Abstract
Background
Microbiological laboratories seek technologically innovative solutions to cope with large numbers of samples and limited personnel and financial resources. One platform that has recently become available is the Kiestra Total Laboratory Automation (TLA) system (BD Kiestra B.V., the Netherlands). This fully automated sample processing system, equipped with digital imaging technology, allows superior detection of microbial growth. Combining this approach with matrix-assisted laser desorption ionization time-of-flight mass spectrometry (MS) (Bruker Daltonik, Germany) is expected to enable more rapid identification of pathogens.
Methods
Early growth detection by digital imaging using Kiestra TLA combined with MS was compared to conventional methods (CM) of detection. Accuracy and time taken for microbial identification were evaluated for the two methods in 219 clinical blood culture isolates. The possible clinical impact of earlier microbial identification was assessed according to antibiotic treatment prescription.
Results
Pathogen identification using Kiestra TLA combined with MS resulted in a 30.6 hr time gain per isolate compared to CM. Pathogens were successfully identified in 98.4% (249/253) of all tested isolates. Early microbial identification without susceptibility testing led to an adjustment of antibiotic regimen in 12% (24/200) of patients.
Conclusions
The requisite 24 hr incubation time for microbial pathogens to reach sufficient growth for susceptibility testing and identification would be shortened by the implementation of Kiestra TLA in combination with MS, compared to the use of CM. Not only can this method optimize workflow and reduce costs, but it can allow potentially life-saving switches in antibiotic regimen to be initiated sooner.
Until recently, in most microbiological laboratories, routine identification of clinically relevant bacteria and yeasts was based on conventional methods (CM), including Gram staining and culture of clinical specimens, followed by biochemical or genome-based species identification [1]. Complete identification usually takes up to two days or even longer for fastidious organisms. With ever-increasing sample loads and decreasing financial resources and personnel, there is a need for automated technologies in clinical bacteriology [2]. The time spent on inoculation of agar plates and broth by full-time technicians can be reduced by 50-70% by automation [2]. One currently available solution for total laboratory automation (TLA) is the Kiestra system (BD Kiestra B.V., Drachten, the Netherlands).
The Kiestra TLA system consists of distinct modules linked by a conveyor/track system that can be used in various combinations to create the full TLA system [3]. Digital images of agar plates are automatically produced at set time points using different lighting and exposure settings, aimed at superior detection of (early) growth. More rapid detection of microbial growth offers the possibility of initiating microbiological testing at an earlier time. This potentially benefits clinical care, and also prevents the emergence of antimicrobial resistance by faster optimization of antibiotic therapy [4]. However, no studies to date have defined a threshold of microbial growth detection using TLA to address whether and to what extent antibiotic regimens are actually adjusted when results of microbial identification become available.
In this study, Kiestra TLA was used in combination with matrix-assisted laser desorption ionization time-of-flight mass spectrometry (MS) for microbial identification in clinical specimens. Compared to CM, an automated method provides accurate, rapid, and inexpensive identification of microorganisms, although the initial cost of acquisition is considerable [3]. Three aspects of TLA relevant to clinical microbiology were evaluated. First, the speed of identification using the Kiestra TLA system in combination with MS was investigated, and a threshold of growth detection for important organisms was defined. Second, the use of Kiestra TLA in combination with MS was compared to CM in terms of accuracy and time taken for microbial identification in clinical blood culture (BC) isolates. Third, the potential clinical impact of improved speed of identification was assessed by evaluating how an earlier availability of microbial identification results might influence the prescription of antibiotic treatment.
Of 12 aerobic microbial species that frequently cause sepsis [5, 6], an exact dilution series was created at concentrations of 1×108, 1×106, 1×104, and 1×102 colony-forming units (CFU)/mL to identify the threshold of growth detection by Kiestra TLA (Table 1). Ten microliters of each dilution were inoculated per agar plate containing different media. Gram-negative rods were inoculated on Columbia sheep blood (COS), chocolate polyvitex (PVX), and CPS3 plates, while Gram-positive cocci and yeasts were inoculated on COS and PVX plates. All plates were purchased from bioMérieux Clinical Diagnostics (Marcy l'Etoile, France). Two plates per dilution and type of media were inoculated and incubated in Kiestra TLA under appropriate culture conditions. Enterobacteriaceae, non-fermenters, staphylococci, enterococci, and yeasts were incubated at 37℃; streptococci were incubated at 37℃ with addition of 5% CO2. Digital images of agar plates were automatically taken after 4, 6, 8, 10, 12, and 18 hr incubation using 10 different lighting and exposure settings to detect early microbial growth. For each strain and dilution (n=96), the minimum incubation time until detection of microbial growth in Kiestra TLA and time until sufficient material could be obtained to successfully perform subsequent MS, was calculated as the mean of all measurements at all concentrations.
Clinical isolates were recovered from blood samples incubated in the BacT/Alert 3D automated microbial detection system (bioMérieux Clinical Diagnostics). After Gram staining, isolates were inoculated on appropriate media based on the Gram staining result, incubated at 37℃ overnight, and further analyzed by CM and retrospectively by MS. Identification results obtained by the two methods were compared, and concordant results were considered definitive. Discrepancies were resolved by partial 16S ribosomal RNA (rRNA) sequencing, as described elsewhere [7, 8].
Disk-diffusion susceptibility testing was performed directly from BCs. Identification was performed with commonly used CM, e.g., in-house biochemical tests for identification of Enterobacteriaceae, Slidex Staph Plus (bioMérieux Clinical Diagnostics) for staphylococci, API 20 Strep (bioMérieux Clinical Diagnostics) for streptococci, AUXACOLOR (Sanofi Diagnostics Pasteur Inc., Lyon, France) for yeasts, etc. If the results for Enterobacteriaceae were inconclusive, automated biochemical identification was performed using VITEK2 (bioMérieux Clinical Diagnostics). If CM gave inconclusive results, 16S rRNA sequencing was performed to obtain a positive identification.
A thin film of bacteria or yeasts was deposited on an MS steel target plate and overlaid with 1 µL matrix solution (saturated solution of α-cyano-4-hydroxy-cinnamic acid in 50% acetonitrile and 2.5% trifluoroaceticacid). Each microorganism was tested in duplicate. Isolates that could not be identified directly were retested after an acetonitrile/formic acid extraction, as described elsewhere [9]. Measurements were performed with a Microflex mass spectrometer (Bruker Daltonik, Bremen, Germany) using Flex Control software (v3.0). Spectra were recorded using default settings. The spectra were imported into the MALDI Biotyper software (v2.0.43.8; Bruker Daltonik) and analyzed by standard spectra pattern matching. The results were expressed as scores ranging from 0 to 3. Scores below 1.7 were regarded as unreliable; scores ≥1.7 were considered as a reliable identification of genus; and scores ≥2.0 were deemed as a reliable identification of species. Results were considered definitive when identifications by MS in duplicate tests matched exactly, and scores were ≥2.0. In cases of discrepant results, identifications with a score ≥2.0 overruled those with a score <2.0. Isolates were retested if both scores were <2.0. If scores were <2.0 after retesting, identification was considered unsuccessful.
Logistical data were obtained for 219 consecutive positive BCs collected from patients admitted to the hospital, including the times at which results from Gram staining, pathogen identification, and susceptibility testing became available. Clinical data was also obtained, including diagnosis, types and duration of antibiotic treatment during admission, and duration of hospital stay. Specifically, a retrospective appraisal was made to determine when and if the antibiotic treatment regimen was adjusted on the basis of the availability of preliminary and final pathogen analysis results. Gram staining, pathogen identification, and susceptibility testing results of positive BCs were communicated directly to the clinician, and advice on antimicrobial therapy was given as is customary at the hospital. Results were subsequently reported in the electronic patient record system.
Statistical analyses were performed with SPSS 19.0 (SPSS Inc., Chicago, IL, USA). Descriptive statistics were used for data analysis. Categorical and continuous variables were analyzed using the chi-square test, Student t-test, or nonparametric tests to assess differences in time to microbial identification of MS and CM. P values <0.05 were regarded as significant. The normal distribution of data sets was confirmed by the Shapiro-Wilk test.
The preparation, measurement, and analysis of samples, including the time needed to perform the extraction protocol and obtain a second measurement by MS only without prior incubation in Kiestra TLA, took an average of 2.5 min per spot. Detection of microbial growth by Kiestra TLA and successful subsequent MS identification was achieved after different incubation times, depending on the species and concentrations. At higher concentrations (1×108 and 1×106 CFU/mL), MS could reliably be performed on average after 7.9 hr (95% confidence interval [CI], 7.5-8.1) of incubation, while 12.9 hr (95% CI, 12.2-13.5) were needed for lower concentrations (1×104 and 1×102 CFU/mL). At lower concentrations, 7.6% (17/223) of plates were sterile and were excluded from the analysis. Minimum incubation time at all concentrations was shortest for Gram-negative rods, with a mean of 9.6 hr (95% CI, 9.0-10.1), followed by Gram-positive cocci at 10.4 hr (95% CI, 9.6-11.2), and was longest for yeasts at 12.4 hr (95% CI, 9.4-15.5) (Table 1). On average, identification by Kiestra TLA and MS was 30.6 hr faster than identification by CM. The minimum incubation and identification time needed per isolate for CM and for Kiestra TLA combined with MS were 41.6 hr and 11.04 hr, respectively.
Of 219 consecutive positive BCs that were assessed, 10.1% contained two or more species, and 1.8% were considered false positives as no microorganisms were detected by Gram staining, acridine orange staining, or by culturing. All isolated species are listed in Table 2. From the moment that a positive BC was unloaded from the BacT/Alert system, an average time of 48.5 min (95% CI, 45-52) was required for Gram staining and inoculation on agar plates to be completed.
Of 253 clinical isolates, 239 (94.9%) were correctly identified by MS using only smear preparations. For the remaining 14 isolates, identification was repeated following an acetonitrile/formic acid extraction, which increased the overall identification rate to 98.4% (249/253). Identification by CM and MS were concordant in 97.6% of all isolates. Discrepancies were resolved by partial 16S rRNA sequencing. CM and MS identification was considered discrepant when the results differed either on genus or species level, which occurred in 2.4% of all isolates. The results of the 16S rRNA sequencing were considered as correct identification; results obtained by MS and CM were compared to 16S rRNA results and considered correctly identified or misidentified accordingly. Sequencing revealed that results obtained by MS had lower misidentification rates at the genus level (0% vs. 1.2%), while rates at the species level were higher (0.8% vs. 0.4%) (Table 2).
A mean period of 1.9 days (95% CI, 1.7-2.0) was required to complete microbial identification by CM, calculated from the time at which the technician began processing the positive BC. There was no significant difference in time required for identification between Gram-positive (mean=1.7 days; 95% CI, 1.5-1.8) and Gram-negative bacteria (mean=2.0 days; 95% CI, 1.7-2.3) (P=0.06). The identification of yeasts took significantly longer than bacterial identification with an average of 5.2 days (95% CI, 3.9-6.4) per isolate compared to 1.8 days for bacteria (95% CI, 1.6-1.9) (P=0.001).
In 14.5% of cases, the treatment regimen was adjusted after Gram-staining results became available and was not changed on availability of microbial identification results (Table 3). Availability of these results led to adjustment of the antibiotic regimen in 46.5% of patients. However, in 12% of patients, the reason for adjustment could be traced to knowledge of the pathogen identity only, since in these patients, the antibiotic susceptibility results were not yet available at the same time that identification results were reported. In 33.5% of cases, no adjustments were made in response to the availability of identification results.
The present findings demonstrate the potential for improving the effectiveness and efficiency of microbiological laboratories by implementing TLA systems. The reduction in incubation time using Kiestra TLA enables earlier identification of pathogens in clinical samples. The time required for sufficient microbial growth for successful subsequent MS analysis was longer for yeasts and lower concentrations (Fig. 1). Nevertheless, the results show that incubation time of bacterial cultures on solid media can be decreased depending on the bacterial load of the sample (Fig. 1). Moreover, with the impending addition of a large collection of yeasts to the Bruker database, the time required to identify clinically important Candida species by MS is expected to decrease substantially.
These findings confirmed the previous findings that MS is a rapid and reliable method for pathogen identification, with an overall successful identification rate of 98.4% and low misidentification rates [9-13]. The MS technology is economical, requiring few consumables, making it a favorable addition to any high-throughput system for bacteriological identification and susceptibility testing [14]. Furthermore, by combining this method with Kiestra TLA, the limitations of identification by MS can be overcome. First, an alternate system of identification in case of erroneous database entries is available, since the accuracy of identification by MS is highly dependent on the reference database [10]. Second, the Kiestra TLA system offers the possibility of performing additional tests; for instance, in case of misidentification of phylogenetically similar species such as Shigella and Escherichia. Third, it is sometimes necessary to identify pathogens not included in the database by CM.
MS can be used for the identification of strains directly from positive Bactec BC bottles (BD, Franklin Lakes, NJ, USA) [15]. However, direct MS from BacT/Alert BCs containing activated charcoal is ineffective and less reliable than using the Sepsityper kit (Bruker Daltonik) combined with the extraction procedure used in the authors' laboratory. The latter yielded positive identification rates of 51/90 (56.6%) for BacT/Alert BCs; genus and species identification was obtained for 38/90 (42.2%) samples, and genus identification only for 13/90 (14.4%) (data not shown). In staphylococci, rates of correct identification were low (9/35; 25.7%), while for Enterobacteriaceae, the rates were 100% (20/20). This is in accordance with the findings of other groups that used Sepsityper, who found significantly lower identification rates for Gram-positive (42.3-78%) compared to Gram-negative (91.5-99%) bacteria [16, 17]. Direct MS from BCs, regardless of the manufacturer, is labor-intensive and does not replace bacterial culture preparation, since this is still required in order to perform susceptibility tests and exclude bacterial mixtures. Given that 10.1% of BCs were found to contain more than one species, this is an important consideration, especially since mixed cultures are not amenable to identification by MS [15].
Some limitations were present in this study. The BC contents were not directly streaked on chromogenic agar medium for the identification of clinically relevant Candida species, since sensitivity is low even for the most commonly isolated species (e.g., 71% for Candida albicans) [18]. In addition, no comparisons were made between the combined Kiestra TLA/MS method and the direct biochemical identification from positive BCs by VITEK, since identification rates were not sufficiently high. In a study in this laboratory, 218 positive BacT/Alert BCs containing Gram-negative rods were tested directly using VITEK2. Of these, 183 contained Enterobacteriaceae, of which 145 (79.2%) were correctly identified. The remaining 35 were non-fermenters, of which 31 (88.6%) were correctly identified, resulting in an overall identification rate of only 80.7%. The overall processing time was 6.9 min per measurement, and the mean time required for identification was 5.2 hr (data not shown). Hence, only a moderate gain in time was achieved by this method, with the added disadvantage that mixed cultures and ~20% of Gram-negative bacteria could not be identified; misidentification rates for Gram-positive bacteria were even higher [19]. Since the Kiestra TLA system used in this study was not connected to an anaerobic incubator, anaerobic bacteria were not evaluated. However, in this study anaerobic bacteria were found in only a small fraction of positive BCs (3.2%) (see Table 2). Since only clinical isolates recovered from blood were used, common sepsis-causing bacteria were overrepresented in this study, which was primarily a pilot study intended to evaluate potential gain. A randomized, controlled trial comparing the use of TLA to CM is needed in order to evaluate actual benefits of an automated system in terms of morbidity, mortality, and health care costs.
Nonetheless, using automated technology such as Kiestra TLA makes timely pathogen identification by subsequent MS possible. The assessment of clinical impact (Table 3) suggested that early identification alone could lead to an antibiotic switch in 12% of cases, in addition to the 14.5% of cases, in which a switch was made on the basis of Gram staining results. Antibiotic treatment tailored to the causative pathogen is beneficial to the patient, potentially leading to reductions in morbidity and mortality, while having economic benefits such as shorter hospital stay. Numerous studies have shown that adjustment of antimicrobial therapy for bloodstream infections based on availability of antibiotic susceptibility results can improve clinical outcome [20-23].
The implementation of Kiestra TLA would shorten the first 24 hr of incubation time, which typically elapses before identification procedures and susceptibility testing are initiated. For susceptibility testing, there are benefits to using digital imaging for automatic reading and interpretation [14], since detection and interpretation of inhibition zones by Kiestra TLA can be achieved much more rapidly than by the naked eye, and standardization of prior readings is feasible. By expanding Kiestra TLA to all samples and integrating an automated colony picking system, such as MalditofA (BD Kiestra B.V.), the utility of this system will be further enhanced. With the combination of automated, continuously operating systems and rapid and reliable identification methods such as MS, laboratory workflow can be optimized, costs reduced, and pathogen identification quickened, which can lead to earlier potentially life-saving switches in antibiotic regimens for patients.
Acknowledgment
Parts of the Methods and Results sections were presented in a poster at the 21st European Congress of Clinical Microbiology and Infectious Diseases/27th International Congress of Chemotherapy (May 2011; Milan, Italy). The authors thank Dr. M. van Dijk from the Department of Health Sciences of the Free University of Amsterdam (VU) for her helpful support during the initial preparation of the manuscript.
References
1. Carroll KC, Weinstein MP. Manual and automated systems for detection and identification of microorganisms. In : Murray PR, Baron EJ, Jorgensen JH, Landry ML, Pfaller MA, editors. Manual of clinical microbiology. 9th ed. Washington, DC: American Society for Microbiology;2007. p. 192–217.
2. Greub G, Prod'hom G. Automation in clinical bacteriology: what system to choose? Clin Microbiol Infect. 2011; 17:655–660. PMID: 21521409.


3. Bourbeau PP, Ledeboer NA. Automation in clinical microbiology. J Clin Microbiol. 2013; 51:1658–1665. PMID: 23515547.


4. Huang AM, Newton D, Kunapuli A, Gandhi TN, Washer LL, Isip J, et al. Impact of rapid organism identification via matrix-assisted laser desorption/ionization time-of-flight combined with antimicrobial stewardship team intervention in adult patients with bacteremia and candidemia. Clin Infect Dis. 2013; 57:1237–1245. PMID: 23899684.


5. Dermoumi H. Fungi as pathogens in infection--results of a multicenter study. Mykosen. 1987; 30:349–354. PMID: 3313037.
6. Foltzer MA, Reese RE. Bacteremia and sepsis. In : Reese RE, Betts RF, editors. A practical approach to infectious diseases. 3rd ed. Boston: Little Brown and Company;1991. p. 19–53.
7. Grosse-Herrenthey A, Maier T, Gessler F, Schaumann R, Böhnel H, Kostrzewa M, et al. Challenging the problem of clostridial identification with matrix-assisted laser desorption and ionization-time-of-flight mass spectrometry (MALDI-TOF MS). Anaerobe. 2008; 14:242–249. PMID: 18621134.


8. Drancourt M, Berger P, Raoult D. Systematic 16S rRNA gene sequencing of atypical clinical isolates identified 27 new bacterial species associated with humans. J Clin Microbiol. 2004; 42:2197–2202. PMID: 15131188.


9. van Veen SQ, Claas EC, Kuijper EJ. High-throughput identification of bacteria and yeast by matrix-assisted laser desorption ionization-time of flight mass spectrometry in conventional medical microbiology laboratories. J Clin Microbiol. 2010; 48:900–907. PMID: 20053859.


10. Seng P, Drancourt M, Gouriet F, La Scola B, Fournier PE, Rolain JM, et al. Ongoing revolution in bacteriology: routine identification of bacteria by matrix-assisted laser desorption ionization time-of-flight mass spectrometry. Clin Infect Dis. 2009; 49:543–551. PMID: 19583519.


11. Cherkaoui A, Hibbs J, Emonet S, Tangomo M, Girard M, Francois P, et al. Comparison of two matrix-assisted laser desorption ionization-time of flight mass spectrometry methods with conventional phenotypic identification for routine identification of bacteria to the species level. J Clin Microbiol. 2010; 48:1169–1175. PMID: 20164271.


12. Eigner U, Holfelder M, Oberdorfer K, Betz-Wild U, Bertsch D, Fahr AM. Performance of a matrix-assisted laser desorption ionization-time-of-flight mass spectrometry system for the identification of bacterial isolates in the clinical routine laboratory. Clin Lab. 2009; 55:289–296. PMID: 19894408.
14. Matthews S, Deutekom J. The future of diagnostic bacteriology. Clin Microbiol Infect. 2011; 17:651–654. PMID: 21521408.


15. La Scola B, Raoult D. Direct identification of bacteria in positive blood culture bottles by matrix-assisted laser desorption ionisation time-of-flight mass spectrometry. PLoS One. 2009; 4:e8041. PMID: 19946369.


16. Klein S, Zimmermann S, Köehler C, Mischnik A, Alle W, Bode K. Integration of matrix-assisted laser desorpotion/ionization time-of-flight mass spectrometry in bloodculture diagnostic:a fast and effective approach. J Med Microbiol. 2012; 61:323–331. PMID: 22074848.
17. Loonen AJ, Jansz AR, Stalpers J, Wolffs PF, van den Brule AJ. An evaluation of three processing methods and the effect of reduced culture times for faster direct identification of pathogens from BacT/ALERT blood cultures by MALDI-TOF MS. Eur J Clin Microbiol Infect Dis. 2012; 31:1575–1583. PMID: 22080416.


18. Madhavan P, Jamal F, Chong PP, Ng KP. Identification of local clinical Candida isolates using CHROMagar Candida™ as a primary identification method for various Candida species. Trop Biomed. 2011; 28:269–274. PMID: 22041745.
19. Gherardi G, Angeletti S, Panitti M, Pompilio A, Di Bonaventura G, Crea F, et al. Comparative evaluation of the Vitek-2 Compact and Phoenix systems for rapid identification andantibiotic susceptibility testing directly from blood cultures of Gram-negative and Gram-positive isolates. Diagn Microbiol Infect Dis. 2012; 72:20–31. PMID: 22030102.
20. Harbarth S, Garbino J, Pugin J, Romand JA, Lew D, Pittet D. Inappropriate initial antimicrobial therapy and its effect on survival in a clinical trial of immunomodulating therapy for severe sepsis. Am J Med. 2003; 115:529–535. PMID: 14599631.


22. Kang CI, Kim SH, Park WB, Lee KD, Kim HB, Kim EC, et al. Bloodstream infections caused by antibiotic-resistant gram-negative bacilli: risk factors for mortality and impact of inappropriate initial antimicrobial therapy on outcome. Antimicrob Agents Chemother. 2005; 49:760–766. PMID: 15673761.


23. Micek ST, Lloyd AE, Ritchie DJ, Reichley RM, Fraser VJ, Kollef MH. Pseudomonas aeruginosa bloodstream infection: importance of appropriate initial antimicrobial treatment. Antimicrob Agents Chemother. 2005; 49:1306–1311. PMID: 15793102.
Fig. 1
Minimum incubation time required for detection of growth and successful subsequent identification by MS by strain and concentration. Error bars represent 95% CI. High, strains at higher concentrations (1×108 and 1×106 CFU/mL); low, strains at lower concentrations (1×104 and 1×102 CFU/mL).
Abbreviations: MS, matrix-assisted laser desorption ionization time-of-flight mass spectrometry; Neg, Gram-negative; Pos, Gram-positive; CI, confidence interval.
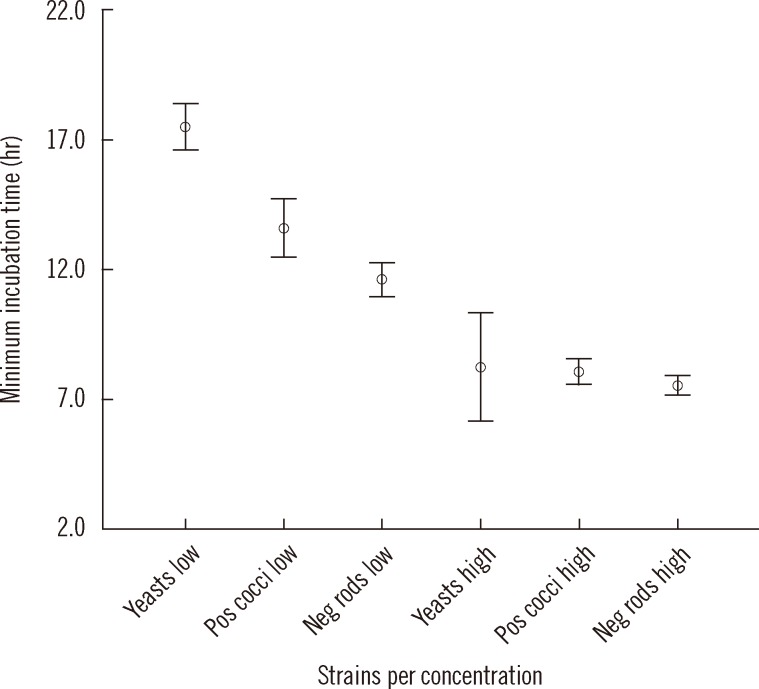
Table 1
Minimum incubation time required for detection of growth and successful subsequent identification by MS for various species
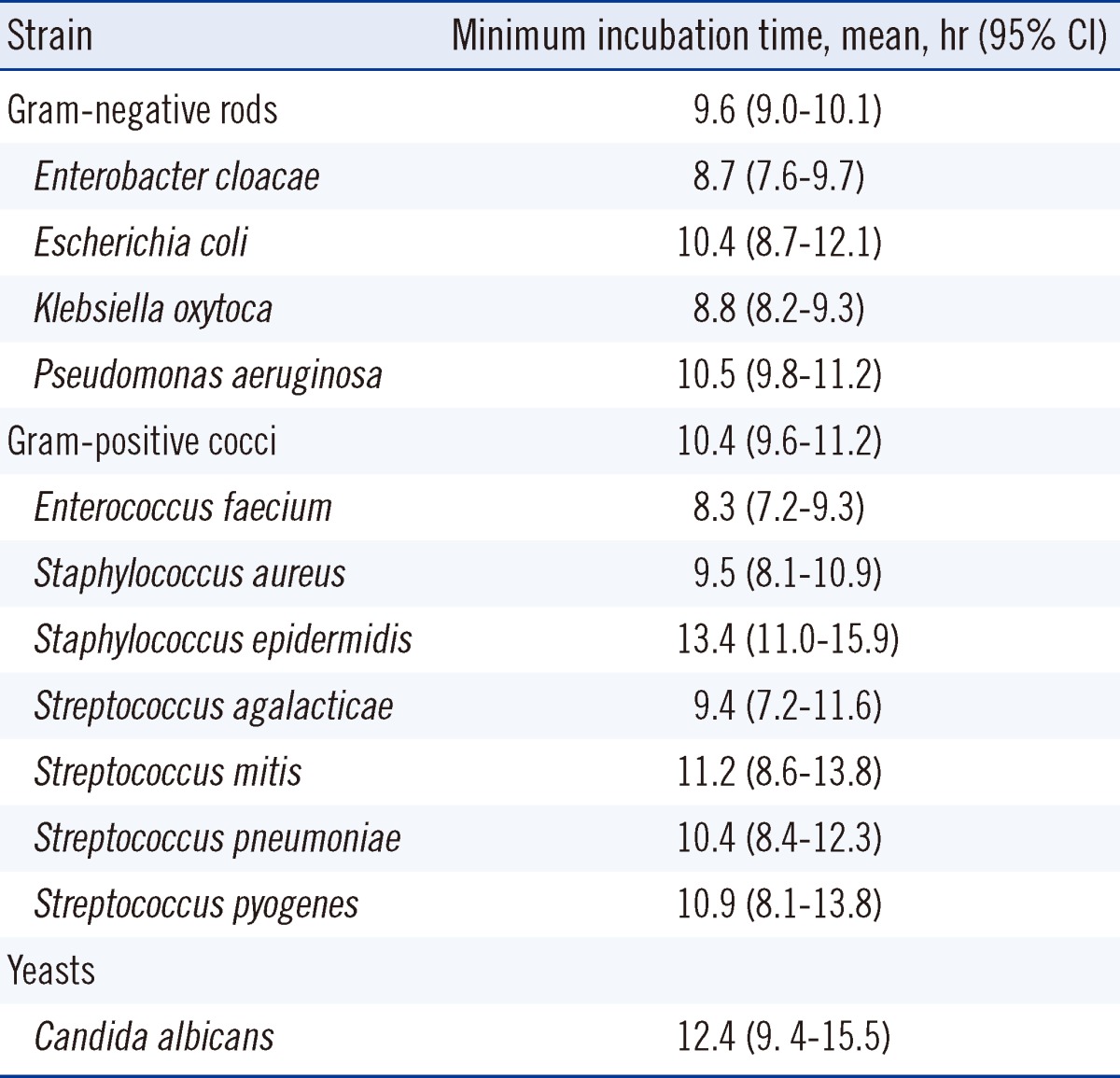