Abstract
Background
Standard protocols are lacking for the preparation of platelet lysates (PL) as an alternative to using fetal bovine serum as a cell culture supplement. This study aimed to establish optimum conditions for preparing PL for use in cell cultures.
Methods
Cell density in three pooled platelet concentrates (PC) were adjusted to 1×1012/L and 2×1012/L. PL was prepared from PC by 1 to 3 freeze-thaw (FT) cycles. HaCaT cells were cultured in media supplemented with 5% or 10% PL. Cell numbers were estimated using a Cell Counting Kit-8 (CCK-8; Dojindo Laboratories, Japan). Growth factors were quantified by using the Luminex 200 system (Luminex Corporation, USA).
Results
Cell proliferation rates in the presence of PLs were similar when prepared from PCs of both cell densities. The rates were higher in media containing 5% PL than 10% PL when prepared by two FT cycles. Concentrations of vascular endothelial growth factor (VEGF), platelet-derived growth factor-AB/BB (PDGF-AB/BB), PDGF-AA, and epidermal growth factor (EGF) were significantly higher in PL prepared from PC with a cell density of 2×1012/L than 1×1012/L PC. However, only VEGF and PDGF-AA concentrations in PLs were correlated with HaCaT cell counts.
Discovery of the release of growth factors from platelets has triggered an interest in using platelet-rich plasma (PRP) for wound healing and tissue regeneration [1-4]. Recently, platelet lysates (PL) derived from PRP have been studied for their potential advantages as a replacement for using fetal bovine serum (FBS) in cell cultures [5-11]. Studies have shown that cells cultured in the presence of FBS or fetal calf serum (FCS), when injected into the human body, produce an immune response due to the animal nature of the sera used for cultures [12]. In addition, FBS could potentially contain prions, and therefore may potentially transmit infectious diseases from animals. Platelet concentrates (PC) have the advantage of being relatively inexpensive since they are by-products of blood preparation.
Most of the studies on using PL as an FBS replacement were focused on in vitro culture and expansion of transplantable mesenchymal stromal cells for cell therapy [5-10]. PLs have also been used for culturing adipose tissue-derived stem cells, fibroblasts, and osteoblasts [13-15]. However, there are no standards for the PL used during cell cultivation, and each research has different methods of usage and production of PRP. Accordingly, it is difficult to find the best way to prepare PL in the laboratory. As the methods of producing PL involving the release of growth factors from platelet granules, PRP is activated either by adding the platelet agonists of thrombin and calcium chloride, or by repeating the freeze-thaw (FT) process. Although the former method is more effective to secrete granule from platelets than the latter method, the latter method is cost-effective, and the lysate can be conveniently stored. PL is also safe for use in humans since it is free from animal products. Although PL has been used for culturing diverse cell types, including adult stem cells, a lack of standard protocols for preparing PL has prevented its widespread use as a replacement for animal sera [5-10, 16]. Therefore, we sought to optimize the conditions for preparing PL as a substitute for FBS in cell culture.
The study protocol was approved by the institutional review board of the Hanmaum Blood Center. PC that were discarded due to high ALT levels (>65 IU/L) were obtained from the Hanmaum Blood Center.
The PC were prepared from whole blood by double centrifugation with citrate phosphate dextrose adenine-1 (CPDA-1; GCMS, Yongin, Korea) as an anticoagulant. PL was prepared by using the methods reported by Schallmoser et al. [5] and Lim et al. [17] with minor modifications. To eliminate the specific effect of individual PC in each experiment, three to fourteen concentrates of identical ABO blood types generated by pooling were used. The PC were prepared within two days of collecting blood and were stored in a platelet agitator at 22℃. After thoroughly mixing the pooled concentrates, platelet and white blood cell (WBC) counts were measured using an LH analyzer (Beckman Coulter, Fullerton, CA, USA). The pooled concentrates were then centrifuged at 2,500 g for 10 min at room temperature, the supernatant (platelet-poor plasma, PPP) was removed, and the final platelet counts were set at 1×1012/L and 2×1012/L, respectively. Three milliliters of each PPP was inoculated into Bactec PEDS PLUS (BD; Baltimore, MD, USA), and was analyzed for bacterial contamination for 5 days. Cell culture media and culture supernatants (spent media) were tested for Mycoplasma pneumonia by PCR with a commercial kit (Seegene; Seoul, Korea) following the manufacturer's instructions. Two sets of each of the pooled PPP and PRP with adjusted platelet counts were stored at -70℃ for less than one month for duplicate experiments. For preparing PL, frozen PRP was first left at room temperature for 10 min. Then, PRP was incubated at 37℃ for two hours in a water bath. The resulting lysate was centrifuged at 4,000 g for 15 min to remove platelet fragments, and the supernatant was filtered through a 0.22-µm filter. Heparin solution (0.002 U/L) (Sigma-Aldrich; St. Louis, MO, USA) was added to the supernatant before cell culture to prevent gel formation resulting from platelet activation.
The number of FT cycles was set to vary from 1 to 3. Platelet and WBC of thawed PL were counted to determine the extent of lysis caused by FT cycles. Lysed platelet counts were calculated by subtracting the platelet counts after FT from the basic platelet counts before FT. Platelet lysis was expressed as a percentage of the lysed platelets to the basic platelet count.
The human immortal keratinocyte cell line, HaCaT, was cultured in DMEM (Lonza; Walkersville, MD, USA) supplemented with 10% FBS and 2 mM L-glutamine in a humidified incubator at 37℃ and 5% CO2. In cultures using media supplemented with PL, the final concentration was adjusted to 5% or 10%. In the PL groups, cells were plated at a density of 2×108 cells/L in a 35-mm dish using serum-free DMEM. PLs that had been subjected to 1, 2, or 3 FT cycles were used for cell culture. Cells were passaged using 5% PL that had undergone one repetition of FT; cells were plated at a density of 2×108 cells/L to reach 70% to 80% confluence in two days.
The HaCaT cell proliferation rate was estimated by using Cell Counting Kit-8 (CCK-8; Dojindo Laboratories, Kumamoto, Japan) following the manufacturer's instructions. Optical density (OD) at 450 nm (formation of formazan) was measured using VERSA Max microplate reader (MDS Analytical Technologies; Sunnyvale, CA, USA) with SoftMaxPro software. Cell counts were indirectly estimated from a standard curve generated using solutions of known cell counts: 0, 1×104, 1×105, 5×105, and 1×106 cells per well. The CCK-8 ratio of HaCaT cells according to the passage number was defined as the ratio of OD450 in 5% PL at 1×1012/L to that in 5% FBS.
Concentrations of transforming growth factor-alpha (TGF-α), vascular endothelial growth factor (VEGF), platelet-derived growth factor-AB/BB (PDGF-AB/BB), PDGF-AA, and epidermal growth factor (EGF) present in PL supernatants corresponding to cell densities of 1×1012/L and 2×1012/L prepared by using 1, 2, or 3 FT cycles of PC were measured. FBS and three PPPs that had each been subjected to 1, 2, or 3 FT cycles were used as the controls. Concentrations of growth factors were determined using human growth factor/Chemokine Panel I reagent (Millipore Co., Billerica, MA, USA) and Luminex 200 system (Luminex Co., Austin, TX, USA).
Statistical analysis was performed using Microsoft Excel 2007 (Microsoft, Redmond, WA, USA) and SPSS 12.0 for Windows (SPSS Inc., Chicago, IL, USA). Experiments were conducted in duplicate and the data were expressed as the average with standard deviations. P<0.05 was considered statistically significant.
The normal distribution of data was confirmed by using the One-Sample Kolmogorov-Smirnov test. Although the number of PL samples was small, parametric statistics were used to enforce statistical power. The storage time of PC belonging to each blood type was analyzed using ANOVA with Tukey HSD as a post-hoc multiple comparison test. The effect of FT cycles on the individual PL samples was analyzed by using repeated measures ANOVA. A paired Student t-test was conducted to determine the difference between the 1×1012/L PL and 2×1012/L PL groups, as well as the difference between the 5% PL and 10% PL groups for the same PL samples. The independent Student t-test was used to determine the difference between the PPP and PL groups. Pearson's correlations were used in the correlation analysis of the concentration of growth factors and HaCaT cells.
There were three blood groups in the pooled PL samples: 10 units of blood type A, 14 units of blood type B, and 3 units of blood type O. The average time spent between whole blood collection at the blood center and the preparation of PLs before freezing was 44.6 hr. There was no significant difference in this parameter among the three pooled PL samples (43.9 hr for blood type A, 46.1 hr for blood type B, and 42.1 hr for blood type O). The average WBC count in the 2×1012/L PL group was 0.26×109/L. This count was significantly higher than that of the 1×1012/L PL group (0.14×109/L) (P=0.013). There was no significant difference in the WBC count in relation to the frequency of FT (1, 2, or 3 cycles). The blood samples were negative for M. pneumoniae and bacterial growth for five days of culture.
Compared to the counts before FT, 25% of the platelets were lysed after one FT cycle, 47% were lysed after two cycles, and 53% were lysed after three cycles. The difference in residual platelet counts in PLs prepared by varying the number of FT cycles was statistically significant (P<0.001) (Table 1).
Only the 5% PL group showed a significant difference in HaCaT cell proliferation with respect to the change in the number of FT cycles (P=0.037). There was no significant difference in HaCaT cell proliferation between the 1×1012/L PL and 2×1012/L PL groups. However, the mean HaCaT cell count per well in the 5% PL group (252,361 cells/well) was significantly higher than that in the 10% PL group (202,194 cells/well) (P=0.003), and this difference was more pronounced in the 1×1012/L PL group (P<0.001) (Table 2). The HaCaT cell count in media supplemented with 5% or 10% FBS was approximately 360,000 cells/well and 470,000 cells/well, respectively. Thus, HaCaT cells proliferated at a much slower rate in PL-supplemented media than in media containing FBS.
The difference between the observed rates of proliferation of HaCaT cells cultured in PL- or FBS-supplemented media became more pronounced as the passage number increased (Fig. 1). The CCK-8 ratios were 0.88-1.02 for the first passage, 0.48-1.15 for the second, and 0.47-1.0 for the third. There were no obvious morphological differences between HaCaT cells cultured in 5% FBS and 5% PL or between cells from different passages (Fig. 2).
Within any group, the HaCaT cell proliferation rate changed significantly with increasing passage (P=0.005 for 1×1012/L PL; P=0.002 for 2×1012/L PL; P<0.001 for both). In particular, the HaCaT cell count after three passages was higher than that estimated after one and two passages, and the counts were also higher than that of the 5% FBS group (n=6; Table 3). The average HaCaT cell count of the 5% PL group was significantly higher in the 1×1012 cells/L treatment than that in the 2×1012 cells/L treatment (P=0.01), irrespective of the passage frequency.
Among the growth factors analyzed, only TGF-α (3.4±1.9 mg/L, n=3) was detected in FBS. The concentrations of VEGF (P<0.001), PDGF-AB/BB (P<0.001), PDGF-AA (P=0.01), and EGF (P<0.001) in PL were significantly higher than those found in PPP. The concentrations of VEGF (P<0.001), PDGF-AB/BB (P<0.001), PDGF-AA (P=0.01), and EGF (P<0.001) in the 2×1012 cells/L PL group were significantly higher than those in the 1×1012 cells/L PL group (Table 4). The concentrations of VEGF (P=0.002), PDGF-AA (P=0.001), and EGF (P=0.049) positively correlated with the number of FT cycles. However, only the VEGF (r=0.485, P=0.04) and PDGF-AA (r=0.470, P=0.048) concentrations were positively correlated with the HaCaT cell count (Fig. 3).
There were significant correlations among the concentrations of growth factors: VEGF and PDGF-AB/BB (r=0.582, P=0.011), VEGF and PDGF-AA (r=0.841, P<0.001), PDGF-AB/BB and PDGF-AA (r=0.675, P=0.002), PDGF-AA and EGF (r=0.588, P=0.011), and PDGF-AB/BB and EGF (r=0.778, P<0.001).
PC contain high levels of growth factors, including PDGF, TGF, insulin like growth factor (IGF), EGF, and VEGF [4]. In our study, PLs were analyzed for the presence of five growth factors. The results revealed that only the VEGF and PDGF-AA concentrations were correlated with HaCaT cell proliferation. Positive correlations between VEGF levels, endothelial cell division, and bone formation have been reported previously [16]. Therefore, conditions that can increase the concentrations of VEGF and PDGF-AA in media supplements may augment the rate of HaCaT cell proliferation.
The concentrations of VEGF, PDGF-AB/BB, PDGF-AA, and EGF were higher in PL than in PPP. Furthermore, their concentrations were higher in the 2×1012 cells/L PL than in the 1×1012 cells/L PL. This finding indicates that a higher platelets cell density increases the concentration of VEGF and PDGF-AA in PL. Repeated FT induces the degranulation of platelet granules, thereby releasing VEGF, PDGF-AA, and EGF. Therefore, it was expected that an increase in the number of FT cycles could result in elevated concentrations of VEGF and PDGF-AA in PL. However, previous studies have shown that PL prepared from PCs with a higher platelet count was not necessarily more effective with respect to cell proliferation [8, 18]. Furthermore, this report showed that HaCaT cell proliferation did not vary with platelet count or the number of FT cycles employed for preparing PL (Table 2). In contrast, as shown in Table 3, we observed higher HaCaT cell counts in the 1×1012 cells/L PL group than in the 2×1012 cells/L PL group for all passages. In addition, HaCaT cell proliferation in the 5% PL group was faster than that in the 10% PL group. Therefore, our results demonstrate that the concentrations of PL and growth factors in culture media determine the rate of cell proliferation.
Previous studies have shown that that 10% PL is more favorable for cell culture than 5% PL [19, 20]. However, the platelet counts used in these studies, respectively 1×1011 cells/L [19] and 3×109/L [20], were lower than that used in our experiments (1×1012 cells/L). Kakudo et al. [13] found that adipose tissue-derived stem cells and human dermal fibroblasts proliferated at a higher rate in the presence of 5% activated PRP (aPRP) than in 1% aPRP. Consistent with our findings, the authors reported that the cell proliferation rate declined when aPRP (platelet cell density=1.3×1012 cells/L) concentrations in cultures were increased to 10% and 20% [13]. Another study found that the rate of cell proliferation was higher in 1.5×1012 cells/L than in 1×1012 cells/L when 5% PL was used [8]. However, this study found no differences between the cell proliferation rates at 5% and 10% PL (prepared from PC with a cell density of 2×1012 cells/L). Together with these reports, our results reveal that when the platelet concentration is lower than 1×1012 cells/L, cells tend to proliferate faster in 10% PL than in 5% PL. However, when the platelet concentration is greater than 1×1012 cells/L, a reverse trend in cell proliferation is observed. Consistent with our results, Doucet et al. [10], who used 1×1012 cells/L PL, selected 5% PL for mesenchymal stem cell cultures.
Since the cell proliferation rate was highest in 5% PL prepared by using two FT cycles from concentrates with a platelet count of 1×1012 cells/L, we conclude that under these conditions, PL serves as an effective substitute for FBS for cell cultures.
PL was reported to be more suitable for increasing cell count than FBS or FCS in bone marrow mesenchymal stem cell cultures [5, 6, 10, 19]. However, we found that proliferation of HaCaT cells belonging to the first passage was lower in PL than in media supplemented with similar concentrations of FBS. However, cells in the third passage proliferated faster in 5% PL than in 5% FBS. One study found that only human dermal fibroblasts exhibited comparable growth characteristics in the presence of FBS and serum derived from PRP, and that the proliferation of human adipose tissue stem cells was lower in PRP-derived sera than in FBS [20]. Mazzocca et al. [18] also reported that different methods for PRP separations are needed for effective proliferation of different kinds of cells, such as human muscle, bone, and tendon cells. These results demonstrated that the effect of PL on cell proliferation was cell type specific.
The method we used for the assessment of cell proliferation rate assumes that cellular dehydrogenase activity is proportional to the number of cells in cultures. This leads to the possibility that a change in the metabolic status of cell type under investigation, induced by factors present in PL or FBS, could affect the estimated rate of cell proliferation.
Various studies have used thrombin, calcium chloride [14, 21], hypotonic solution, or other platelet agonists for preparing PL. The method for preparing PL reported here is simple, economic, and safe, since only the FT process was employed for the release of growth factors from platelets. In our study, platelets were pooled and counts were set at 1×1012/L and 2×1012/L prior to freezing, thus enabling accurate assessment of the effects of platelet counts. Since platelets were frozen before counting, such an accurate assessment could not be made in previous studies [5-7].
This study has several limitations. First, we used only HaCaT cells for testing PL. Therefore it is not known whether these results can be extended to other cell types. Second, since WBC filters were not used for preparing the PRP, there was an average of 0.14×109/L (0.26×109/L) WBC in the 1×1012/L (2×1012/L) PL. Therefore, the differences between the two PL groups may have originated not only from the platelet counts but also from the WBC counts. In addition, WBC may exert a powerful influence on the release of growth factors, particularly TGF-β1 [22].
In summary, the concentrations of VEGF, PDGF-AA and EGF in PL tended to increase as platelet counts and number of FT cycles increased. However, only VEGF and PDGF-AA concentrations were correlated with HaCaT cell proliferation. There was no significant difference in the HaCaT cell proliferation between 1×1012/L PL and 2×1012/L PL, but the proliferation was greater in the 5% PL group than in the 10% PL group. Cell proliferation rate was the highest in PL that underwent two FT cycles, and we considered this condition the most favorable for preparation of PL to be used as FBS replacement in cell culture media.
References
1. Tayapongsak P, O'Brien DA, Monteiro CB, Arceo-Diaz LY. Autologous fibrin adhesive in mandibular reconstruction with particulate cancellous bone and marrow. J Oral Maxillofac Surg. 1994; 52:161–165. PMID: 8295051.


2. Eppley BL, Pietrzak WS, Blanton M. Platelet-rich plasma: a review of biology and applications in plastic surgery. Plast Reconstr Surg. 2006; 118:147e–159e.


3. Mehta S, Watson JT. Platelet rich concentrate: basic science and current clinical applications. J Orthop Trauma. 2008; 22:432–438. PMID: 18594311.


4. Baeyens W, Glineur R, Evrard L. The use of platelet concentrates: platelet-rich plasma (PRP) and platelet-rich fibrin (PRF) in bone reconstruction prior to dental implant surgery. Rev Med Brux. 2010; 31:521–527. PMID: 21290856.
5. Schallmoser K, Bartmann C, Rohde E, Reinisch A, Kashofer K, Stadelmeyer E, et al. Human platelet lysate can replace fetal bovine serum for clinical-scale expansion of functional mesenchymal stromal cells. Transfusion. 2007; 47:1436–1446. PMID: 17655588.


6. Bieback K, Hecker A, Kocaömer A, Lannert H, Schallmoser K, Strunk D, et al. Human alternatives to fetal bovine serum for the expansion of mesenchymal stromal cells from bone marrow. Stem Cells. 2009; 27:2331–2341. PMID: 19544413.


7. Reinisch A, Bartmann C, Rohde E, Schallmoser K, Bjelic-Radisic V, Lanzer G, et al. Humanized system to propagate cord blood-derived multi potent mesenchymal stromal cells for clinical application. Regen Med. 2007; 2:371–382. PMID: 17635045.
8. Lange C, Cakiroglu F, Spiess AN, Cappallo-Obermann H, Dierlamm J, Zander AR. Accelerated and safe expansion of human mesenchymal stromal cells in animal serum-free medium for transplantation and regenerative medicine. J Cell Physiol. 2007; 213:18–26. PMID: 17458897.


9. Cho HS, Song IH, Park SY, Sung MC, Ahn MW, Song KE. Individual variation in growth factor concentrations in platelet-rich plasma and its influence on human mesenchymal stem cells. Korean J Lab Med. 2011; 31:212–218. PMID: 21779198.


10. Doucet C, Ernou I, Zhang Y, Llense JR, Begot L, Holy X, et al. Platelet lysates promote mesenchymal stem cell expansion: a safety substitute for animal serum in cell-based therapy applications. J Cell Physiol. 2005; 205:228–236. PMID: 15887229.


11. Schallmoser K, Strunk D. Preparation of pooled human platelet lysate (pHPL) as an efficient supplement for animal serum-free human stem cell cultures. J Vis Exp. 2009; 32:1523. PMID: 19881465.


12. Sundin M, Ringdén O, Sundberg B, Nava S, Götherström C, Le Blanc K. No alloantibodies against mesenchymal stromal cells, but presence of anti-fetal calf serum antibodies, after transplantation in allogeneic hematopoietic stem cell recipients. Haematologica. 2007; 92:1208–1215. PMID: 17666368.


13. Kakudo N, Minakata T, Mitsui T, Kushida S, Notodihardjo FZ, Kusumoto K. Proliferation-promoting effect of platelet-rich plasma on human adipose-derived stem cells and human dermal fibroblasts. Plast Reconstr Surg. 2008; 122:1352–1360. PMID: 18971718.


14. Valeri CR, Saleem B, Ragno G. Release of platelet-derived growth factors and proliferation of fibroblasts in the releasates from platelets stored in the liquid state at 22 degrees C after stimulation with agonists. Transfusion. 2006; 46:225–229. PMID: 16441599.
15. García-Martínez O, Reyes-Botella C, Díaz-Rodríguez L, De Luna-Bertos E, Ramos-Torrecillas J, Vallecillo-Capilla MF, et al. Effect of platelet-rich plasma on growth and antigenic profile of human osteoblasts and its clinical impact. J Oral Maxillofac Surg. 2012; 70:1558–1564. PMID: 21864971.


16. Pawitan JA. Platelet rich plasma in xeno-free stem cell culture: the impact of platelet count and processing method. Curr Stem Cell Res Ther. 2012; 7:329–335. PMID: 22849700.
17. Lim YA, Baik SY, Lee WG. The effect of freezing-thawing activated platelet rich plasmas (PRP) on the proliferations of bacteria. Korean J Blood Transfus. 2011; 22:221–230.
18. Mazzocca AD, McCarthy MB, Chowaniec DM, Dugdale EM, Hansen D, Cote MP, et al. The positive effects of different platelet-rich plasma methods on human muscle, bone, and tendon cells. Am J Sports Med. 2012; 40:1742–1749. PMID: 22802273.


19. Goedecke A, Wobus M, Krech M, Münch N, Richter K, Hölig K, et al. Differential effect of platelet-rich plasma and fetal calf serum on bone marrow-derived human mesenchymal stromal cells expanded in vitro. J Tissue Eng Regen Med. 2011; 5:648–654. PMID: 21774088.


20. Kurita M, Aiba-Kojima E, Shigeura T, Matsumoto D, Suga H, Inoue K, et al. Differential effects of three preparations of human serum on expansion of various types of human cells. Plast Reconstr Surg. 2008; 122:438–448. PMID: 18626359.


21. Roussy Y, Bertrand Duchesne MP, Gagnon G. Activation of human platelet-rich plasmas: effect on growth factors release, cell division and in vivo bone formation. Clin Oral Implants Res. 2007; 18:639–648. PMID: 17590158.
22. Dohan Ehrenfest DM, Bielecki T, Jimbo R, Barbé G, Del Corso M, Inchingolo F, et al. Do the fibrin architecture and leukocyte content influence the growth factor release of platelet concentrates? An evidence-based answer comparing a pure platelet-rich plasma (P-PRP) gel and a leukocyte-and platelet-rich fibrin (L-PRF). Curr Pharm Biotechnol. 2012; 13:1145–1152. PMID: 21740377.
Fig. 1
Standard curves of Cell Counting Kit-8 (CCK-8; Dojindo Laboratories, Kumamoto, Japan) for HaCaT cell proliferation according to the frequency of passage in the 5% PL and 5% FBS groups. Optical density (OD) means the amount of formazan dye generated by the activity of dehydrogenases in living cells.
Abbreviation: FBS, fetal bovine serum.
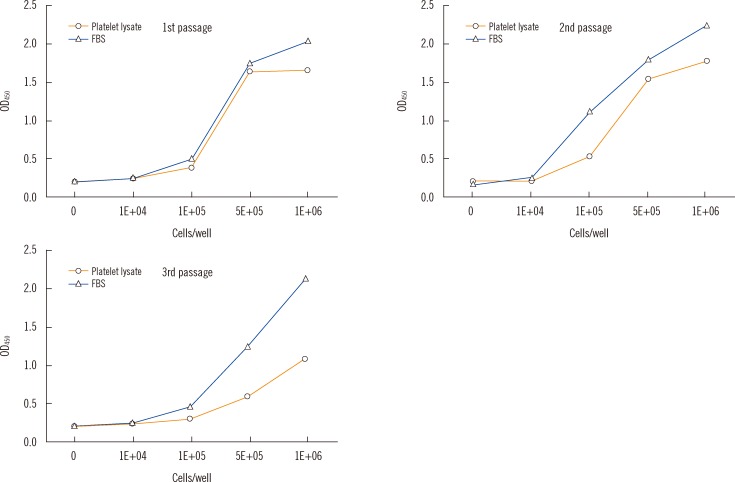
Fig. 2
HaCaT cell morphology according to the frequency of passage. There were no obvious morphological differences between HaCaT cells cultured in 5% fetal bovine serum (FBS) and 5% platelet lysate (PL) or between cells from different passages; (A) The first passage of 5% FBS. (B) The first passage of 5% PL with 1×109/mL. (C) The third passage of 5% FBS. (D) The third passage of 5% PL with 1×109/mL.
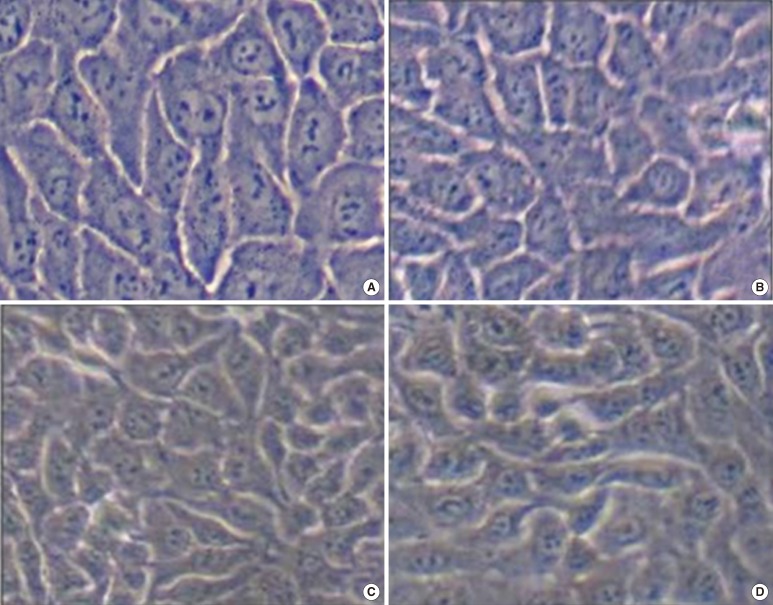
Fig. 3
Pearson's correlations between HaCaT cell count and vascular endothelial growth factor (VEGF) or platelet-derive growth factor-AA (PDGF-AA).
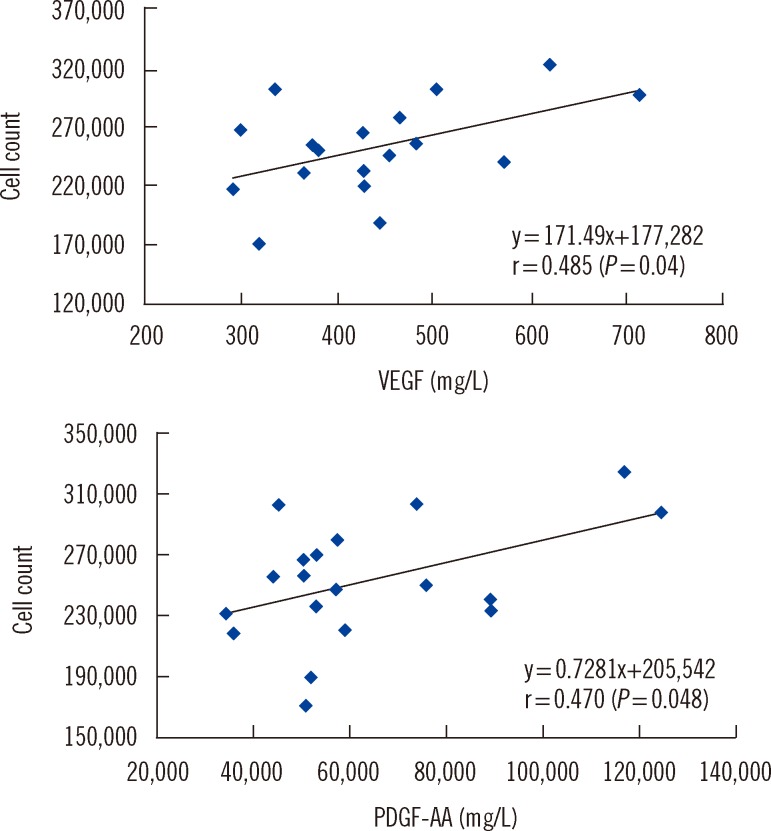
Table 2
The relationship among HaCaT cell proliferation (mean number of cells/well), the platelet count, concentration of platelet lysate (PL), and the number of freeze-thaw (FT) cycles
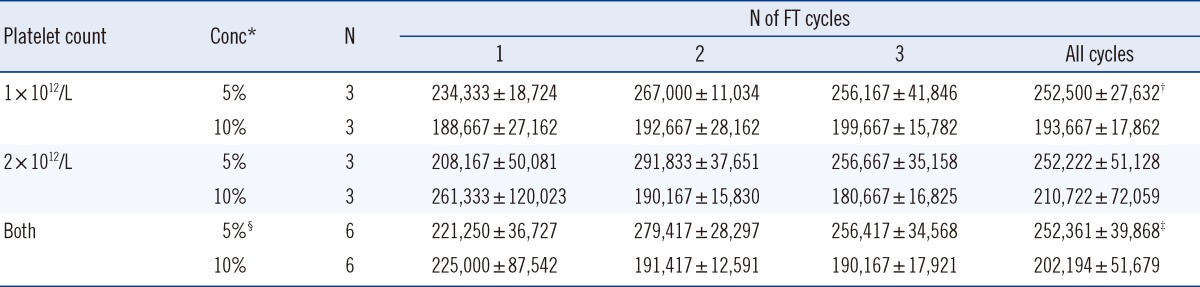
Table 3
The relationship between HaCaT cell proliferation (mean number of cells/well) and the number of passages in the 5% platelet lysate (PL) groups

Table 4
Relationship among the growth factor concentration (mg/L), the platelet count, and the number of freeze-thaw (FT) cycles in platelet lysate, platelet-poor plasma (PPP)

*P<0.01 and †P<0.001 vs. 1×1012/L by paired t test; ‡P<0.001 or §P=0.01 vs. PPP by independent t-test; the concentrations of VEGF (P=0.002), PDGF-AA (P=0.001), and EGF (P=0.049) showed significant differences at different numbers of FT cycles.
Abbreviations: PLT, platelet; TGF-α, transforming growth factor alpha; VEGF, vascular endothelial growth factor; PDGF, platelet-derived growth factor-AB/BB; PDGF, platelet-derived growth factor-AA; EGF, epidermal growth factor.