Abstract
Changes in microparticles (MP) from red blood cell (RBC) concentrates in the context of irradiation have not been investigated. The aim of this study was to evaluate how irradiation affects the number of MPs within transfusion components. Twenty RBC concentrates, within 14 days after donation, were exposed to gamma rays (dose rate: 25 cGy) from a cesium-137 irradiator. Flow cytometry was used to determine the numbers of MPs derived from RBC concentrates before and 24 hr after irradiation. The mean number of MPs (±standard deviation) in RBC concentrates was 21.9×109/L (±22.7×109/L), and the total number of MPs ranged from 2.6×109/L to 96.9×109/L. The mean number of MPs increased to 22.6×109/L (±31.6×109/L) after irradiation. Before irradiation, the CD41-positive and CD235a-positive MPs constituted 9.5% (1.0×109/L) and 2.2% (263×106/L) of total MPs, respectively. After irradiation, CD41-positive MPs increased to 12.1% (1.5×109/L) (P=0.014), but the CD235a-positive MPs decreased to 2.0% (214×106/L) of the total MPs (P=0.369). Irradiation increases the number of CD41-positive MPs within RBC concentrates, suggesting the irradiation of RBC concentrates could be associated with thrombotic risk of circulating blood through the numerical change.
Microparticles (MPs), ranging in size from 0.1 to 1.0 µm, are released in the blood circulation from the cell membrane of various cells, following activation or apoptosis [12]. They retain the characteristics of their parent cells, thus allowing identification of their cellular origin [1].
Increased numbers of certain kinds of MPs (endothelial, erythrocyte, or other cell-derived MPs) are reported in cancer, autoimmune diseases, blood disorders, cardiovascular diseases, infectious diseases, disorders of the female reproductive system, and kidney disorders [23]. They have diagnostic potential as biomarkers for those diseases [24]. However, only a few studies have focused on the MPs of blood products [567]. In a previous study on blood products, cell activation through calcium influx during the storage of blood products was suggested to form immunogenic MPs and increase the risk of transfusion complications [5]. However, changes in MPs from RBC concentrates in the context of irradiation have not been investigated. The aim of this study was to demonstrate the hypothesis that gamma-irradiation of RBC concentrates might induce increased MPs in the blood product through comparing the number of MPs before and after gamma-irradiation of RBC concentrates.
RBC concentrates were derived from twenty healthy donors. Whole blood (400 mL) was collected from each volunteer donor who attended the Republic of Korea National Red Cross on the same day. The collected blood was stored in bags containing a citrate-phosphate-dextrose-adenine-1 anticoagulant solution at room temperature, prior to processing. After separation of plasma from the erythrocytes by centrifugation within eight hours of phlebotomy, the RBC concentrates were stored at 4℃. All RBC units in blood bags were individually irradiated with an IBL 437 cesium-137 irradiator (CIS bio International, Bangnols sur Ceze, France) at doses of 25 cGy within 14 days after collection. Samples for counting MPs were drawn from RBC concentrates prior to and 24 hr after irradiation. Using these samples, white blood cells (WBCs), RBCs, platelet counts, Hb concentration, and Hct in RBC concentrates were determined by the Sysmex XE-5000 automated cell counter (Sysmex Corporation, Kobe, Japan).
To isolate MPs as previously described [8], 800 µL of RBC concentrates in 1.5 mL micro-centrifuge tube (Sigma Aldrich Co., St. Louis, MO, USA) was centrifuged at 1,500g for 15 min to remove the blood cells. The supernatant containing the MPs was collected, followed by a second ultracentrifugation step at 13,000g for 2 min. The residual pellet was then suspended in phosphate buffered saline (PBS) and tested within 4 hr. For labeling MPs, 10 µL of the MP suspension was incubated with 1.5 µL each of anti-human CD235a labeled with phycoerythrin (PE) and anti-human CD41 labeled with fluorescein isothiocyanate (FITC) from BD Bioscience Inc. (Franklin Lakes, NJ, USA) for 10 min in the dark. Just prior to flow cytometric analysis, 500 µL of PBS and 30 µL of size-calibration beads of 0.5 and 0.9 µm in diameter formulated to a numerical ratio of 2:1, called Megamix (0.5, 0.9, and 3.0 µm; BioCytex, Marseille, France), were added to the labeled samples. In addition, 30 µL of counting beads with an established concentration of around 1,000 beads/µL (Flow Count Fluorospheres; Beckman-Coulter, Villepinte, France) were added to each sample in order to enumerate the MPs as absolute numbers per microliter [9].
Flow cytometric analyses were performed by using the Cytomics FC500 flow cytometer (Beckman-Coulter). MPs were gated on the basis of their forward scatter (FS) and side scatter (SS) signals with logarithmic fluorescence scales as previously reported [8] (Fig. 1). MPs were defined as events within the MP region as shown in the FS×SS plot (Fig. 1).
The statistical analyses were performed by using SPSS software (version 20.0, SPSS, Chicago, IL, USA). Paired t-test was used to compare numbers of MPs, WBCs, RBCs, platelets, and the concentrations of Hb and Hct within transfusion components in pre-irradiation and post-irradiation conditions. Pearson's correlation coefficient between the number of platelets (or WBCs) and total MPs was determined. P<0.05 was considered statistically significant.
The characteristics of RBC concentrates before and after irradiation are summarized in Table 1. The means of WBC, RBC, and platelet counts, and the concentrations of Hb and Hct did not show any statistical differences between pre-irradiation and post-irradiation samples. Before irradiation, the Pearson's correlation coefficients between the number of platelets and total MPs and between the number of WBCs and total MPs were -0.235 (P=0.332) and 0.117 (P=0.634), respectively. After irradiation, these values changed to -0.141 (P=0.565) and 0.046 (P=0.851), respectively. There was no significant difference between correlation coefficients before and after irradiation.
The baseline mean MP counts of the RBC concentrates (n=20) was 21.9×109/L, which increased to 22.6×109/L at 24 hr after irradiation without reaching statistical significance (P=0.785). Before irradiation, the mean percentage (mean number) of CD41-positive MPs and CD235a-positive MPs was 9.5% (1.0×109/L) and 2.2% (263×106/L) of total MPs, respectively (Table 1). After irradiation, the mean percentage of CD41-positive MPs significantly increased to 12.1% (1.5×109/L) (P=0.014). However, the mean percentage of CD235a-positive MPs decreased to 2.0% (214×106/L) of total MPs without reaching statistical significance (P=0.369).
In a recent study using rat-derived mesenteric arteries, Liu et al. [10] showed that MPs could contribute to increased inflammation, thrombosis, and blood storage lesions through loss of nitric oxide (NO) bioavailability. MP-encapsulated Hb was demonstrated to reduce NO bioavailability about 1,000 times faster than the red cell-encapsulated Hb and scavenge NO similarly to cell free Hb [10]. Transfusion of three or four units of stored blood could increase red cell MPs to a level at which they could affect NO bioavailability [10]. Accordingly, the numerical change in the MPs in the transfusion units has a direct impact on such negative consequences.
In this study, the total average MP count within the RBC concentrates increased after irradiation, even though no statistically significant difference was observed. According to their cellular origin, only CD41-positive MPs showed a statistically significant numerical increase after irradiation, whereas CD235a-positive MPs did not increase.
CD41-positive MPs in the RBC concentrates were more numerous than the CD235a-positive MPs, consistent with the report that platelet-derived MPs are the most abundant types of MPs in human circulation [11]. CD41-positive MPs might be helpful in restoring hemostasis and preventing overt bleeding in patients post-surgery or post liver biopsy (in case of severe liver cirrhosis) [8]. CD41-positive MPs are reported to facilitate decreased bleeding time in diseases such as storage pool disease [5]. On the other hand, CD41-positive MPs were demonstrated to have about 50 to 100 times more procoagulant activity than the platelets, being associated with certain pathophysiologic processes of prothrombotic diseases [1112]. Therefore, increased CD41-positive MPs could lead to thrombosis associated with tumor development [13]. In addition, CD41-positive MPs may contribute to the residual thrombotic risk reported in splenectomized, multi-transfused β-thalassemia major patients [14] and the vaso-occlusive phenotype-related severity in sickle cell anemia [15]. Besides, CD41-positive MPs represent a pathological mediator of inflammation, and increased CD41-positive MPs were detected in diseases such as rheumatoid arthritis, Sjogren syndrome, mixed connective tissue disease, and atherothrombosis [216]. Although the precise risk for CD41-positive MPs in inflammatory and thrombotic diseases is yet unknown, the possible risk could be high enough when irradiated transfusion units are donated to patients with such diseases [11]. Such a risk would be especially evident under conditions like massive transfusion.
A previous study evaluating the effects of ionizing radiation on cellular pro-coagulability of human peripheral blood mononuclear cells (PBMNCs) showed that both cellular components and MPs derived from the PBMNCs had greater tissue factor activity after irradiation than before irradiation [17]. Although the numerical change in MPs after irradiation was not evaluated in that study, the large irradiation dose (20 Gy) used in the previous study compared with that (25 cGy) used in this study could have possibly increased the numbers of total MPs including CD41 and CD235a-positive MPs in PBMNCs [17].
In this study, we selected the surface markers, CD41 and CD235, because the most frequently detected MPs in healthy individuals are derived from platelets and erythrocytes [1518]. However, in future studies, inclusion of CD45 as a surface marker for WBC-derived MPs will clearly help elucidate the effect of irradiation on MPs from RBC concentrates.
In conclusion, only CD41-positive MPs in the RBC concentrates showed a significant numerical increase after irradiation. While future evaluation using more specimens under various irradiation doses is required, the present result suggests that irradiation of transfusion units could increase thrombotic risk of circulating blood owing to the change in the number of CD41-positive MPs.
References
1. Ayers L, Kohler M, Harrison P, Sargent I, Dragovic R, Schaap M, et al. Measurement of circulating cell-derived microparticles by flow cytometry: sources of variability within the assay. Thromb Res. 2011; 127:370–377. PMID: 21257195.


2. Barteneva NS, Fasler-Kan E, Bernimoulin M, Stern JN, Ponomarev ED, Duckett L, et al. Circulating microparticles: square the circle. BMC Cell Biol. 2013; 14:23. PMID: 23607880.


3. Jayachandran M, Litwiller RD, Owen WG, Heit JA, Behrenbeck T, Mulvagh SL, et al. Characterization of blood borne microparticles as markers of premature coronary calcification in newly menopausal women. Am J Physiol Heart Circ Physiol. 2008; 295:H931–H938. PMID: 18621859.


4. Jung KH, Chu K, Lee ST, Park HK, Bahn JJ, Kim DH, et al. Circulating endothelial microparticles as a marker of cerebrovascular disease. Ann Neurol. 2009; 66:191–199. PMID: 19743467.


5. Canellini G, Rubin O, Delobel J, Crettaz D, Lion N, Tissot JD. Red blood cell microparticles and blood group antigens: an analysis by flow cytometry. Blood Transfus. 2012; 10(S2):S39–S45. PMID: 22890266.
6. Chan KS, Sparrow RL. Microparticle profile and procoagulant activity of fresh-frozen plasma is affected by whole blood leukoreduction rather than 24-hour room temperature hold. Transfusion. 2014; 54:1935–1944. PMID: 24635475.


7. Lawrie AS, Harrison P, Cardigan RA, Mackie IJ. The characterization and impact of microparticles on haemostasis within fresh-frozen plasma. Vox Sang. 2008; 95:197–204. PMID: 19121184.


8. Keuren JF, Magdeleyns EJ, Govers-Riemslag JW, Lindhout T, Curvers J. Effects of storage-induced platelet microparticles on the initiation and propagation phase of blood coagulation. Br J Haematol. 2006; 134:307–313. PMID: 16848773.


9. Robert S, Poncelet P, Lacroix R, Arnaud L, Giraudo L, Hauchard A, et al. Standardization of platelet-derived microparticle counting using calibrated beads and a Cytomics FC500 routine flow cytometer: a first step towards multicenter studies. J Thromb Haemost. 2009; 7:190–197. PMID: 18983485.


10. Liu C, Zhao W, Christ GJ, Gladwin MT, Kim-Shapiro DB. Nitric oxide scavenging by red cell microparticles. Free Radic Biol Med. 2013; 65:1164–1173. PMID: 24051181.


11. Kriebardis A, Antonelou M, Stamoulis K, Papassideri I. Cell-derived microparticles in stored blood products: innocent-bystanders or effective mediators of post-transfusion reactions. Blood Transfus. 2012; 10(S2):S25–S38. PMID: 22890265.
12. Rubin O, Delobel J, Prudent M, Lion N, Kohl K, Tucker EI, et al. Red blood cell-derived microparticles isolated from blood units initiate and propagate thrombin generation. Transfusion. 2013; 53:1744–1754. PMID: 23228139.


13. Mezouar S, Mege D, Darbousset R, Farge D, Debourdeau P, Dignat-George F, et al. Involvement of platelet-derived microparticles in tumor progression and thrombosis. Semin Oncol. 2014; 41:346–358. PMID: 25023350.


14. Agouti I, Cointe S, Robert S, Judicone C, Loundou A, Driss F, et al. Platelet and not erythrocyte microparticles are procoagulant in transfused thalassaemia major patients. Br J Haematol. 2015; 171:615–624. PMID: 26205481.


15. Nebor D, Bowers A, Connes P, Hardy-Dessources MD, Knight-Madden J, Cumming V, et al. Plasma concentration of platelet-derived microparticles is related to painful vaso-occlusive phenotype severity in sickle cell anemia. PLoS One. 2014; 9:e87243. PMID: 24475257.


16. Goubran HA, Burnouf T, Stakiw J, Seghatchian J. Platelet microparticle: a sensitive physiological "fine tuning" balancing factor in health and disease. Transfus Apher Sci. 2015; 52:12–18. PMID: 25599988.


17. Goldin-Lang P, Niebergall F, Antoniak S, Szotowski B, Rosenthal P, Pels K, et al. Ionizing radiation induces upregulation of cellular procoagulability and tissue factor expression in human peripheral blood mononuclear cells. Thromb Res. 2007; 120:857–864. PMID: 17337288.


18. Van Der Meijden PE, Van Schilfgaarde M, Van Oerle R, Renné T, ten Cate H, Spronk HM. Platelet- and erythrocyte-derived microparticles trigger thrombin generation via factor XIIa. J Thromb Haemost. 2012; 10:1355–1362. PMID: 22537188.


Fig. 1
(A-C) Gating strategy for the identification of microparticles (MPs). (A) The MP region is set up. On a forward scatter (FS) log×side scatter (SS) log cytogram, the MP region is restricted to the lower region by the 0.5 µm beads and to the upper region by the 0.9 µm beads. Three-micrometer beads were responsible for a discrete cloud of events that were easily distinguished above background noise or debris. MPs are seen in (B) as blue dots against the background (gray dots). CD235a-positive MPs are shown in (C) as violet dots within the box. (D-G) Analysis of CD41/CD235a stained MPs in pre- and post-irradiation conditions using flow cytometry. (D) CD235a-positive MPs within RBC concentrates (violet dots) before irradiation; (E) CD235a-positive MPs within RBC concentrates (violet dots) after irradiation; (F) CD41-positive MPs within RBC concentrates before irradiation (green dots); (G) CD41-positive MPs within RBC concentrates after irradiation (green dots).
Abbreviations: PE, phycoerythrin; FITC, fluorescein isothiocyanate; RBC, red blood cell.
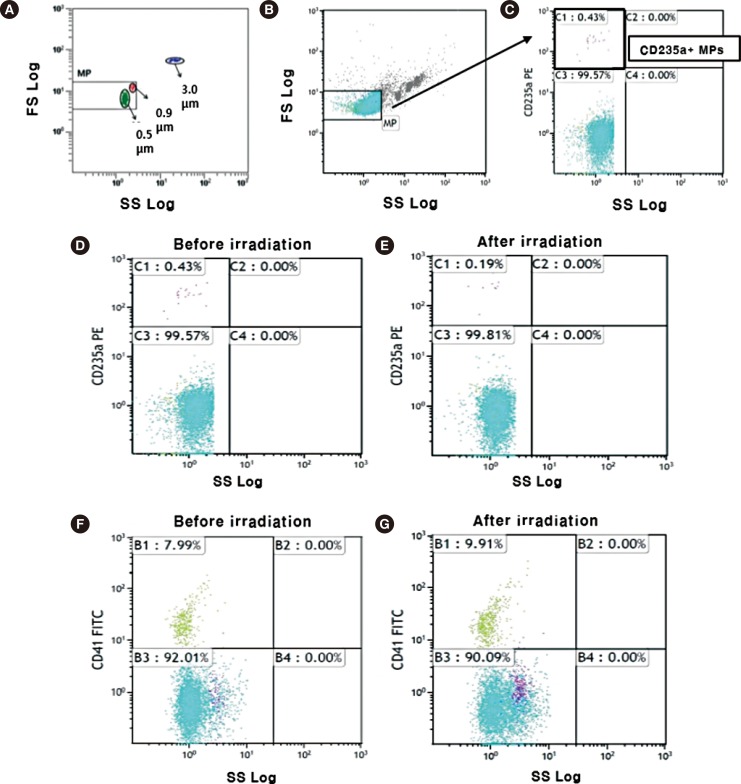
Table 1
Changes in RBC- and platelet-derived microparticle counts and the blood component parameters before and after irradiation of the RBC concentrates (n=20)
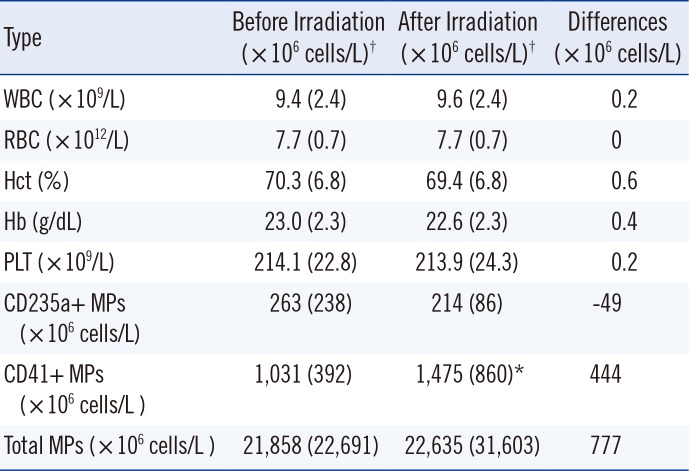