicrobial susceptibility testing of positive blood cultures. J Clin Microbiol. 2016; 54:2820–2824. PMID: 27629893.
42. Sparbier K, Lange C, Jung J, Wieser A, Schubert S, Kostrzewa M. MALDI biotyper-based rapid resistance detection by stable-isotope labeling. J Clin Microbiol. 2013; 51:3741–3748. PMID: 24006001.
43. Wieser A, Schubert S. MALDI-TOF MS entering the microbiological diagnostic laboratory - from fast identification to resistance testing. TrAC Trends Anal Chem. 2016; 84:80–87.
44. Hrabák J, Walková R, Studentová V, Chudácková E, Bergerová T. Carbapenemase activity detection by matrix-assisted laser desorption ionization-time of flight mass spectrometry. J Clin Microbiol. 2011; 49:3222–3227. PMID: 21775535.
45. Hooff GP, van Kampen JJ, Meesters RJ, van Belkum A, Goessens WH, Luider TM. Characterization of β-lactamase enzyme activity in bacterial lysates using MALDI-mass spectrometry. J Proteome Res. 2012; 11:79–84. PMID: 22013912.
46. Sparbier K, Schubert S, Weller U, Boogen C, Kostrzewa M. Matrix-assisted laser desorption ionization-time of flight mass spectrometry-based functional assay for rapid detection of resistance against β-lactam antibiotics. J Clin Microbiol. 2012; 50:927–937. PMID: 22205812.
47. Hrabák J, Studentová V, Walková R, Zemlicková H, Jakubu V, Chudácková E, et al. Detection of NDM-1, VIM-1, KPC, OXA-48, and OXA-162 carbapenemases by matrix-assisted laser desorption ionization-time of flight mass spectrometry. J Clin Microbiol. 2012; 50:2441–2443. PMID: 22553235.
48. Knox J, Jadhav S, Sevior D, Agyekum A, Whipp M, Waring L, et al. Phenotypic detection of carbapenemase-producing Enterobacteriaceae by use of matrix-assisted laser desorption ionization-time of flight mass spectrometry and the Carba NP test. J Clin Microbiol. 2014; 52:4075–4077. PMID: 25187633.
49. Chong PM, McCorrister SJ, Unger MS, Boyd DA, Mulvey MR, Westmacott GR. MALDI-TOF MS detection of carbapenemase activity in clinical isolates of Enterobacteriaceae spp., Pseudomonas aeruginosa, and Acinetobacter baumannii compared against the Carba-NP assay. J Microbiol Methods. 2015; 111:21–23. PMID: 25644285.
50. Carvalhaes CG, da Silva AC, Streling AP, Cayô R, Rockstroh AC, Machado AM, et al. Detection of carbapenemase activity using VITEK MS: interplay of carbapenemase type and period of incubation. J Med Microbiol. 2015; 64:946–947. PMID: 26293667.
51. Carvalhaes CG, Cayô R, Visconde MF, Barone T, Frigatto EA, Okamoto D, et al. Detection of carbapenemase activity directly from blood culture vials using MALDI-TOF MS: a quick answer for the right decision. J Antimicrob Chemother. 2014; 69:2132–2136. PMID: 24722840.
52. Johansson Å, Nagy E, Sóki J. Instant screening and verification of carbapenemase activity in Bacteroides fragilis in positive blood culture, using matrix-assisted laser desorption ionization--time of flight mass spectrometry. J Med Microbiol. 2014; 63:1105–1110. PMID: 24850880.
53. Kulkarni MV, Zurita AN, Pyka JS, Murray TS, Hodsdon ME, Peaper DR. Use of imipenem to detect KPC, NDM, OXA, IMP, and VIM carbapenemase activity from gram-negative rods in 75 minutes using liquid chromatography-tandem mass spectrometry. J Clin Microbiol. 2014; 52:2500–2505. PMID: 24789180.
54. Peaper DR, Kulkarni MV, Tichy AN, Jarvis M, Murray TS, Hodsdon ME. Rapid detesction of carbapenemase activity through monitoring ertapenem hydrolysis in Enterobacteriaceae with LC-MS/MS. Bioanalysis. 2013; 5:147–157. PMID: 23330558.
55. Fleurbaaij F, Heemskerk AA, Russcher A, Klychnikov OI, Deelder AM, Mayboroda OA, et al. Capillary-electrophoresis mass spectrometry for the detection of carbapenemases in (multi-)drug-resistant Gram-negative bacteria. Anal Chem. 2014; 86:9154–9161. PMID: 25155175.
56. Hart PJ, Wey E, McHugh TD, Balakrishnan I, Belgacem O. A method for the detection of antibiotic resistance markers in clinical strains of Escherichia coli using MALDI mass spectrometry. J Microbiol Methods. 2015; 111:1–8. PMID: 25633625.
57. Charretier Y, Dauwalder O, Franceschi C, Degout-Charmette E, Zambardi G, Cecchini T, et al. Rapid bacterial identification, resistance, virulence and type profiling using selected reaction monitoring mass spectrometry. Sci Rep. 2015; 5:13944. PMID: 26350205.
58. Karlsson R, Gonzales-Siles L, Boulund F, Svensson-Stadler L, Skovbjerg S, Karlsson A, et al. Proteotyping: Proteomic characterization, classification and identification of microorganisms - A prospectus. Syst Appl Microbiol. 2015; 38:246–257. PMID: 25933927.
59. Aguirre-Quiñonero A, Martínez-Martínez L. Non-molecular detection of carbapenemases in Enterobacteriaceae clinical isolates. J Infect Chemother. 2017; 23:1–11. PMID: 27769646.
60. Monteferrante CG, Sultan S, Ten Kate MT, Dekker LJ, Sparbier K, Peer M, et al. Evaluation of different pretreatment protocols to detect accurately clinical carbapenemase-producing Enterobacteriaceae by MALDI-TOF. J Antimicrob Chemother. 2016; 71:2856–2867. PMID: 27287232.
61. Mirande C, Canard I, Buffet Croix, Charrier JP, van Belkum A, Welker M, et al. Rapid detection of carbapenemase activity: benefits and weaknesses of MALDI-TOF MS. Eur J Clin Microbiol Infect Dis. 2015; 34:2225–2234. PMID: 26337432.
62. van Wuijckhuijse AL, Stowers MA, Kleefsman WA, van Baar BLM, Kientz CE, Marijnissen JCM. Matrix-assisted laser desorption/ionisation aerosol time-of-flight mass spectrometry for the analysis of bioaerosols: development of a fast detector for airborne biological pathogens. J Aerosol Sci. 2005; 36:677–687.
63. Madonna AJ, Basile F, Furlong E, Voorhees KJ. Detection of bacteria from biological mixtures using immunomagnetic separation combined with matrix-assisted laser desorption/ionization time-of-flight mass spectrometry. Rapid Commun Mass Spectrom. 2001; 15:1068–1074. PMID: 11404843.
64. Ochoa ML, Harrington PB. Immunomagnetic isolation of enterohemorrhagic Escherichia coli O157:H7 from ground beef and identification by matrix-assisted laser desorption/ionization time-of-flight mass spectrometry and database searches. Anal Chem. 2005; 77:5258–5267. PMID: 16097767.
65. Khot PD, Fisher MA. Novel approach for differentiating Shigella species and Escherichia coli by matrix-assisted laser desorption ionization-time of flight mass spectrometry. J Clin Microbiol. 2013; 51:3711–3716. PMID: 23985919.
66. Paauw A, Jonker D, Roeselers G, Heng JM, Mars-Groenendijk RH, Trip H, et al. Rapid and reliable discrimination between Shigella species and Escherichia coli using MALDI-TOF mass spectrometry. Int J Med Microbiol. 2015; 305:446–452. PMID: 25912807.
67. Pupo GM, Lan R, Reeves PR. Multiple independent origins of Shigella clones of Escherichia coli and convergent evolution of many of their characteristics. Proc Natl Acad Sci U S A. 2000; 97:10567–10572. PMID: 10954745.
68. Buckwalter SP, Olson SL, Connelly BJ, Lucas BC, Rodning AA, Walchak RC, et al. Evaluation of matrix-assisted laser desorption ionization-time of flight mass spectrometry for identification of Mycobacterium species, Nocardia species, and other aerobic Actinomycetes. J Clin Microbiol. 2016; 54:376–384. PMID: 26637381.
69. Girard V, Mailler S, Welker M, Arsac M, Cellière B, Cotte-Pattat PJ, et al. Identification of mycobacterium spp. and nocardia spp. from solid and liquid cultures by matrix-assisted laser desorption ionization-time of flight mass spectrometry (MALDI-TOF MS). Diagn Microbiol Infect Dis. 2016; 86:277–283. PMID: 27567285.
70. Patel R. Matrix-assisted laser desorption ionization-time of flight mass spectrometry in clinical microbiology. Clin Infect Dis. 2013; 57:564–572. PMID: 23595835.
71. Tran A, Alby K, Kerr A, Jones M, Gilligan PH. Cost savings realized by implementation of routine microbiological identification by matrix-assisted laser desorption ionization-time of flight mass spectrometry. J Clin Microbiol. 2015; 53:2473–2479. PMID: 25994167.
72. Tan KE, Ellis BC, Lee R, Stamper PD, Zhang SX, Carroll KC. Prospective evaluation of a matrix-assisted laser desorption ionization-time of flight mass spectrometry system in a hospital clinical microbiology laboratory for identification of bacteria and yeasts: a bench-by-bench study for assessing the impact on time to identification and cost-effectiveness. J Clin Microbiol. 2012; 50:3301–3308. PMID: 22855510.
73. Neville SA, Lecordier A, Ziochos H, Chater MJ, Gosbell IB, Maley MW, et al. Utility of matrix-assisted laser desorption ionization-time of flight mass spectrometry following introduction for routine laboratory bacterial identification. J Clin Microbiol. 2011; 49:2980–2984. PMID: 21632894.
74. Becker K, Heilmann C, Peters G. Coagulase-negative staphylococci. Clin Microbiol Rev. 2014; 27:870–926. PMID: 25278577.
Fig. 1
Schematic summary of commonly applied sample preparation procedures for particular types of samples in matrix-assisted laser desorption ionization time-of-flight mass spectrometry (MALDI-TOF MS)-based microbial identification. See the text for details.
Abbreviation: BSL, biological safety level.
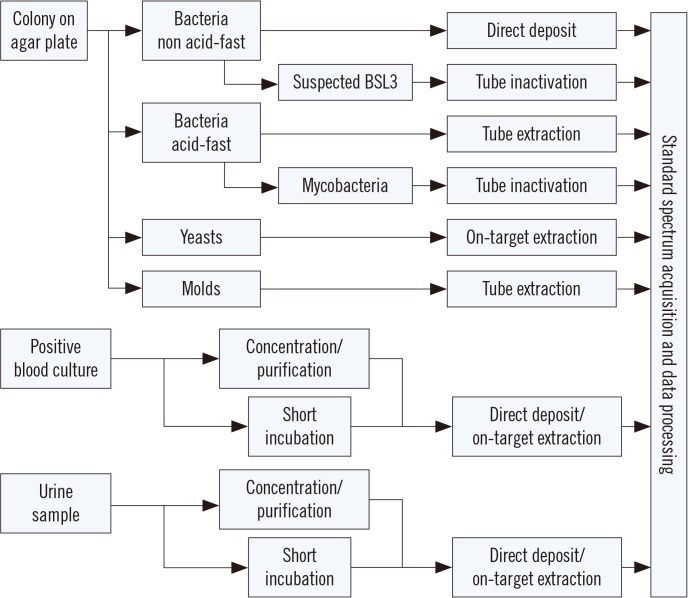