Abstract
Background
Acinetobacter baumannii infections are difficult to treat owing to the emergence of various antibiotic resistant isolates. Because treatment options are limited for multidrug-resistant (MDR) A. baumannii infection, the discovery of new therapies, including combination therapy, is required. We evaluated the synergistic activity of colistin, doripenem, and tigecycline combinations against extensively drug-resistant (XDR) A. baumannii and MDR A. baumannii.
Methods
Time-kill assays were performed for 41 XDR and 28 MDR clinical isolates of A. baumannii by using colistin, doripenem, and tigecycline combinations. Concentrations representative of clinically achievable levels (colistin 2 µg/mL, doripenem 8 µg/mL) and achievable tissue levels (tigecycline 2 µg/mL) for each antibiotic were used in this study.
Results
The colistin-doripenem combination displayed the highest rate of synergy (53.6%) and bactericidal activity (75.4%) in 69 clinical isolates of A. baumannii. Among them, thedoripenem-tigecycline combination showed the lowest rate of synergy (14.5%) and bacteri-cidal activity (24.6%). The doripenem-tigecycline combination showed a higher antagonistic interaction (5.8%) compared with the colistin-tigecycline (1.4%) combination. No antagonism was observed for the colistin-doripenem combination.
Conclusions
The colistin-doripenem combination is supported in vitro by the high rate of synergy and bactericidal activity and lack of antagonistic reaction in XDR and MDR A. baumannii. It seems to be necessary to perform synergy tests to determine the appropri-ate combination therapy considering the antagonistic reaction found in several isolates against the doripenem-tigecycline and colistin-tigecycline combinations. These findings should be further examined in clinical studies.
Acinetobacter baumannii has emerged as an important nosocomial pathogen that causes infections mainly in patients in intensive care units [12]. These bacteria cause various accompanying illnesses such as pneumonia, urinary tract infections, septicemia, meningitis, and wound infections in immunocompromised patients [1]. During the past decade, the management of A. baumannii infections has been a great challenge owing to the increasing number of isolates exhibiting multiple antimicrobial resistance. Multidrug-resistant A. baumannii (MDR-AB) infections are associated with high mortality rates and longer hospital stays [3]. Because optimal treatment regimens for MDR-AB isolates are not well defined, the discovery of new treatments, including combination therapy, is required.
Carbapenems have been commonly used as the treatment of choice for MDR-AB infections [4]. However, MDR-AB isolates resistant to carbapenems have been increasingly reported worldwide [567]. Recently, colistin and tigecycline have emerged as alternative therapeutic options for MDR-AB infections [891011]. However, resistance to these antimicrobial agents has also been reported as a result of the increased usage of colistin and tigecycline [8121314]. Thus, many recent studies have investigated combinations of two or more agents for treating MDR-AB infections. Specifically, studies on combinations including colistin have frequently been reported [13]. Colistin combination treatment is useful for preventing antibiotic resistance and reducing toxicity [15]. Doripenem, the latest broad-spectrum carbapenem approved in the United States, is more stable against carbapenemase than other carbapenems [16]. Thus, doripenem has emerged as a new treatment option for MDR-AB infections.
Thus far, several combinations, including colistin-tigecycline and colistin-doripenem, have been reported to be effective in vivo or in vitro against MDR-AB [1617]. However, reports on the effects of these combinations against extensively drug-resistant (XDR) and MDR-AB clinical isolates have been rarely found. In this study, we evaluated the synergistic effects of combinations of antimicrobial agents against XDR and MDR-AB isolates by in vitro time-kill analysis.
Of 69 A. baumannii clinical isolates, 64 were obtained from Chosun University during the period from January 2009 to June 2014. Five isolates, characterized in a previous study of antimicrobial resistance carried out by the Korean Antimicrobial Resistance Monitoring System (KARMS) in 2013 [18], were added. A. baumannii was initially identified by using the Vitek 2 system (bioMérieux, Marcy-l''Etoile, France). Thereafter, species identification was confirmed by gyrB multiplex PCR. Some isolates of A. baumannii, identified previously upto the species level by molecular methods using rpoB gene sequencing and 16S rRNA gene sequencing, were also included [19]. Additionally, they were divided into the XDR (41 isolates) and MDR groups (28 isolates) according to the antimicrobial susceptibility patterns in the following antimicrobial categories: aminoglycosides, antipseudomonal carbapenems, antipseudomonal fluoroquinolones,antipseudomonal penicillins plus β-lactamase inhibitors, extended-spectrum cephalosporins, folate pathway inhibitors, penicillins plus β-lactamase inhibitors, polymyxins, and tetracyclines [20]. XDR was defined as acquired non-susceptibility to at least one agent in all but two or fewer antimicrobial categories. MDR was defined as non-susceptible to at least one agent in three or more antimicrobial categories.
Minimum inhibitory concentration (MIC) determinations for colistin, doripenem, and tigecycline were performed by agar dilution in accordance with the CLSI recommendations [21]. The antimicrobial agents used in this study were purchased from Sigma-Aldrich (St. Louis, MO, USA). Colistin and doripenem MIC results were interpreted according to the CLSI breakpoint criteria [21]. No breakpoints for tigecycline are available from the CLSI guidelines. Thus, the criteria of the United States Food and Drug Administration for Enterobacteriaceae were used for tigecycline (susceptibility, ≤2 µg/mL; resistance, ≥8 µg/mL) [21]. Staphylococcus aureus (ATCC 29213) and Pseudomonas aeruginosa (ATCC 27853) were used as quality control isolates.
To assess interrelationship between MLST type and time-kill assay results, 35 isolates were randomly selected from 69 serially arranged isolates according to the isolation time. In the same isolates, genes encoding carbapenemases were detected by PCR and sequencing as described previously [23]. Nucleotide sequences obtained by PCR sequencing were compared with sequence databases using BLAST (http://blast.ncbi.nlm.nih.gov/). MLST was performed by PCR and sequencing analysis of seven housekeeping genes (gltA, gyrB, gdhB, recA, cpn60, gpi, rpoD), as described previously [23]. Nucleotide sequences obtained by PCR sequencing were compared with sequences pre-existing in the MLST databases (http://pubmlst.org/abaumannii) to assign the allelic number and sequence types (STs).
Time-kill assays were performed for the three antibiotics (colistine, doripenem, and tigecycline) and three antibiotic combinations (colistin-doripenem, doripenem-tigecycline, and colistin-tigecycline). Concentrations representative of clinically achievable levels (colistin: 2 µg/mL; doripenem: 8 µg/mL) [25] and achievable tissue levels (tigecycline: 2 µg/mL) [26] for each antibiotic were used in this study. Tubes containing Mueller-Hinton broth supplemented with the drug were inoculated with the test organism to a density of approximately 5×105 colony-forming unit (CFU)/mL in a final volume of 10 mL and incubated in a shaker incubator at 37℃. Diluted samples (100 µL) were plated on Mueller-Hinton agar plates, and the total colony count was determined after a 24 hr incubation period at 37℃. Bactericidal activity was defined as ≥3 log10 CFU/mL reduction compared with the initial inoculums [17]. Synergism was defined as ≥2 log10 CFU/mL reduction with the combination compared with the most active single agent and ≥2 log10 CFU/mL reduction below the initial inoculum at 24 hr. Antagonism was defined as ≥2 log10 CFU/mL increase with the combination compared with the most active single agent at 24 hr. Indifference was defined as <2 log10 change in CFU/mL at 24 hr with the combination compared with the most active single agent [27].
To determine whether the differences among the time-kill assay results of the three antibiotic combinations in each group were statistically significant, McNemar's test was conducted. Chi-square (χ2) test was performed to compare the differences in the results of the XDR and MDR groups for each drug combination. The SPSS 18.0 program (SPSS Inc., Chicago, IL, USA) was used, and a P value less than 0.05 was considered statistically significant.
Among the 41 XDR isolates, 51.2%, 7.3%, and 29.3% of the isolates were susceptible to colistin, doripenem, and tigecycline, respectively. Among the 28 MDR isolates, 100%, 0%, and 25% of the isolates were susceptible to colistin, doripenem and tigecycline, respectively. The MIC50 and MIC90 values and percent antimicrobial resistance are presented in Table 1.
Thirty-four (97.1%) of the 35 isolates tested for the OXA gene carried the OXA-23 gene, whereas only two (5.7%) isolates carried the ISAba-OXA-51 gene. The STs identified by MLST were divided into six groups, of which the frequencies were as follows: ST191 (45.7%), ST208 (22.9%), ST737 (20%), ST357 (5.7%), ST229 (2.9%), and ST369 (2.9%).
The time-kill assay results for all A. baumannii isolates are presented in Table 2. Colistin-doripenem showed the highest synergy rate in both the XDR (53.7%) and MDR (53.6%) groups. By contrast, doripenem-tigecycline showed the lowest synergy rate in both the XDR (14.6%) and MDR (14.3%) groups. The difference in the time-kill assay results between the XDR and MDR groups with regard to all antibiotic combinations are shown in Table 3. The significant difference of the time-kill assay results was found between following antibiotic combinations: colistin-doripenem vs. doripenem-tigecycline (P=0.000); colistin-tigecycline vs. doripenem-tigecycline (P=0.000) in the XDR group and colistin-doripenem vs. doripenem-tigecycline (P=0.003) in the MDR group (Table 3). However, no significant difference was found between the time-kill assay results of the XDR and MDR group for each drug combination. Antagonism was observed for doripenem-tigecycline and colistin-tigecycline combinations. Among the total number of isolates, doripenem-tigecycline showed a relatively high percentage (5.8%) of antagonism compared with that of colistin-tigecycline (1.4%).
The rate of bactericidal activity increased from 23.2% for the most active single agent to 75.4% for the colistin-doripenem combination among the total number ofisolates. The rate of bactericidal activity increased from 11.6% for the best single agent to 24.6% for the doripenem-tigecycline combination. The rate of bactericidal activity rose from 23.2% for the single most active agent to 63.8% for the colistin-tigecycline combination.
Several in vitro studies have reported synergy rates against A. baumannii for the colistin-doripenem, colistin-tigecycline, and doripenem-tigecycline combinations as 33.3-100%, 8.3-100%, and 33.3%, respectively [151722282930].
In our study, antibiotic combinations were found to be superior to monotherapy, with regard to bactericidal activity in the XDR and MDR groups. In previous studies, the colistin-doripenem combination was reported to have a better therapeutic effect than the colistin-tigecycline combination against XDR-AB infection [3132]. However, no significant difference between the two combinations was found in our study. In agreement with the previous results [33], the synergy rate of the doripenem-tigecycline combination was found to be low (14.6%) in the present study. Several in vitro studies of XDR-AB isolates have shown higher synergy rates (72-100%) for the colistin-doripenem and colistin-tigecycline combinations compared with our results (43.9-53.7%) [253435].
Although the exact causes for the different synergy rates between various studies are unknown, they may be attributable to differences in sample size, regional epidemiologic features, test methods used, and interpretation criteria for the synergy. A previous study revealed that synergy rates vary according to the test method used (time-kill assay, E test, chequerboard test) [36]. In addition, the synergy rate differed between studies because each used different interpretation criteria for synergy. For example, Principe et al. [17] admitted synergistic activity at any time the criteria for synergy were fulfilled, whereas the present study admitted synergy at 24 hr post-inoculation. Applying the criteria for synergy in the present study converted all four synergy cases discussed by Principe et al. [17] into indifference cases because synergy was not found at 24 hr. Appropriate comparison of the synergy rates in different studies requires the establishment of a standard protocol, including common interpretation criteria.
Dinc et al. [16] reported that the doripenem-tigecycline combination was more effective than the colistin-doripenem combination in their in vivo study. In contrast, doripenem-tigecycline showed the lowest synergy rate in the MDR groups in our study. It is known that in vitro study results are not always similar to those of in vivo studies, because in vivo environments cannot be completely mimicked in vitro [36]. Therefore, the results obtained from in vitro studies need to be supported by further in vivo studies before these results are used to inform clinical practice.
In the present study, antagonism was detected for doripenem-tigecycline and colistin-tigecycline combinations in the total number of isolates, and antagonistic rates varied from 0% to 10.7% (Table 3). In particular, the doripenem-tigecycline combination showed the highest antagonistic activity in the MDR group. In contrast, antagonism was not detected against A. baumanii isolates with the colistin, doripenem, and tigecycline combinations in most previous studies [15172225293435]. Thus far, the tigecycline-piperacillin/tazobactam, tigecycline-amikacin, and colistin-tigecycline combinations have shown antagonistic activity [30]. However, the underlying cause of the antagonism demonstrated in the use of combinations of antibiotics against A. baumannii isolates has not been clearly identified.
Such dissimilar or even discrepant results have also been reported in the field of drug interaction [3738]. Although the reasons for such differences are not clear, some contributing factors or possible causes have been postulated. For example, the concentration of drug components used and the ratio in which the two drug components co-exist may affect the results of drug interaction. Other possible contributing or influential factors for the differing interactions include differences in species, strain, the time required for whole test procedure, the read-outs for growth in culture, infection, dosing regimens, and efficacy criteria in animal models used in each study [37]. Although we could not fully explain the reasons for these differences in the rates of synergism or antagonism in the present study and most time-kill assay studies, we suspect differences in the concentrations of drugs used to be the main cause of the different results. Most studies for drug interaction have tested diverse concentrations of drugs ranging from 1/4×MIC to 4×MIC of each isolates, usually using small number of bacterial isolates. We have tested a single achievable serum concentration for each drug using larger number of isolates. Taken as a whole, these findings underscore the importance of standardization of isolates, methods, and analysis to identify the worthiest combinations of drugs recognized previously [37]. Further studies such as comparison studies of diverse methods implementing standardized protocols may help to explain such differences in the future.
The frequencies of synergy were similar among different ST clusters of A. baumannii in this study (data not shown). Because the results of the time-kill assay of isolates belonging to the same ST clusters vary from isolate to isolate, it may be necessary to perform the synergy test individually for each isolate.
In summary, the colistin-doripenem combination showed the highest rate of synergy and bactericidal activity and no antagonistic reaction in XDR- and MDR-AB. Since the effects of colistin-tigecycline and doripenem-tigecycline combinations were not only synergistic but also antagonistic, it is important to check possible interactions between candidate drugs before adopting combination therapy. The present data may provide useful information for clinicians while considering optimal treatment options for MDR-AB infections.
Acknowledgments
This research was supported by Basic Science Research Program through the National Research Foundation of Korea (NRF) funded by the Ministry of Education, Science and Technology (grant number:NRF-2011-0025617). This study was supported by research fund from Chosun University, 2015.
References
1. Perez F, Hujer AM, Hujer KM, Decker BK, Rather PN, Bonomo RA. Global challenge of multidrug-resistant Acinetobacter baumannii. Antimicrob Agents Chemother. 2007; 51:3471–3484. PMID: 17646423.
2. Appleman MD, Belzberg H, Citron DM, Heseltine PN, Yellin AE, Murray J, et al. In vitro activities of nontraditional antimicrobials against multiresistant Acinetobacter baumannii strains isolated in an intensive care unit outbreak. Antimicrob Agents Chemother. 2000; 44:1035–1040. PMID: 10722508.
3. Sunenshine RH, Wright MO, Maragakis LL, Harris AD, Song X, Hebden J, et al. Multidrug-resistant Acinetobacter infection mortality rate and length of hospitalization. Emerg Infect Dis. 2007; 13:97–103. PMID: 17370521.
4. Fishbain J, Peleg AY. Treatment of Acinetobacter infections. Clin Infect Dis. 2010; 51:79–84. PMID: 20504234.
5. Mera RM, Miller LA, Amrine-Madsen H, Sahm DF. Acinetobacter baumannii 2002-2008: increase of carbapenem-associated multiclass resistance in the United States. Microb Drug Resist. 2010; 16:209–215. PMID: 20707714.
6. Higgins PG, Dammhayn C, Hackel M, Seifert H. Global spread of carbapenem-resistant Acinetobacter baumannii. J Antimicrob Chemother. 2010; 65:233–238. PMID: 19996144.
7. Poirel L, Nordmann P. Carbapenem resistance in Acinetobacter baumannii: mechanisms and epidemiology. Clin Microbiol Infect. 2006; 12:826–836. PMID: 16882287.
8. Maragakis LL, Perl TM. Acinetobacter baumannii: epidemiology, antimicrobial resistance, and treatment options. Clin Infect Dis. 2008; 46:1254–1263. PMID: 18444865.
9. Karageorgopoulos DE, Kelesidis T, Kelesidis I, Falagas ME. Tigecycline for the treatment of multidrug-resistant (including carbapenem-resistant) Acinetobacter infections: a review of the scientific evidence. J Antimicrob Chemother. 2008; 62:45–55. PMID: 18436554.
10. Gordon NC, Wareham DW. Multidrug-resistant Acinetobacter baumannii: mechanisms of virulence and resistance. Int J Antimicrob Agents. 2010; 35:219–226. PMID: 20047818.
11. Peleg AY, Seifert H, Paterson DL. Acinetobacter baumannii: emergence of a successful pathogen. Clin Microbiol Rev. 2008; 21:538–582. PMID: 18625687.
12. Park YK, Jung SI, Park KH, Cheong HS, Peck KR, Song JH, et al. Independent emergence of colistin-resistant Acinetobacter spp. isolates from Korea. Diagn Microbiol Infect Dis. 2009; 64:43–51. PMID: 19362258.
13. Cai Y, Chai D, Wang R, Liang B, Bai N. Colistin resistance of Acinetobacter baumannii: clinical reports, mechanisms and antimicrobial strategies. J Antimicrob Chemother. 2012; 67:1607–1615. PMID: 22441575.
14. Navon-Venezia S, Leavitt A, Carmeli Y. High tigecycline resistance in multidrug-resistant Acinetobacter baumannii. J Antimicrob Chemother. 2007; 59:772–774. PMID: 17353223.
15. Pankuch GA, Seifert H, Appelbaum PC. Activity of doripenem with and without levofloxacin, amikacin, and colistin against Pseudomonas aeruginosa and Acinetobacter baumannii. Diagn Microbiol Infect Dis. 2010; 67:191–197. PMID: 20338710.
16. Dinc G, Demiraslan H, Elmali F, Ahmed SS, Alp E, Doganay M. Antimicrobial efficacy of doripenem and its combinations with sulbactam, amikacin, colistin, tigecycline in experimental sepsis of carbapenem-resistant Acinetobacter baumannii. New Microbiol. 2015; 38:67–73. PMID: 25742149.
17. Principe L, Capone A, Mazzarelli A, D'Arezzo S, Bordi E, Di Caro A, et al. In vitro activity of doripenem in combination with various antimicrobials against multidrug-resistant Acinetobacter baumannii: possible options for the treatment of complicated infection. Microb Drug Resist. 2013; 19:407–414. PMID: 23659601.
18. KARMS. Korean Antimicrobial Resistance Monitoring System. KCDC;2013.
19. Lee MJ, Jang SJ, Li XM, Park G, Kook JK, Kim MJ, et al. Comparison of rpoB gene sequencing, 16S rRNA gene sequencing, gyrB multiplex PCR, and the VITEK2 system for identification of Acinetobacter clinical isolates. Diagn Microbiol Infect Dis. 2014; 78:29–34. PMID: 24157058.
20. Magiorakos AP, Srinivasan A, Carey RB, Carmeli Y, Falagas ME, Giske CG, et al. Multidrug-resistant, extensively drug-resistant and pandrug-resistant bacteria: an international expert proposal for interim standard definitions for acquired resistance. Clin Microbiol Infect. 2012; 18:268–281. PMID: 21793988.


21. Clinical and Laboratory Standards Institute. Performance standards for antimicrobial susceptibility testing, 25th Informational supplement, M100-S25. Wayne, PA: Clinical and Laboratory Standards Institute;2015.
22. Peck KR, Kim MJ, Choi JY, Kim HS, Kang CI, Cho YK, et al. In vitro time-kill studies of antimicrobial agents against blood isolates of imipenem-resistant Acinetobacter baumannii, including colistin- or tigecycline-resistant isolates. J Med Microbiol. 2012; 61:353–360. PMID: 22016557.
23. Turton JF, Ward ME, Woodford N, Kaufmann ME, Pike R, Livermore DM, et al. The role of ISAba1 in expression of OXA carbapenemase genes in Acinetobacter baumannii. FEMS Microbiol Lett. 2006; 258:72–77. PMID: 16630258.
24. Bartual SG, Seifert H, Hippler C, Luzon MA, Wisplinghoff H, Rodriguez-Valera F. Development of a multilocus sequence typing scheme for characterization of clinical isolates of Acinetobacter baumannii. J Clin Microbiol. 2005; 43:4382–4390. PMID: 16145081.
25. Oleksiuk LM, Nguyen MH, Press EG, Updike CL, O'Hara JA, Doi Y, et al. In vitro responses of Acinetobacter baumannii to two- and three-drug combinations following exposure to colistin and doripenem. Antimicrob Agents Chemother. 2014; 58:1195–1199. PMID: 24247139.
26. Tan TY, Lim TP, Lee WH, Sasikala S, Hsu LY, Kwa AL. In vitro antibiotic synergy in extensively drug-resistant Acinetobacter baumannii: the effect of testing by time-kill, checkerboard, and Etest methods. Antimicrob Agents Chemother. 2011; 55:436–438. PMID: 20956606.
27. Entenza JM, Moreillon P. Tigecycline in combination with other antimicrobials: a review of in vitro, animal and case report studies. Int J Antimicrob Agents. 2009; 34:8.e1–8.e9. PMID: 19162449.


28. Ni W, Cui J, Liang B, Cai Y, Bai N, Cai X, et al. In vitro effects of tigecycline in combination with colistin (polymyxin E) and sulbactam against multidrug-resistant Acinetobacter baumannii. J Antibiot (Tokyo). 2013; 66:705–708. PMID: 23981963.
29. Karaoglan I, Zer Y, Bosnak VK, Mete AO, Namiduru M. In vitro synergistic activity of colistin with tigecycline or β-lactam antibiotic/β-lactamase inhibitor combinations against carbapenem-resistant Acinetobacter baumannii. J Int Med Res. 2013; 41:1830–1837. PMID: 24265334.
30. Principe L, D'Arezzo S, Capone A, Petrosillo N, Visca P. In vitro activity of tigecycline in combination with various antimicrobials against multidrug resistant Acinetobacter baumannii. Ann Clin Microbiol Antimicrob. 2009; 8:18. PMID: 19460166.


31. Shields RK, Kwak EJ, Potoski BA, Doi Y, Adams-Haduch JM, Silviera FP, et al. High mortality rates among solid organ transplant recipients infected with extensively drug-resistant Acinetobacter baumannii: using in vitro antibiotic combination testing to identify the combination of a carbapenem and colistin as an effective treatment regimen. Diagn Microbiol Infect Dis. 2011; 70:246–252. PMID: 21353436.
32. Shields RK, Clancy CJ, Gillis LM, Kwak EJ, Silveira FP, Massih RC, et al. Epidemiology, clinical characteristics and outcomes of extensively drug-resistant Acinetobacter baumannii infections among solid organ transplant recipients. PLoS One. 2012; 7:e52349. PMID: 23285002.
33. Clock SA, Tabibi S, Alba L, Kubin CJ, Whittier S, Saiman L. In vitro activity of doripenem alone and in multi-agent combinations against extensively drug-resistant Acinetobacter baumannii and Klebsiella pneumoniae. Diagn Microbiol Infect Dis. 2013; 76:343–346. PMID: 23601454.
34. O'Hara JA, Ambe LA, Casella LG, Townsend BM, Pelletier MR, Ernst RK, et al. Activities of vancomycin-containing regimens against colistin-resistant Acinetobacter baumannii clinical strains. Antimicrob Agents Chemother. 2013; 57:2103–2108. PMID: 23422916.
35. Dizbay M, Tozlu DK, Cirak MY, Isik Y, Ozdemir K, Arman D. In vitro synergistic activity of tigecycline and colistin against XDR-Acinetobacter baumannii. J Antibiot (Tokyo). 2010; 63:51–53. PMID: 19942947.
36. Ni W, Shao X, Di X, Cui J, Wang R, Liu Y. In vitro synergy of polymyxins with other antibiotics for Acinetobacter baumannii: a systematic review and meta-analysis. Int J Antimicrob Agents. 2015; 45:8–18. PMID: 25465524.
37. Bell A. Antimalarial drug synergism and antagonism: mechanistic and clinical significance. FEMS Microbiol Lett. 2005; 253:171–184. PMID: 16243458.


38. Watine J, Seigneuric B, Charet JC. In vitro quinolone-gentamicin antagonism demonstrated by time-kill and combination experiments with clinical isolates of Enterococcus. J Infect Chemother. 1997; 3:55–57.
Table 1
MIC results of colistin, doripenem, and tigecycline for 41 XDR and 28 MDR clinical isolates of A. baumannii
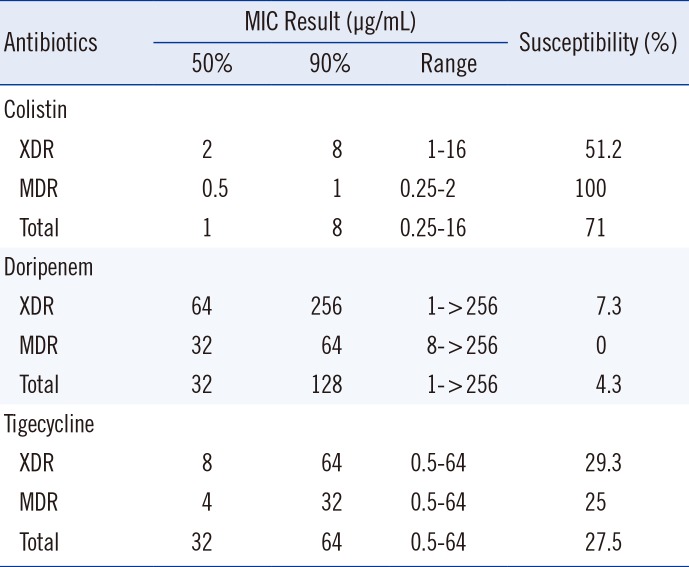
Table 2
Results of time-kill assay and bactericidal activity against two-drug combinations of colistin, doripenem, and tigecycline of 41 XDR and 28 MDR clinical isolates of A. baumannii
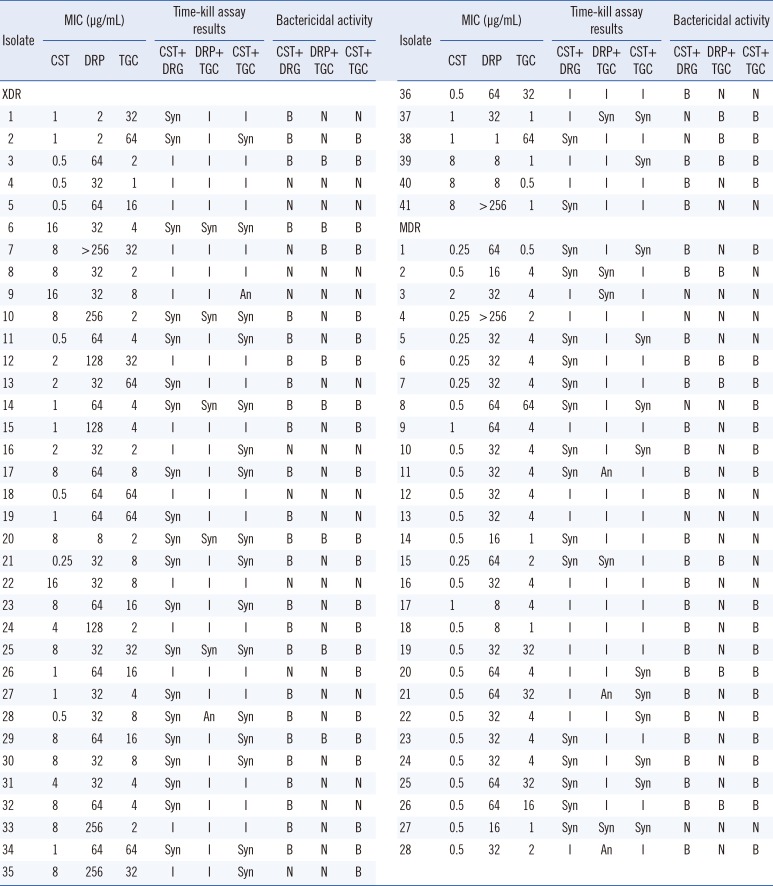
Table 3
Comparison of time-kill assay results between XDR and MDR groups* against two-drug combinations of colistin, doripenem, and tigecycline†
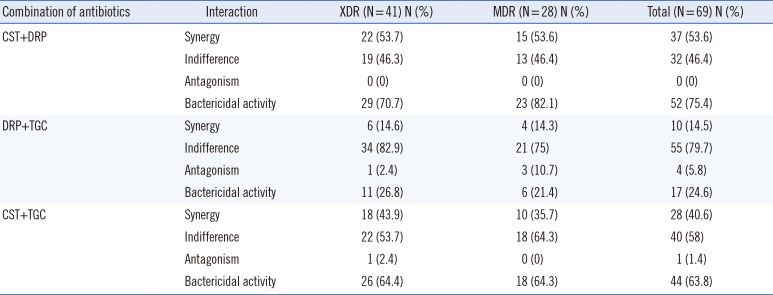
*No significant difference was found between the XDR and MDR group for each drug combination (χ2 test); †Comparison pairs showing statistically significant differences among antibiotic combinations in time-kill results: CST-DRP vs. DRP-TGC (P=0.000) and CST-TGC vs. DRP-TGC (P=0.000) in the XDR group; CST-DRP vs. DRP-TGC (P=0.003) in the MDR group (McNemar's test).
Abbreviations: XDR, extensively drug-resistant; MDR, multidrug-resistant; CST, colistin; DRP, doripenem; TGC, tigecycline.