Abstract
Background
Streptococcus dysgalactiae subsp. equisimilis (SDSE; a β-hemolytic streptococcus of human or animal origin) infections are emerging worldwide. We evaluated the clonal distribution of complement-mediated cell lysis-like gene (sicG) among SDSE isolates from three central prefectures of Japan.
Methods
Group G/C β-hemolytic streptococci were collected from three institutions from April 2014 to March 2016. Fifty-five strains (52 from humans and three from animals) were identified as SDSE on the basis of 16S rRNA sequencing data.; they were obtained from 25 sterile (blood, joint fluid, and cerebrospinal fluid) and 30 non-sterile (skin-, respiratory tract-, and genitourinary tract-origin) samples. emm genotyping, multilocus sequence typing, sicG amplification/sequencing, and random amplified polymorphic DNA (RAPD) analysis of sicG-positive strains were performed.
Results
sicG was detected in 30.9% of the isolates (16 human and one canine) and the genes from the 16 human samples (blood, 10; open pus, 3; sputum, 2; throat swab, 1) and one canine sample (open pus) showed the same sequence pattern. All sicG-harboring isolates belonged to clonal complex (CC) 17, and the most prevalent emm type was stG6792 (82.4%). There was a significant association between sicG presence and the development of skin/soft tissue infections. CC17 isolates with sicG could be divided into three subtypes by RAPD analysis.
In 1996, Vandamme et al [1] proposed that the new streptococcus subspecies, Streptococcus dysgalactiae subsp. equisimilis (SDSE), detected among human- and animal-origin streptococcal isolates, is a clinically relevant pathogen. This microorganism possesses Lancefield group G/C antigens (and rarely group A antigen), exhibits strong β-hemolytic activity and large colony size, and exerts streptokinase activity on human plasminogen and proteolytic activity on human fibrin. The S. anginosus group is a distinct category of group G/C streptococci, consisting of S. anginosus, S. constellatus, and S. intermedius. This S. anginosus group is easily distinguished from SDSE on the basis of biochemical characteristics and colony size on sheep blood agar. Since 2000, severe invasive infections (e.g., streptococcal toxic shock syndrome [STSS], necrotizing fasciitis, meningitis, infectious endocarditis, septic arthritis, and osteomyelitis) due to SDSE have been increasingly reported among elderly patients. The current worldwide medical conditions [23456] are similar to those caused by group A streptococci (GAS, S. pyogenes) and group B streptococci (GBS, S. agalactiae). In addition, neonatal STSS and the disease burden caused by SDSE among schoolchildren have also been examined [78]. Interestingly, sepsis with unknown foci is more frequent among GBS-infected patients than among SDSE- or GAS-infected patients, while cellulitis is less frequent among GBS-infected patients than among SDSE- or GAS-infected patients [6]. SDSE-infected patients present more often with septic arthritis than GBS-infected patients, whereas GAS-infected patients exhibit abscesses involving deeper than skin sites more often than SDSE-infected patients [6].
The emm gene encodes the filamentous surface M protein, which plays a role in attachment to host epithelial cells and exerts anti-phagocytosis activity against immune cells. The M protein is used for typing GAS and SDSE isolates in epidemiological investigations. Of the 229 isolates with emm genotypes investigated by Takahashi et al [9], 55 (24%) had the stG6792 type, which was strongly associated with poor patient outcomes.
A number of GAS strains express related secretory proteins: streptococcal inhibitor of complement (SIC) and the distantly related SIC (DRS) variant. SIC is found in only two GAS emm types (emm1 and emm57) and is thought to be a ligand for complement proteins C6 and C7, inhibiting the formation of the membrane attack complex, thereby blocking complement-mediated lysis [1011]. Similar to GAS, the streptococcal inhibitor of complement-mediated cell lysis-like gene (sicG), which encodes a newly discovered extracellular virulence factor, DrsG, is harbored by only a few emm type SDSE strains [12]. Interestingly, the protein encoded by sicG is reported to suppress the antimicrobial peptide LL-37, which is expressed in sweat as a part of the skin's innate defense system [13], suggesting the potential for cutaneous colonization by SDSE.
To date, only a few studies have examined the relationship between the presence of sicG and molecular epidemiological markers (e.g., emm genotypes, sequence types [STs], and antimicrobial resistance determinants) in SDSE isolates [14]. We aimed to determine the association between the presence of sicG and the microbiological and clinical features of SDSE isolates collected from three central prefectures (Saitama, Tokyo, and Chiba) in Japan over two years (2014–2016). At present, many people in Japan (from children to the elderly) have companion animals (dogs and cats) in their household. In addition, a number of hospitals have introduced animal-assisted therapy as a part of their mental care of patients. Therefore, we evaluated the molecular characteristics of SDSE strains from these animals.
A total of 64 group G/C β-hemolytic streptococci isolates were selected from the databases of three institutions (two hospitals and one laboratory center located in Saitama, Tokyo, and Chiba) from April 2014 to March 2016. Two veterinary clinicians kindly provided stored group G streptococcal isolates from companion animal infection sites. Each of the strains (one strain per host) was inoculated onto a sheep blood agar plate, which was incubated under 5% CO2 at 35℃ for 24 hr. Gray-white colonies with β-hemolytic activity were subjected to a latex agglutination test with specific antisera for the classification of Lancefield antigens (Seroiden Strepto kit, Eiken Chemical Co., Ltd., Tokyo, Japan). All isolates were stored at −70℃ to −80℃ until being processed for further evaluation. Group G strain D166B (ATCC 12394) was used as a quality control [15].
Patient information (including underlying medical conditions, clinical diagnoses, blood test data for bacterial cultures, therapeutic antimicrobial agents, and outcomes) concerning the presence of invasive or noninvasive diseases was retrieved from their medical charts. Invasive infections were defined as the isolation of SDSE from a sterile site (blood, joint fluid, or cerebrospinal fluid) [9], while noninvasive infections were defined as the isolation of SDSE from a nonsterile site (skin-, respiratory tract-, or genitourinary tract-origin). Information regarding companion animals (animal species, sex, age, clinical specimen, date collected, infectious disease, or clinical signs) was retrieved from the request sheets.
Several isolates were identified as SDSE on the basis of their biochemical properties determined by using either the API-20 Strep or Vitek2 system with the GP ID Card (SYSMEX bioMérieux Co., Ltd., Tokyo, Japan) after isolating the strains at three different institutions. These institutions sent all the isolates, which were identified as SDSE, to one laboratory (Kitasato Institute for Life Sciences) for further genetic analyses. Accurate identification was based on the sequencing results of the PCR-amplified 16S rRNA gene. SDSE identification was accepted if the isolate sequence yielded only one identification option with ≥98.7% similarity to the 16S rRNA sequence of SDSE type strain NCFB 1356(T). After determining species identity, we confirmed 55 strains (52 from humans and three from animals) as SDSE; they were derived from 25 sterile and 30 non-sterile samples.
The minimum inhibitory concentrations (MICs) of various antimicrobial agents, including classes of β-lactam, tetracycline, macrolide/lincosamide, fluoroquinolone, and others, against β-hemolytic streptococci were examined by using the broth microdilution method according to the Clinical and Laboratory Standards Institute guidelines with the same ATCC strain (Group G strain D166B, ATCC 12394) as the control [16].
We performed emm genotyping, multilocus sequence typing (MLST), and amplification/sequencing of sicG at our laboratory (Kitasato Institute for Life Sciences). Briefly, emm typing was based on the Centers for Disease Control and Prevention database (http://www2a.cdc.gov/ncidod/biotech/strepblast.asp) [9]. The MLST procedure was performed by sequencing seven housekeeping genes (gki, gtr, murI, mutS, recP, xpt, and atoB) according to the pubMLST website for S. dysgalactiae (http://pubmlst.org/sdysgalactiae/). The STs were grouped into clonal complexes (CCs), in which the connected STs were single locus variants differing by only one housekeeping gene [17]. sicG was PCR amplified by using two external and internal specific-primer sets, and amplified DNA was sequenced with the external forward primer, as previously described [13]. Furthermore, random amplified polymorphic DNA (RAPD) was analyzed for the sicG isolates as well as for the ATCC control strain, by using three different primers (H2, P5, and P6), to clarify the genetic relationship (subclonality) among the sicG-possessing strains [18].
The presence of antimicrobial resistance genes, including tet(M), tet(O), tet(K), tet(L), and tet(S) (tetracycline-resistance determinants) and erm(A), erm(B), and mef(A) (macrolide/lincosamide-resistance determinants), was confirmed by using PCR, as previously described [1920]. Additionally, we confirmed the correct resistance determinant sequences of several isolates that were positive for the target genes.
The study protocol was examined and approved by the human ethics committee of the three institutions as well as the ethics committee of the Sanritsu Zelkova Veterinary Laboratory before starting the investigation.
The relationship between emm genotype and CC number among the collected SDSE strains (n=55) is shown in Table 1. We identified 16 different emm types (most prevalent stG6792, n=18, 32.7%) and twenty different STs (most prevalent ST17, n=14, 25.5%). These STs were grouped into five CCs (CC17, CC15, CC29, CC8, and CC25), an ST37/ST278 group, and singletons (ST14, ST20, ST26, and ST294). There were three SDSE isolates from dogs (n=2, stG6792/ST17 and stC9431/ST14) and a cat (n=1, stC1929/ST294); the cat-origin ST294 was a novel strain.
The antimicrobial susceptibility test showed that 18 strains are resistant to either macrolide/lincosamide or tetracycline, as well as fluoroquinolone-resistant isolates (n=5). In addition, we found eight different single or combined resistance genotypes (most prevalent erm(A); n=7, 38.9%).
The SDSE strains with sicG features and host information from the three central prefectures (2014–2016), are summarized in Table 2. Carbohydrate group G was detected in 30.9% of the sicG-possessing isolates. These isolates originated from 16 human (blood, n=10; open pus, n=3; sputum, n=2; throat swab, n=1) and one canine (open pus) samples. The sequences of all sicG amplicons exhibited a single pattern and were closely related to the sicG sequence of Japanese strain RE378 (containing stG6792, 486-bp, 100% homology, accession no. AP011114.1) and Japanese strain TK01 (containing stG2078, 485/486-bp, 99.8% homology, AB508817.1), which were already present in the database for BLAST analysis. Multi-alignments of sicG from isolate 6 (dog), isolate 14 (human), and isolate 17 (human), as well as that from strain RE378 and strain TK01, are shown in Fig. 1.
All isolates possessing sicG carried CC17, which consisted of ST17 (n=11), ST205 (n=3), or ST206 (n=3). In addition, isolates with sicG harbored stG6792 (n=14, 82.4%), stG2078 (n=2), or stC36 (n=1). Two isolates were resistant to fluoroquinolone class antibiotics, and one was resistant to tetracycline owing to the presence of tet(M). The sicG strains were isolated in Saitama (n=6), Chiba (n=6), and Tokyo (n=5).
We observed statistically significant relationships between sicG detection and the presence of either CC17 or stG6792 (P<0.01 for each). There was also a significant association between sicG presence and the development of skin and soft tissue infections (P=0.03). In contrast, although we encountered invasive (n=10) and noninvasive (n=7) cases due to strains with sicG, there was no significant association between the presence of sicG and the development of invasive infections (P=0.24).
Images based on RAPD of SDSE isolates using different primers (H2, P5, and P6) are presented in Fig. 2. All isolates with sicG were different from the ATCC control strain. The clones harboring both sicG and CC17 were divided into three groups (A, B, and C), as demonstrated by RAPD analysis. Group A included 13 strains, whereas groups B and C included only two isolates each, suggesting the spread of the dominant group A sub-clone into the three central prefectures.
Table 3 summarizes our sicG-positive SDSE isolate prevalence data and that from previously published studies [12132122]. Davies et al [23] applied a targeted microarray containing 216 GAS virulence genes to profile the virulence gene repertoires of 58 human-origin SDSE isolates including 46 group G strains (16 from invasive infections) and 12 group C strains (two from invasive infections) in Australia; 9% of the group G isolates carried sicG, while no group C isolates harbored sicG. Although there are differences in strain source, investigation period, and the number of screened isolates, the detection rate of sicG-positive isolates (30.9%) seems to be relatively high in our study, suggesting that it constitutes a clonal type. Interestingly, our findings that stG6792 (32.7%) was the most prevalent emm type, indicating a clonal type, and that a significant proportion of isolates carrying sicG (82.4%) also harbored stG6792, suggest the clonal combination of sicG with stG6792. Moreover, the presence of sicG was significantly associated with the development of skin and soft tissue infections. In addition, sicG strains containing stG2078 (n=3) were consistently associated with severe soft tissue infections [22]. In contrast, there was no significant relationship between sicG detection and the development of invasive infections in our survey. As this is a preliminary study conducted over a short period (two years), further investigation of the epidemiological features of additional SDSE strains should be undertaken to clarify the association between the presence of sicG and microbiological and clinical features,.
Oppegaard et al [22] described the sequence diversity of sicG (six alleles, sicG1-6) among SDSE strains. This diversity was mainly caused by in-frame deletion/insertion mutations involving repeats, along with point mutations leading to non-synonymous substitutions. However, in this study, all sicG amplicons (n=17) exhibited a single sequence pattern, which was similar to that of sicG in strains RE378 (stG6792, 100% homology) and TK01 (stG2078, 99.8% homology, sicG4 allele). Likewise, sequencing analysis of other sicG-positive isolates (n=19) from Japan revealed that their sequence was identical to that in TK01 [12]. In addition, all sicG isolates in this study belonged to CC17. To the best of our knowledge this is the first report regarding the association between the presence of sicG and CC. The genetic relatedness among sicG strains, analyzed by RAPD, suggests the spread of a dominant sub-clone (group A, n=13). Therefore, this main sub-clone harboring sicG and CC17 might be distributed in the three central prefectures of Japan.
The location of the sic gene (915-bp) was previously identified in the chromosome of a GAS M1 strain [1024]. This gene is located in the Mga regulon, which includes three other virulence genes: emm1; sph, encoding protein H; and scpA, encoding streptococcal C5a peptidase. The sic gene was found in a very limited number of emm type GAS strains. However, sicG is not located near emm. For example, in the genome of RE378, the sicG sequence starts at nucleotide 950611, while emm (stG6792) starts at nucleotide 208440. In the genome of Japanese strain GGS_124 (AP010935.1), the sicG sequence starts at nucleotide 917183, whereas the emm (stG480) sequence starts at nucleotide 204718. Further investigation of the regulatory mechanism and other functions of sicG might elucidate the virulent conditions among SDSE isolates from humans and animals.
To the best of our knowledge, this is the first report of a sicG-possessing SDSE isolate (CC17 and stG6792) from the open pus of a house pet (a dog). Our observations suggest a potential risk for the transfer of some virulent strains between companion animals and human owners and the subsequent clonal spread in a community. Schrieber et al [25] documented the findings of an identical SDSE isolate (carbohydrate group C, stC839.5, and ST3) using pharyngeal swabs collected from a child and his pet dog in an Aboriginal Australian community. They recommended that the epidemiological investigation of SDSE in hyper-endemic human populations should include strains isolated from animals. Therefore, on the basis of the “One Health” concept [26], an epidemiological survey of SDSE (a zoonotic pathogen) isolated from humans and animals needs to be conducted. Moreover, several SDSE strains are able to transfer between host species and may possess genetic variability and the ability to acquire virulence.
In conclusion, our results suggest the possible spread of three SDSE CC17 subclones with sicG in three prefectures in central Japan. Further studies of isolates from diverse geographic areas might be required to monitor the clonal spread of these virulent SDSE strains.
Figures and Tables
Fig. 1
Multi-alignments of isolate 6 (dog), isolate 14 (human), isolate 17 (human), strain RE378, and strain TK01. These alignments were generated with CLUSTAL W version 2.1 (latest version) (http://clustalw.ddbj.nig.ac.jp/).
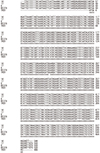
Fig. 2
Random amplified polymorphic DNA analysis of Streptococcus dysgalactiae subsp. equisimilis isolates with streptococcal inhibitor of complement-mediated cell lysis-like gene (sicG), using three different primers: H2, P5, and P6.
Abbreviations: M, marker; A, ATCC 12394.
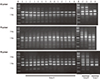
Table 1
Relationship between emm type and clonal complex number among Streptococcus dysgalactiae subsp. equisimilis strains collected
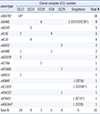
Table 2
Features of Streptococcus dysgalactiae subsp. equisimilis strains with streptococcal inhibitor of complement-mediated cell lysis-like gene and the hosts, Japan (2014–2016)
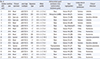
All the strains with sicG belonged to the carbohydrate group G and clonal complex 17.
Abbreviations: sicG, streptococcal inhibitor of complement-mediated cell lysis-like gene; y, years; m, months; M, male; F, female.
Sequence of all sicG amplicons was single pattern, and was similar to those of sicG in RE378 (AP011114.1, stG6792) (full length of sicG 486 bp, 100% homology) and TK01 (AB508817.1, stG2078) (485/486 bp, 99.8%).
Table 3
Prevalence of Streptococcus dysgalactiae subsp. equisimilis strains with streptococcal inhibitor of complement-mediated cell lysis-like gene
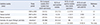
Isolation country (area) | Study period | N of screened isolates (N of isolates from sterile specimens) | N (%) of sicG-positive isolates | N (%) of sicG- positive isolates from sterile specimens | N (%) of sicG- positive isolates with stG6792 | Reference No. |
---|---|---|---|---|---|---|
Japan | 2000 to 2009 | 110 (51) | 19 (17.3) | 11 (57.9) | 0 | 12 |
Taiwan (central) | 2007 to 2011 | 246 (66) | 6 (2.4) | 1 (16.7) | 3 (50) | 21 |
Norway (western) | 2003 to 2009 | 129 (65) | 15 (11.6) | 8 (53.3) | 1 (6.7) | 22 |
Australia, US, Japan, Portugal, and India | Uncertain* | 193 (ND) | 30 (15.5) | ND | 0 | 13 |
Japan (central 3 prefectures) | 2014 to 2016 | 55 (25) | 17 (30.9) | 10 (58.8) | 14 (82.4) | This study |
Acknowledgments
The authors wish to thank Ms. Mieko Goto (Laboratory of Infectious Diseases, Kitasato Institute for Life Sciences, Kitasato University), Dr. Goro Kurita (Kurita Animal Hospital), and Dr. Yoshiteru Murata (Murata Animal Hospital) for their assistance. This publication made use of the Streptococcus dysgalactiae MLST website (http://pubmlst.org/sdysgalactiae/) developed at the University of Oxford (Jolley & Maiden 2010, BMC Bioinformatics, 11:595). The development of this site has been funded by the Wellcome Trust. In addition, we wish to thank Editage (www.editage.jp) for English language editing.
References
1. Vandamme P, Pot B, Falsen E, Kersters K, Devriese LA. Taxonomic study of lancefield streptococcal groups C, G, and L (Streptococcus dysgalactiae) and proposal of S. dysgalactiae subsp. equisimilis subsp. nov. Int J Syst Bacteriol. 1996; 46:774–781.
2. Broyles LN, Van Beneden C, Beall B, Facklam R, Shewmaker PL, Malpiedi P, et al. Population-based study of invasive disease due to β-hemolytic streptococci of groups other than A and B. Clin Infect Dis. 2009; 48:706–712.


3. Cohen-Poradosu R, Jaffe J, Lavi D, Grisariu-Greenzaid S, Nir-Paz R, Valinsky L, et al. Group G streptococcal bacteremia in Jerusalem. Emerg Infect Dis. 2004; 10:1455–1460.


4. Liao CH, Liu LC, Huang YT, Teng LJ, Hsueh PR. Bacteremia caused by group G streptococci, Taiwan. Emerg Infect Dis. 2008; 14:837–840.


5. Sylvetsky N, Raveh D, Schlesinger Y, Rudensky B, Yinnon AM. Bacteremia due to beta-hemolytic Streptococcus group G: increasing incidence and clinical characteristics of patients. Am J Med. 2002; 112:622–626.


6. Takahashi T, Ubukata K, Watanabe H. Invasive infection caused by Streptococcus dysgalactiae subsp. equisimilis: characteristics of strains and clinical features. J Infect Chemother. 2011; 17:1–10.
7. Yamaoka S, Ogihara T, Yasui M, Hasegawa M, Hira S, Oue S, et al. Neonatal streptococcal toxic shock syndrome caused by Streptococcus dysgalactiae subsp. equisimilis. Pediatr Infect Dis J. 2010; 29:979–981.
8. Bramhachari PV, Kaul SY, McMillan DJ, Shaila MS, Karmarkar MG, Sriprakash KS. Disease burden due to Streptococcus dysgalactiae subsp. equisimilis (group G and C streptococcus) is higher than that due to Streptococcus pyogenes among Mumbai school children. J Med Microbiol. 2010; 59:220–223.
9. Takahashi T, Sunaoshi K, Sunakawa K, Fujishima S, Watanabe H, Ubukata K. Clinical aspects of invasive infections with Streptococcus dysgalactiae ssp. equisimilis in Japan: differences with respect to Streptococcus pyogenes and Streptococcus agalactiae infections. Clin Microbiol Infect. 2010; 16:1097–1103.
10. Åkesson P, Sjöholm AG, Björck L. Protein SIC, a novel extracellular protein of Streptococcus pyogenes interfering with complement function. J Biol Chem. 1996; 271:1081–1088.
11. Fernie-King BA, Seilly DJ, Willers C, Würzner R, Davies A, Lachmann PJ. Streptococcal inhibitor of complement (SIC) inhibits the membrane attack complex by preventing uptake of C567 onto cell membranes. Immunology. 2001; 103:390–398.


12. Minami M, Ichikawa M, Matsui H, Hata N, Wakiyama N, Matsumoto M, et al. Prevalence of a streptococcal inhibitor of a complement-mediated cell lysis-like gene (sicG) in Streptococcus dysgalactiae subsp. equisimilis. Curr Microbiol. 2011; 62:884–887.
13. Smyth D, Cameron A, Davies MR, McNeilly C, Hafner L, Sriprakash KS, et al. DrsG from Streptococcus dysgalactiae subsp. equisimilis inhibits the antimicrobial peptide LL-37. Infect Immun. 2014; 82:2337–2344.
14. Fujita T, Horiuchi A, Ogawa M, Yoshida H, Hirose Y, Nagano N, et al. Genetic diversity in Streptococcus dysgalactiae subsp. equisimilis isolates from patients with invasive and noninvasive infections in a Japanese university hospital (2014–2015). Jpn J Infect Dis. 2017; 70:100–104.
15. Streptococcus dysgalactiae subsp. equisimilis. Vandamme et al. emend. Vieira et al. (ATCC® 12394™). Accessed on September 8, 2015. http://www.atcc.org/products/all/12394.aspx.
16. Clinical and Laboratory Standards Institute. Performance standards for antimicrobial susceptibility testing. Twenty-second Informational supplement, M100-S22. Wayne, PA: Clinical and Laboratory Standards Institute;2012.
17. McMillan DJ, Bessen DE, Pinho M, Ford C, Hall GS, Melo-Cristino J, et al. Population genetics of Streptococcus dysgalactiae subspecies equisimilis reveals widely dispersed clones and extensive recombination. PLoS One. 2010; 5:e11741.
18. Bert F, Picard B, Branger C, Lambert-Zechovsky N. Analysis of genetic relationships among strains of groups A, C and G streptococci by random amplified polymorphic DNA analysis. J Med Microbiol. 1996; 45:278–284.


19. Haenni M, Saras E, Bertin S, Leblond P, Madec JY, Payot S. Diversity and mobility of integrative and conjugative elements in bovine isolates of Streptococcus agalactiae, S. dysgalactiae subsp. dysgalactiae, and S. uberis. Appl Environ Microbiol. 2010; 76:7957–7965.
20. Malhotra-Kumar S, Lammens C, Piessens J, Goossens H. Multiplex PCR for simultaneous detection of macrolide and tetracycline resistance determinants in streptococci. Antimicrob Agents Chemother. 2005; 49:4798–4800.


21. Lo HH, Cheng WS. Distribution of virulence factors and association with emm polymorphism or isolation site among beta-hemolytic group G Streptococcus dysgalactiae subspecies equisimilis. APMIS. 2015; 123:45–52.
22. Oppegaard O, Mylvaganam H, Skrede S, Langeland N, Kittang BR. Sequence diversity of sicG among group C and G Streptococcus dysgalactiae subspecies equisimilis isolates associated with human infections in western Norway. Eur J Clin Microbiol Infect Dis. 2014; 33:273–277.
23. Davies MR, McMillan DJ, Beiko RG, Barroso V, Geffers R, Sriprakash KS, et al. Virulence profiling of Streptococcus dysgalactiae subspecies equisimilis isolated from infected humans reveals 2 distinct genetic lineages that do not segregate with their phenotypes or propensity to cause diseases. Clin Infect Dis. 2007; 44:1442–1454.
24. Mejia LM, Stockbauer KE, Pan X, Cravioto A, Musser JM. Characterization of group A Streptococcus strains recovered from Mexican children with pharyngitis by automated DNA sequencing of virulence-related genes: unexpectedly large variation in the gene (sic) encoding a complement-inhibiting protein. J Clin Microbiol. 1997; 35:3220–3224.
25. Schrieber L, Towers R, Muscatello G, Speare R. Transmission of Streptococcus dysgalactiae subsp. equisimilis between child and dog in an Aboriginal Australian community. Zoonoses Public Health. 2014; 61:145–148.
26. Centers for Disease Control and Prevention. One Health. Updated on January 25, 2017. https://www.cdc.gov/onehealth/.