Abstract
Background
Establishment of trimester- and assay-specific reference intervals for every population is recommended. The aim of this study was to establish a trimester- and assay-specific reference interval for thyroid-stimulating hormone (TSH) and free thyroxine (FT4) in Korean pregnant women.
Methods
From April 2012 to December 2012, 531 pregnant women receiving prenatal care and 238 age-matched, non-pregnant women were enrolled in this study. After excluding patients with pregnancy-associated complications or thyroid-specific autoantibody, 465 pregnant and 206 non-pregnant women were included. Non-parametric analysis (2.5-97.5th percentile) was performed to determine the reference interval. Levels of TSH and FT4 were determined by electrochemiluminescence immunoassay (Elecsys thyroid tests, Roche Diagnostics, Germany).
Results
The TSH reference intervals were 0.01-4.10, 0.01-4.26, and 0.15-4.57 mIU/L for the first, second, and third trimester, respectively. From the first trimester to the third trimester, the median TSH levels showed a significantly increasing trend (P<0.0001). The FT4 reference intervals were 0.83-1.65, 0.71-1.22, and 0.65-1.13 ng/dL for the first, second, and third trimester, respectively, showing a significantly decreasing trend (P<0.0001).
Maternal thyroid hormones play important roles in fetal development, and their dysfunction has an adverse effect on both the mother and child [1, 2, 3]. Maternal hypothyroidism can cause adverse effects on cognitive and neurological development of the fetus [4]. Therefore, accurate assessment of thyroid function during pregnancy and timely intervention are important. Significant hormonal changes and metabolic demands during pregnancy affect thyroid hormones and their binding proteins [5], making the interpretation of thyroid function tests difficult. Moreover, many factors such as ethnicity, age, type of assays, and iodine status can affect the results of thyroid function tests [6]. Although many previous studies have attempted to establish a reference interval of thyroid hormones during pregnancy, these data are difficult to extrapolate owing to variability in ethnicity and immunoassays [6, 7, 8, 9, 10, 11]. Thus, establishment of trimester- and assay-specific reference intervals in each population is recommended by several clinical guidelines [5, 12].
Serum measurements of key laboratory markers that are indicative of thyroid status, such as thyroid stimulating hormone (TSH) and free thyroxine (FT4), require a trimester-specific reference interval [13]. However, in Korea, limited data are available to help establish a trimester-specific reference interval for thyroid hormones. Therefore, in this study, we aimed at establishing a trimester- and assay-specific reference interval for TSH and FT4 in Korean pregnant women by using routine methods of analysis.
Pregnant women who visited the Department of Obstetrics and Gynecology at Konkuk University Medical Center and Cheil General Hospital and Women's Healthcare Center, Korea, from April 2012 to December 2012 for prenatal care were enrolled. The exclusion criteria were as follows: history of thyroid disease or goiter; any pregnancy-associated complications including hypertension, gestational diabetes, premature birth, or other adverse maternal-fetal outcomes; twin pregnancy; and positive thyroid-specific auto-antibody (thyroid peroxidase [TPO] or thyroglobulin [Tg] Ab). A total of 531 pregnant women were initially enrolled, of which 7 were excluded because of diabetes, hypertension, abortion, or twin pregnancy. In addition, 59 women were excluded because of positive TPO or Tg Ab. Therefore, the remaining 465 pregnant women were included for analysis. The median gestational ages (range) of these women at the first, second, and third trimesters were 12.1 (6.3-13.7), 16.6 (14.4-27.7), and 39.6 (28.1-41.8) weeks, respectively. A total of 238 age-matched, healthy, non-pregnant women who visited the health care center for a general examination were used as controls.
We used residual serum specimens for thyroid tests after routine laboratory tests were completed for general examination. Of the 238 non-pregnant women, 206 were negative for both TPO and Tg Ab. Therefore, they were included for determination of reference intervals. The rates of Tg Ab positivity in non-pregnant patients and in pregnant patients at the first, second, and third trimesters were 11.3%, 12.3%, 5.5%, and 4.9%, respectively, and the TPO Ab-positive rates were 11.6%, 8.5%, 4.2%, and 8.2%, respectively. Median ages of non-pregnant women and women at the first, second, and third trimesters were 32, 33, 32, and 33 yr, respectively, with no significant difference. The study protocol was approved by the Institutional Review Boards of Konkuk University Medical Center and Cheil General Hospital and Women's Healthcare Center, Korea.
Blood samples were collected from the controls in the morning after an overnight fast. However, fasting was not mandatory in pregnant patients. Samples were centrifuged at 2,500 g for 10 min within 1 hr after collection. Serum was stored at -70℃ until analysis. The levels of TSH, FT4, TPO, and Tg Ab were determined by using electrochemiluminescence immunoassay (Elecsys thyroid tests, Roche Diagnostics, Mannheim, Germany), according to the manufacturer's instructions. Patients were considered positive for TPO and Tg Ab, if their TPO and Tg Ab levels were >35 IU/mL and >115 IU/mL, respectively. To determine the difference between the assays, 55 specimens of pregnant women were additionally analyzed for TSH and FT4 levels, by using ADVIA Centaur Assay (Siemens Healthcare Diagnostics Inc., Tarrytown, NY, USA).
The reference interval was calculated according to the guidelines of the Clinical and Laboratory Standards Institute. After excluding outliers by Turkey method, the data set was subjected to non-parametric analysis (2.5-97.5th percentile interval) [14]. The relationships between the continuous variables of the different groups were analyzed by using ANOVA. The significance of categorical variables was assessed by using the Chi-square test. Pearson's correlation coefficient test was used to compare Elecsys and ADVIA Centaur assay for TSH and FT4. The r coefficients ≤0.35 were considered to represent low or weak correlations; 0.36-0.67 modest or moderate correlations; and 0.68-1.0 strong or high correlations with r coefficients ≥0.90 very high correlations [15]. Bland-Altman plot was used to calculate mean difference and 95% limits of agreement of two assays to assess the degree and trend of difference.
Statistical analysis was performed by using PAWS Statistics software, version 17.0 (SPSS Inc., Chicago, IL, USA) and MedCalc Statistical software 12.3.0.0 (Mariakerke, Belgium). P values <0.05 were considered statistically significant.
The TSH reference intervals were 0.01-4.10, 0.01-4.26, and 0.15-4.57 mIU/L at the first, the second, and the third trimester of pregnancy, respectively. In the non-pregnant, age-matched control women, the TSH reference interval was 0.56-4.98 mIU/L. The FT4 reference intervals were 0.83-1.65, 0.71-1.22, and 0.65-1.13 ng/dL at the first, the second, and the third trimester, respectively. In non-pregnant, age-matched controls, the FT4 reference interval was 0.94-1.50 ng/dL.
Analysis of the median values of FT4 and TSH revealed significant differences between trimesters for both TSH and FT4 values (P<0.001). The median TSH level during the first trimester significantly decreased as compared to that of non-pregnant controls (P<0.001). In addition, a significantly increasing trend of TSH level from the first to the third trimester was observed, which reached levels similar to those observed in non-pregnant controls (P<0.001). The median FT4 level showed a significantly decreasing trend with an increase in gestational age, which was lowest in the third trimester (P<0.001) (Fig. 1).
The correlation coefficient between Elecsys and ADVIA Centaur was higher for TSH than for FT4 (R=0.9930 and 0.8025, respectively). The between-assay bias was evaluated by Bland-Altman plots. The TSH concentrations obtained by Elecsys were higher on average than those obtained by ADVIA Centaur (mean difference, +0.31 mIU/L; % difference, +17.5%). In contrast, the FT4 levels obtained by Elecsys were lower on average than those obtained by ADVIA Centaur (mean difference, -0.21 ng/dL; % difference, -20.2%) (Fig. 2).
Pregnancy can cause significant changes in thyroid function [5]. Increases of thyroxine-binding globulin and human chorionic gonadotropin are known to be associated with thyroid function during pregnancy. An increase in the thyroxine-binding globulin levels can increase the T4 and triiodothyronine levels by up to 50%, which can result in an increase of up to 50% in daily iodine requirement. An increase in the human chorionic gonadotropin level is associated with a decreased serum TSH level during pregnancy, especially during the first trimester [16]. Levels of FT4 together with free triiodothyronine have been reported to peak during the first trimester and decline thereafter (during the second and third trimester) to 20-40% below the normal level [5, 6].
In this study, the levels of TSH and FT4 were significantly different between trimesters in pregnant women as compared to the levels in non-pregnant controls. The current report confirmed previous results that demonstrated clear trends of increasing TSH and decreasing FT4 levels from the first to the third trimester, although a different trend for TSH has been reported [17]. Nevertheless, the lower TSH level in the first trimester remains poorly understood. The reported upper limits of TSH vary across studies. Guidelines from the American Thyroid Association suggest that trimester-specific reference intervals for TSH should be used in every population [5]. When trimester-specific reference intervals are not available, the following reference intervals can be used: first trimester, 0.1-2.5 mIU/L; second trimester, 0.2-3.0 mIU/L; and third trimester, 0.3-3.0 mIU/L [5].
In this study, the reference interval of TSH was wider than that obtained from other studies and American Thyroid Association guidelines. The lower limits (2.5th percentile) of TSH in the first and second trimesters were very low (0.01 mIU/L) in the current study and previous studies [6, 18, 19, 20]. Therefore, using non-pregnant reference intervals can lead to misclassification of normal pregnant women with low TSH levels [6]. Furthermore, the upper limit (97.5th percentile) of TSH in this study was higher than that reported in other studies (Table 2). The serum TSH in African-Americans is known to be lower than that in Caucasians. The National Health and Nutrition Examination Survey III report also showed a significant decrease in the median TSH levels in African-Americans compared to Caucasians [21, 22, 23].
For Asian populations, there are relatively less data, and available data show variable results. In general, the upper limits of TSH were higher in the Asian population than in the African-American population [6, 9, 11, 17, 24, 25]. A study by Marwaha et al. [11] in India reported the highest upper limit of reference intervals for TSH. In addition, Chinese studies have reported higher values than Caucasian studies [6, 9]. However, another Chinese study reported a lower limit for TSH [18]. Therefore, different methodologies can generate conflicting results. Assays in ACS 180 and ADVIA Centaur studies used originally similar systems that generated close results in the assurance program and the experiment [26]. Moreover, these studies even showed different trends of TSH during pregnancy. Thus, other factors such as characteristics of the study population, selection criteria, method of reference interval estimation, and iodine status could contribute to the existent discrepancy.
The TSH upper limits reported during pregnancy in the current study were higher than those observed in Caucasian populations and comparable to those in Chinese populations, but were lower than those observed in Indian populations [6, 7, 9, 11, 19, 20, 25, 27, 28] (Table 2). Three studies-the Indian, Chinese, and current study (Korean)-using the Roche Elecsys assay showed a higher upper limit for TSH than those of other studies, which could be partly explained by the combined effects of ethnicity and methodology. Indeed, the current study revealed that TSH concentrations obtained by Elecsys showed higher values than those obtained by ADVIA Centaur (mean difference, +0.31 mIU/L; percentage difference, +17.5%).
In a study on the role of ethnicity in thyroid tests, the reason for a higher prevalence of positive results for TPO or Tg Ab in the Asian populations was suggested to be related to a higher age of Asian pregnant women (30 yr) than African-American pregnant women (24 yr) [24]. However, we excluded antibody-positive pregnant women for reference interval determination. Furthermore, the upper limit for TSH was similar among younger (<30 yr) pregnant women when they were analyzed separately.
Reference intervals for FT4 in this study were comparable to those in other studies and those from the manufacturer (Table 2). However, most FT4 immunoassays are known to have significant bias related to the binding proteins in a method-specific manner [29]. Our study also showed a lower correlation coefficient between Elecsys and ADVIA Centaur for FT4 (R=0.8025) than TSH, which showed very high correlation between the assays (R=0.9930). The recommended method to assess serum FT4 levels during pregnancy is liquid chromatography/tandem mass spectrometry (LC/MS/MS) [5]. If LC/MS/MS is not available, method-specific reference intervals for FT4 are required [5]. Nonetheless, the differences between thyroid assays remains an issue of concern [29, 30], and expert groups are focusing on harmonizing and standardizing thyroid function tests [31, 32].
A summary of the reported reference intervals for TSH and FT4 according to populations and type of assays is provided in Table 2. In addition to ethnicity and types of assays, the selection criteria, cut-off of reference interval (percentiles used), and number of subjects varied across assays. Therefore, direct comparison was not feasible. Because most manufactures provide the reference intervals for TSH and FT4 for non-pregnant women or Caucasian pregnant women, establishing a trimester- and assay-specific reference interval in Asian countries is essential. Further studies in other Asian pregnant women would be valuable to confirm the current results.
Our study has several limitations. First, the TSH and FT4 levels could not be measured serially in each individual. Therefore, our data could be influenced by inter-individual variation. In addition, fasting was not mandatory for pregnant patients in our study. Although fasting is not necessary for thyroid tests and the reference interval for pregnant women would be used regardless of the fasting status, preanalytical factors related to the fasting status may affect the results [33]. Second, we did not evaluate the iodine status by urine iodine estimation. However, daily consumption of seaweed-containing food is notably common in Korea and Japan [34]; therefore, the amount of iodine in the diet exceeded the allowance level by a large margin. Thus, we assumed iodine sufficiency in all women.
In conclusion, we established reference intervals for TSH and FT4 in Korean pregnant women. The levels of TSH and FT4 were different from those in non-pregnant women or among trimesters. Further, there were considerable variations among studies involving different ethnicities and methods of analysis. Establishing trimester-specific reference intervals in each population is essential for accurate assessment of thyroid function. These population-specific and method-specific reference intervals will be useful for screening Korean pregnant women for thyroid disease. The harmonization and standardization of thyroid assays is also an important issue.
References
1. Casey BM, Dashe JS, Wells CE, McIntire DD, Byrd W, Leveno KJ, et al. Subclinical hypothyroidism and pregnancy outcomes. Obstet Gynecol. 2005; 105:239–245. PMID: 15684146.


2. Allan WC, Haddow JE, Palomaki GE, Williams JR, Mitchell ML, Hermos RJ, et al. Maternal thyroid deficiency and pregnancy complications: implications for population screening. J Med Screen. 2000; 7:127–130. PMID: 11126160.


3. Abalovich M, Gutierrez S, Alcaraz G, Maccallini G, Garcia A, Levalle O. Overt and subclinical hypothyroidism complicating pregnancy. Thyroid. 2002; 12:63–68. PMID: 11838732.


4. Haddow JE, Palomaki GE, Allan WC, Williams JR, Knight GJ, Gagnon J, et al. Maternal thyroid deficiency during pregnancy and subsequent neuropsychological development of the child. N Engl J Med. 1999; 341:549–555. PMID: 10451459.


5. Stagnaro-Green A, Abalovich M, Alexander E, Azizi F, Mestman J, Negro R, et al. Guidelines of the American Thyroid Association for the diagnosis and management of thyroid disease during pregnancy and postpartum. Thyroid. 2011; 21:1081–1125. PMID: 21787128.


6. Yan YQ, Dong ZL, Dong L, Wang FR, Yang XM, Jin XY, et al. Trimester- and method-specific reference intervals for thyroid tests in pregnant Chinese women: methodology, euthyroid definition and iodine status can influence the setting of reference intervals. Clin Endocrinol (Oxf). 2011; 74:262–269. PMID: 21044115.


7. Karakosta P, Chatzi L, Bagkeris E, Daraki V, Alegakis D, Castanas E, et al. First- and second-trimester reference intervals for thyroid hormones during pregnancy in "Rhea" mother-child cohort, crete, greece. J Thyroid Res. 2011; 2011:490783. PMID: 22175032.


8. Azizi F, Mehran L, Amouzegar A, Delshad H, Tohidi M, Askari S, et al. Establishment of the trimester-specific reference range for free thyroxine index. Thyroid. 2013; 23:354–359. PMID: 23167270.


9. Yu B, Wang QW, Huang RP, Cao F, Zhu ZQ, Sun DC, et al. Establishment of self-sequential longitudinal reference intervals of maternal thyroid function during pregnancy. Exp Biol Med (Maywood). 2010; 235:1212–1215. PMID: 20810761.


10. Kurioka H, Takahashi K, Miyazaki K. Maternal thyroid function during pregnancy and puerperal period. Endocr J. 2005; 52:587–591. PMID: 16284437.


11. Marwaha RK, Chopra S, Gopalakrishnan S, Sharma B, Kanwar RS, Sastry A, et al. Establishment of reference range for thyroid hormones in normal pregnant Indian women. BJOG. 2008; 115:602–606. PMID: 18333941.


12. Baloch Z, Carayon P, Conte-Devolx B, Demers LM, Feldt-Rasmussen U, Henry JF, et al. Laboratory medicine practice guidelines. Laboratory support for the diagnosis and monitoring of thyroid disease. Thyroid. 2003; 13:3–126. PMID: 12625976.
13. Glinoer D. What happens to the normal thyroid during pregnancy? Thyroid. 1999; 9:631–635. PMID: 10447005.


14. Clinical and Laboratory Standards Institute. CLSI document C28-A. How to define and determine reference intervals in the clinical laboratory; approved guideline. Second edition. Wayne, PA: CLSI;2000.
15. Taylor R. Interpretation of the correlation coefficient: a basic review. J Diagn Med Sonogr. 1990; 1:35–39.


16. Fantz CR, Dagogo-Jack S, Ladenson JH, Gronowski AM. Thyroid function during pregnancy. Clin Chem. 1999; 45:2250–2258. PMID: 10585360.


17. Dhatt GS, Jayasundaram R, Wareth LA, Nagelkerke N, Jayasundaram K, Darwish EA, et al. Thyrotrophin and free thyroxine trimester-specific reference intervals in a mixed ethnic pregnant population in the United Arab Emirates. Clin Chim Acta. 2006; 370:147–151. PMID: 16554043.


18. Panesar NS, Li CY, Rogers MS. Reference intervals for thyroid hormones in pregnant Chinese women. Ann Clin Biochem. 2001; 38:329–332. PMID: 11471873.
19. Stricker R, Echenard M, Eberhart R, Chevailler MC, Perez V, Quinn FA, et al. Evaluation of maternal thyroid function during pregnancy: the importance of using gestational age-specific reference intervals. Eur J Endocrinol. 2007; 157:509–514. PMID: 17893266.


20. Gilbert RM, Hadlow NC, Walsh JP, Fletcher SJ, Brown SJ, Stuckey BG, et al. Assessment of thyroid function during pregnancy: first-trimester (weeks 9-13) reference intervals derived from Western Australian women. Med J Aust. 2008; 189:250–253. PMID: 18759718.


21. Surks MI, Boucai L. Age- and race-based serum thyrotropin reference limits. J Clin Endocrinol Metab. 2010; 95:496–502. PMID: 19965925.


22. Walker JA, Illions EH, Huddleston JF, Smallridge RC. Racial comparisons of thyroid function and autoimmunity during pregnancy and the postpartum period. Obstet Gynecol. 2005; 106:1365–1371. PMID: 16319264.


23. Hollowell JG, Staehling NW, Flanders WD, Hannon WH, Gunter EW, Spencer CA, et al. Serum TSH, T(4), and thyroid antibodies in the United States population (1988 to 1994): National Health and Nutrition Examination Survey (NHANES III). J Clin Endocrinol Metab. 2002; 87:489–499. PMID: 11836274.


24. La'ulu SL, Roberts WL. Second-trimester reference intervals for thyroid tests: the role of ethnicity. Clin Chem. 2007; 53:1658–1664. PMID: 17712003.
25. Mehran L, Amouzegar A, Delshad H, Askari S, Hedayati M, Amirshekari G, et al. Trimester-specific reference ranges for thyroid hormones in Iranian pregnant women. J Thyroid Res. 2013; 2013:651517. PMID: 23841018.


26. Panesar NS. TSH levels in human pregnancy. Clin Endocrinol (Oxf). 2011; 75:406–407. PMID: 21521330.


27. Springer D, Zima T, Limanova Z. Reference intervals in evaluation of maternal thyroid function during the first trimester of pregnancy. Eur J Endocrinol. 2009; 160:791–797. PMID: 19228824.


28. Lambert-Messerlian G, McClain M, Haddow JE, Palomaki GE, Canick JA, Cleary-Goldman J, et al. First- and second-trimester thyroid hormone reference data in pregnant women: a FaSTER (First- and Second-Trimester Evaluation of Risk for aneuploidy) Research Consortium study. Am J Obstet Gynecol. 2008; 199:62.e1–62.e6. PMID: 18585522.


29. Sapin R, d'Herbomez M. Free thyroxine measured by equilibrium dialysis and nine immunoassays in sera with various serum thyroxine-binding capacities. Clin Chem. 2003; 49:1531–1535. PMID: 12928239.


30. Rawlins ML, Roberts WL. Performance characteristics of six third-generation assays for thyroid-stimulating hormone. Clin Chem. 2004; 50:2338–2344. PMID: 15472032.


31. Thienpont LM, Van Uytfanghe K, Beastall G, Faix JD, Ieiri T, Miller WG, et al. Report of the IFCC Working Group for Standardization of Thyroid Function Tests; part 2: free thyroxine and free triiodothyronine. Clin Chem. 2010; 56:912–920. PMID: 20395623.


32. Thienpont LM, Van Uytfanghe K, Beastall G, Faix JD, Ieiri T, Miller WG, et al. Report of the IFCC Working Group for Standardization of Thyroid Function Tests; part 1: thyroid-stimulating hormone. Clin Chem. 2010; 56:902–911. PMID: 20395624.


33. Andersen S, Bruun NH, Pedersen KM, Laurberg P. Biologic variation is important for interpretation of thyroid function tests. Thyroid. 2003; 13:1069–1078. PMID: 14651790.


34. Yamashita S, Amino N, Shong YK. The American Thyroid Association and American Association of Clinical Endocrinologists hyperthyroidism and other causes of thyrotoxicosis guidelines: viewpoints from Japan and Korea. Thyroid. 2011; 21:577–580. PMID: 21663418.


Fig. 1
Trends of TSH (A) and FT4 (B) levels during each trimester of pregnancy and non-pregnancy. A significantly increasing trend of TSH level from the first to the third trimester was observed (P<0.001), and FT4 level showed a significantly decreasing trend with an increase in gestational age (P<0.001), which was lowest in the third trimester. The central box represents values from the lower to the upper quartile. The middle line represents the median and the bars represent the 2.5th and 97.5th percentile.
Abbreviations: TSH, thyroid stimulating hormone; FT4, free thyroxine.

Fig. 2
Bland-Altman plots showing differences between Elecsys and ADVIA Centaur in TSH (A) and FT4 (B) measurements using 55 specimens of pregnant women. Thick solid lines represent the mean bias (means of paired differences). Dashed lines represent 95% limits of agreement (bias±1.96 SD).
Abbreviations: TSH, thyroid stimulating hormone; FT4, free thyroxine.
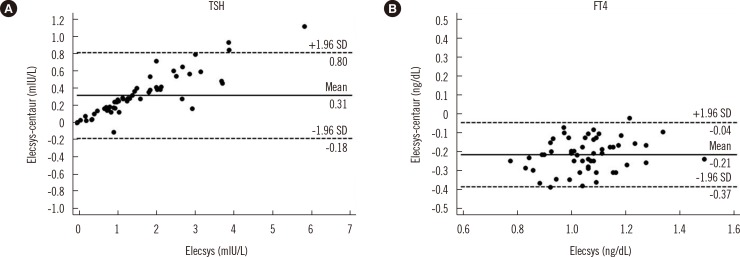