Abstract
Background
Validation of hemagglutination inhibition (HI) assays is important for evaluating antibody responses to influenza virus, and selection of erythrocytes for use in these assays is important. This study aimed to determine the correlation between receptor binding specificity and effectiveness of the HI assay for detecting antibody response to pandemic influenza H1N1 (pH1N1) virus.
Methods
Hemagglutination (HA) tests were performed using erythrocytes from 6 species. Subsequently, 8 hemagglutinating units of pH1N1 from each species were titrated by real-time reverse transcription-PCR. To investigate the effect of erythrocyte binding preference on HI antibody titers, comparisons of HI with microneutralization (MN) assays were performed.
Results
Goose erythrocytes showed most specific binding with pH1N1, while HA titers using human erythrocytes were comparable to those using turkey erythrocytes. The erythrocyte binding efficiency was shown to have an impact on antibody detection. Comparing MN titers, HI titers using turkey erythrocytes yielded the most accurate results, while those using goose erythrocytes produced the highest geometric mean titer. Human blood group O erythrocytes lacking a specific antibody yielded results most comparable to those obtained using turkey erythrocytes. Further, pre-existing antibody to pH1N1 and different erythrocyte species can distort HI assay results.
Conclusions
HI assay, using turkey and human erythrocytes, yielded the most comparable and applicable results for pH1N1 than those by MN assay, and using goose erythrocytes may lead to overestimated titers. Selection of appropriate erythrocyte species for HI assay allows construction of a more reliable database, which is essential for further investigations and control of virus epidemics.
Since March 2009 the H1N1 influenza pandemic has continued to emerge worldwide [1]. The infection with human pandemic influenza H1N1 (pH1N1) virus can cause respiratory illness, such as fever, cough, and sore throat and may cause severe symptoms in people with underlying conditions, such as heart and lung diseases, obesity, and diabetes [2]. To date, more than 18,398 individuals have died from pH1N1 infection [3]. Although the WHO has categorized pH1N1 virus as a seasonal influenza virus that continuously co-circulates with H3N2 and influenza B, observations of cross-species infection of the triple re-assortant virus have raised suspicions. Various strategies have been developed to control outbreaks and detection of virus genomic material can be performed using multiplex PCR [4, 5]; however, this method cannot be used to identify the influenza infection record after the clearance of viral particles in host cells.
The hemagglutination (HA) test is based on the HA protein's specific ability to agglutinate red blood cells, and thus facilitates quantification of viral antigen prior to performing the hemagglutination inhibition (HI) assay [6], which is one of the various laboratory techniques applied to validate antibody titer against the pH1N1 virus. The microneutralization (MN) assay, which is considered the gold standard of antibody evaluation, blocks virus infection of host cell cultures by specific binding of the HA protein to antibody. In comparison, the HI assay, which is cheaper and less laborious, relies on the HA antigen to suppress HA in serum in the presence of antibodies specific to pH1N1.
Because of the different types of glycoprotein and glycolipid composition of sialic acid (SA) receptors in each species, the influenza A virus preferentially binds to specific host erythrocytes [7-9]. Hence, human influenza A virus preferentially binds to sialyloligosaccharides containing N-acetyl neuraminic acid α-2,6-galactose (NeuAc α-2,6-Gal), while avian and equine influenza viruses favorably biwnd to oligosaccharides containing N-acetylneuraminic acid and N-glycolylneuraminic acid α-2,3-Gal, respectively [10, 11]. According to a previous study, there are more NeuAc α-2,6-Gal molecules than NeuAc α-2,3-Gal molecules on the surface of human erythrocytes (blood type O), turkey, pig, and guinea pig erythrocytes, than on those of chicken and goose erythrocytes [12, 13]. The aim of this study was to establish the correlation between receptor binding specificity and the likelihood of HA during HA tests and the effect of HA on antibody investigation by using the HI assay. To select the most appropriate erythrocytes for the HI assay, potential interference with the assay of specific erythrocyte preferences was investigated and compared with the results obtained by MN assay. We consider that the results of this study could facilitate the construction of a more accurate database, which is essential for the study and control of virus epidemics.
Four strains of virus comprising 3 strains of human pandemic virus H1N1, A/Thailand/CU-H88/2009 (CU-H88), A/Thailand/CU-B1400/2009 (CU-B1400), and A/Thailand/CU-H1492/2010 (CU-H1492) (Genbank Accession Nos., HM446345 and HM446344), and a seasonal H1N1 influenza virus, A/Thailand/CU-41/2006 (Accession No., EU021246.1) were used as the viral antigens in this study. These viruses had been isolated from previous outbreaks of influenza virus in Thailand [5, 14]. The viruses were propagated in 10-day-old embryonated eggs at the Center of Excellence in Clinical Virology, Faculty of Medicine, Chulalongkorn University, as previously described [15].
Six erythrocyte species derived from healthy turkeys (4), chicken (2), geese (3), pigs (3), horses (2), and humans (blood group O; 4) were used in this study. Fresh blood samples were combined with equal volumes of Alsever's solution and stored at 4℃ until used (within 72 hr). Because of reports of pH1N1 infection in human and swine populations, human and pig erythrocytes were tested for pre-existing antibodies against pH1N1 virus before performing the HA test. Pig and human erythrocytes, which were used in HA did not have pre-existing antibody against pH1N1 virus as determined by the HI assay. Because of lack of evidence for horse and avian pH1N1 infection, their sera were not tested for pH1N1 antibody.
The human specimens used were anonymous and provided by the Director of King Chulalongkorn Memorial Hospital and Chumphae Hospital, Khon Kaen. The human specimens were used under the Institutional Review Board (IRB) No. 431/52, which was approved by the Ethics Committee of the Faculty of Medicine, Chulalongkorn University.
The 96 serum samples used in this study were divided into 2 groups, as described below.
Twenty-one paired sera (acute and convalescent paired samples) were obtained from outpatients diagnosed with acute respiratory tract illness with ILI at Chumphae Hospital, Khon Kaen province, Thailand. The participants in the study consisted of 8 males and 13 females with average age of 14.86 years. Their nasal swabs were positive for pH1N1 when screened using real-time reverse transcription (RT)-PCR assays, as described in a previous study [16]. The acute sera were collected within the first 7 days after onset of symptoms, and the convalescent sera were collected at least 14 days after onset of the disease.
Fifty-four single sera were collected from healthy volunteers without respiratory symptoms, from Chumphae district, Khon Kaen province, Thailand to be screened for antibodies against pH1N1 virus in the general population of Thailand. The participants in this study consisted of 30 men and 24 women with an average age of 47.6 yr [17].
All samples were stored at -70℃ until tested.
The HA assay against pH1N1 was performed on 6 erythrocyte species; turkey, chicken, goose, human, horse, and pig. Identical aliquots of pH1N1 strains A/Thailand/CU-H88/2009 (H1N1), A/Thailand/CU-B1400/2009, and A/Thailand/CU-H1492/2010 (H1N1) and a seasonal H1N1 influenza virus A/Thailand/CU-41/2006 were used as virus antigens in the HA test. Erythrocyte species were used at a 0.5% (for chicken, goose, and turkey) and 1% (for pig, horse, and human) concentrations suspended in phosphate buffered saline (PBS; pH 7.4). Each strain of virus was titrated by HA test in all 6 erythrocyte species in 96-well microtiter plates with V-shaped wells (Greiner Bio-One GmbH, Kremsmuenster, Austria). The HA test procedure was developed on the basis of a previous publication by the WHO [6, 18].
The 8 hemagglutinating unit virus (HAU) detected by HA test with each erythrocyte species were 10-fold serially diluted. RNA was extracted from each virus dilution using the Viral Nucleic Acid Extraction Kit (RBC Bioscience Co, Taipei, Taiwan) following the manufacturer's instructions. RNA was used as template for viral titration by real-time RT-PCR with primers and probes as previously described; briefly, reactions were performed using the SuperScript III Platinum One-Step RT-PCR system (Invitrogen, Carlsbad, CA, USA) on a Rotor-Gene 3000 (Corbett Research, New South Wales, Australia) [5].
To determine whether erythrocyte binding preference of pH1N1 virus could alter the results of HI assays, sera were initially incubated with receptor-destroying enzyme (RDE), as described previously [19]. In the HI assay, pH1N1 A/Thailand/CU-H1492 (CU-H1492) was used as the viral antigen. Different erythrocyte species (turkey, chicken, goose, and human O type) were tested at identical concentrations (0.5% v/v). The HI assay was performed as previously described [6, 18].
The MN assay was performed following the Centers for Disease Control and Prevention (CDC) protocol [20, 21]. Serum samples were 2-fold serially diluted from 1:10 to 1:1,280 in 96-well microtiter plates using virus diluent (85.8% DMEM [GIBCO, Grand Island, NY, USA], 0.56% bovine serum albumin fraction V [Merck, Darmstadt, Germany], 25 mM HEPES buffer [Invitrogen], 100 mg/L streptomycin [Invitrogen], and 10,000 units/L penicillin [Invitrogen]). pH1N1 A/Thailand/CU-H1492 (CU-H1492) propagated in embryonated eggs was used in the MN assay. After serial dilution of sera, the 100 median tissue culture infective dose (TCID50) was added to the plate so that each well contained 100 TCID50/50 µL of virus, calculated using the Reed-Muench method [22]. The mixture of serum and virus was incubated for 60 min before 100 µL of 1.5×105 Madin-Darby Canine Kidney (MDCK) cells/mL in log phase growth, not exceeding the 25th passage, were added to the plate. The mixture was incubated for 18-22 hr at 37℃, 5% CO2. After incubation, the plates were washed with PBS followed by cell fixation in cold 80% acetone. ELISA against the nucleoprotein (NP) of influenza A was performed and endpoint titers were expressed as optical density (OD).
To compare HI titers to the titers obtained by the MN assay, percent accuracy and percent acceptability of the HI assay using different erythrocyte species were calculated. To calculate the percent accuracy, the number of HI titers that perfectly matched the MN titer were divided by total number of serum samples and then multiplied by 100. The number of HI titers matching the MN titer within±2-fold were used to calculate percent acceptability by using the same formula.
The geometric mean titers (GMTs) of HI assays obtained using different erythrocyte species and MN titers were calculated. The Statistical Package for the Social Sciences (SPSS) software version 17.0 (IBM, Somers, NY, USA) was used for statistical evaluation.
All participants were notified about their rights and the objectives of the study. Their written consent was obtained before specimen collection. The protocol of the immunological study was approved by the Ethics Committee of the Faculty of Medicine, Chulalongkorn University.
Four virus strains were employed in 3 different experiments, with 6 distinct species of red blood cells. In the HA test, the erythrocyte binding efficiency was demonstrated by the HA titers obtained for each human influenza virus with the 6 different erythrocyte species (Table 1). The results showed that the HA test conducted on goose erythrocytes gave the highest titers than those obtained using other erythrocyte species, while horse and pig erythrocytes did not agglutinate with both human seasonal or pandemic influenza virus. Human and turkey erythrocytes appeared to express comparable HA titers, although turkey erythrocytes are used as the standard erythrocytes according to WHO recommendation; HA test using chicken erythrocytes yielded lower HA titers than those using turkey erythrocytes (Table 1).
To confirm the quantity of virus in relation to different red blood cell binding specificity and to compare the viral titers obtained using the HA test, 8 HAU of seasonal and pH1N1 influenza A virus obtained using 4 erythrocyte species (turkey, chicken, goose, and human) were serially diluted and titrated by real-time RT-PCR. The cycle threshold (CT) value of real-time RT-PCR (Table 2) represents the amount of virus in each species. The 8 HAU of pH1N1 obtained using goose erythrocytes showed the highest CT values (19.7-33.0) using a 1 to 10-4 dilution for HA system, while the CT values obtained in turkey and human erythrocytes were comparable (18.6-31.3 and 18.1-31.3, respectively). Low CT values were obtained for the 8 HAU pH1N1 using chicken erythrocytes (16.8-30.8). As expected, 8 HAU of seasonal influenza virus had high CT values in all erythrocyte species (Table 2).
Additionally, to investigate whether the erythrocyte binding preference of pH1N1 influenza virus had any effect on the level of HI antibody titers, the HI assay was performed on 96 serum samples with pH1N1 strain A/Thailand/CU-H1492/2010 in 4 distinct erythrocyte species (turkey, chicken, goose, and human). The 8 HAU were titrated following WHO recommendations. The GMT for the MN assay was 91.52. The HI titers obtained with each erythrocyte species were compared. Furthermore, the percent accuracy (values exactly equal to MN titers) and percent acceptability (±2-fold of MN titers) were calculated after comparing with MN titers (Table 3).
This study proved that pre-existing antibody can interfere with titers observed in the HI assay. Turkey erythrocytes were used for testing the HI titer of serum obtained from human subjects whose erythrocytes were used in the HI assay and found that performing the assay on human blood group O erythrocytes negative for antibody against pH1N1 yielded HI titers most closely related to those obtained using turkey erythrocytes. Goose erythrocytes yielded the highest GMT (122.19), followed by that of turkey (71.91), human group O (62.64), and human blood group O with antibody (39.61). Using chicken erythrocytes in the HI assay provided the lowest GMT value (32.10). Comparison of HI results with those from MN assay indicated that turkey erythrocytes provided the most reliable results (% accuracy=56.25; % acceptability= 64.58) and human erythrocytes (without antibody against pH1N1) showed similar results (% accuracy=55.21; % acceptability= 62.50), followed by goose, human blood group O with antibody, and chicken erythrocytes (Table 3).
This study aimed to investigate the erythrocyte binding preferences of human pandemic influenza virus, H1N1 and their effects on antibody levels measured by HI titers of various erythrocyte species. Various studies have described different sialyloligosaccharide distributions; however, their exact biological role is unknown [19, 23-27]. This phenomenon can cause differences in the erythrocyte binding efficiency of influenza virus, depending on receptor specificity in the host species of origin [8, 12].
The HA molecules of the influenza virus interact in a different manner with specific types of SA molecules [13, 28]. Therefore, avian and equine influenza viruses prefer to interact with the different SA molecule in human [8, 10, 29]. According to a previous study, the classical swine influenza virus can bind to both galactose linkages, but the modified swine influenza virus is not able to bind to swine erythrocytes [30]. These previous findings correspond well with our results from the HA test and real-time RT-PCR. From the real-time RT-PCR results, high CT values for the same virus dilution (interpreted as low viral quantities) represent high binding efficiency, indicating the binding preference.
Therefore, the results showed that the pH1N1 virus had the highest efficiency in binding with goose erythrocyte but its efficiency was less than that of seasonal H1N1 virus. The binding efficiencies of human and turkey erythrocytes for pH1N1 virus were comparable, while the virus showed relatively low preference for chicken erythrocytes. These results indicated that pH1N1 virus can recognize and bind to cells containing SA-α-2,6-Gal. It appears that pH1N1 agglutinates goose erythrocytes more than the erythrocytes of other avian species (chicken and turkey). This observation suggests that there are some differences on the erythrocyte membranes of chicken, turkey, and goose, which could be the proportion of SA-α-2,6-Gal and SA-α-2,3-Gal molecules or that of the SAs, NeuGc and NeuAc. It may also indicate different distributions of other residues [13]. In addition, the results showed that neither pH1N1 nor seasonal H1N1 influenza virus were able to agglutinate equine erythrocytes. These results agree with those of a previous study showing that SA molecules on horse and cow cell membranes consist mainly of large amounts (93%) of glycolyl type, especially the α-2,3 linkage [31].
The results obtained using goose erythrocytes should be considered. Our results, similar to those of a study by Wiriyarat et al. [32], showed that goose erythrocytes yielded more sensitive HA titers than that using turkey erythrocytes, while a study by Ilyushina et al. showed a significantly lower HA titer using goose erythrocytes than that using turkey erythrocytes [30]. This phenomenon may be clarified by the fact that within the genus of goose, there are 3 genera of living true geese belonging to the subfamily Anserinae: Anser, referring to the gray geese or domestic geese; Chen, referring to white geese, although sometimes included in the genus Anser; and Branta which are black geese, such as the Canada goose [33]. The use of different genera of geese may affect the proportion of different types of SA molecules on the cell membrane, and thus result in different HA titers. Molecular sequencing of the SA receptor in these 3 genera of geese and comparison with the sequence of the pH1N1 influenza virus at the receptor binding site should be considered.
Our results of the HA test using chicken erythrocytes produced result similar to that by previous studies [30, 32]. However, in contrast to the results of our study, Wiriyarat et al. showed that pH1N1 virus could not agglutinate chicken erythrocytes [32]. Although the HA titers obtained using chicken erythrocytes were quite low, our results showed that the pH1N1 virus can agglutinate chicken erythrocytes, which was in concordance with the results of the study by Ilyushina et al. [30]. However, an alteration in the specificity for SA binding preference from SA-α-2,6-Gal to SA-α-2,3-Gal can occur because of a single amino acid change in the HA molecule [11]. This suggests that a mutation which potentially could have occurred during the emergence of this virus, may be correlated with the agglutination capacity of the virus. Potential mutations that might affect the binding affinity of HA molecules of pH1N1 species would require further investigation.
We also investigated whether different specificities in erythrocyte binding of pH1N1 resulted in variation in HI titers. The results showed that goose erythrocytes were the most sensitive in HI assays. However, although the HI assays performed with goose erythrocytes provided the highest HI titers among all species tested, the accuracy compared to MN assay results was less than that obtained using turkey erythrocytes. According to a previous study, there are more α-2,6-Gal than α-2,3-Gal on erythrocytes of human, turkey, and pig and more α-2,3-Gal on those of chicken and goose [12]. Therefore, there may be another general recognition mechanism for pH1N1 resulting in higher affinity of pH1N1 HA for the receptor molecule of goose erythrocytes; this requires further investigation. The HI titers obtained with human blood group O (without antibody against pH1N1) ranked second, most closely related to HI titers obtained with turkey erythrocytes followed by human erythrocytes with pH1N1 specific antibody, however these yielded the lowest percent accuracy, while the HI assay conducted on chicken erythrocytes produced the least sensitive result, with the lowest percent accuracy and also some incomplete inhibitions.
In addition, when comparing the HI titers obtained with human blood group O erythrocytes with and without specific antibody, the results showed an interference of specific antibodies with the HI assays. Therefore, substitution of human blood group O erythrocytes in HI assays in place of turkey erythrocytes would require prior testing of specific antibody. On the basis of a previous study, more than 40% of Thai people have already been infected with pH1N1 [17]. Moreover, it appears difficult to identify naïve humans as the pH1N1 has been continuously erupting for more than 1 year. In addition, perinatal transmission of pH1N1 has been reported [34]. pH1N1 displayed a host species-specific preference in its erythrocyte agglutination. Use of human blood group O (without antibody against pH1N1) will provide HI titers most closely related to those obtained with turkey erythrocytes. The various affinities in erythrocyte binding will affect the reliability of the results obtained using the HI assay. Appropriate selection of erythrocyte species for HI assay will allow construction of a more reliable database, which will be essential for further investigation and control of virus epidemics, such as herd immunity and vaccine policy.
Figures and Tables
Table 2
CT values of 8 hemagglutination (HA) unit serial dilutions for 4 erythrocyte species of human pandemic H1N1 and seasonal H1N1*, obtained using real-time reverse transcription (RT)-PCR assays
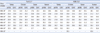
Table 3
GMTs, percent accuracy, and percent acceptability of HI assays obtained with each erythrocyte species, compared to MN assay titers
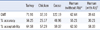
Human erythrocytes without antibody (*) and with Ab (†) were identified by testing for pre-existing antibody against the pH1N1 virus in human subjects whose erythrocytes were obtained for the HI assay.
Abbreviations: GMT, geometric mean titer; HI, hemagglutination inhibition; MN, microneutralization.
Acknowledgements
We would like to express our gratitude to Dr. Xiuhua Lu and Mr. David Wang (Immunology and Pathogenesis Branch, Influenza Division, CDC for training in serologic assays. We would like to thank the Commission on Higher Education, Ministry of Education, the Center of Excellence in Clinical Virology, Chulalongkorn University, CU Centenary Academic Development Project, King Chulalongkorn Memorial Hospital, Graduate School of Biomedical Science, Chulalongkorn University, and the Chula DusadeePipat Fund. This work was supported by the Higher Education Research Promotion and National Research University Project of Thailand (HR1155A). Thailand Research Fund (DPG5480002) and in part with federal funds from the NIAID, NIH, Department of Health and Human Services, under Contract no. HHSN266200700007c. We would also like to thank MK Restaurant Company Limited for their generous support, Dr. Praveena Kittikhun and Ms. Aunyaratana Thontiravong for laboratory assistance, the staff and nurses of Chumphae Hospital, Khon Kaen province, Thailand for collecting the clinical data and specimens, and Ms. Petra Hirsch and Mr. Patrick Beuhler for assistance in editing the manuscript.
References
1. Centers for Disease Control and Prevention. Swine influenza A (H1N1) infection in two children-Southern California, March-April 2009. MMWR Morb Mortal Wkly Rep. 2009. 58:400–402.
2. World Health Organization. Clinical management of adult patients with complications of pandemic influenza A (H1N1) 2009: emergency guidelines for the management of patients with severe respiratory distress and shock in district hospitals in limited-resource settings. 2010. Geneva, Switzerland: World Health Organization.
3. Pandemic (H1N1) 2009 - update 111. World Health Organization. Updated on Jul 2010. http://www.who.int/csr/don/2010_07_30/en/.
4. Boonsuk P, Payungporn S, Chieochansin T, Samransamruajkit R, Amonsin A, Songserm T, et al. Detection of influenza virus types A and B and type A subtypes (H1, H3, and H5) by multiplex polymerase chain reaction. Tohoku J Exp Med. 2008. 215:247–255.


5. Chieochansin T, Makkoch J, Suwannakarn K, Payungporn S, Poovorawan Y. Novel H1N1 2009 influenza virus infection in Bangkok, Thailand: effects of school closures. Asian Biomed. 2009. 3:469–475.
6. World Health Organization. WHO manual on animal influenza diagnosis and surveillance. Department of communicable disease, World Health Organization. 2002. Geneva, Switzerland: World Health Organization.
7. Klenk E, Uhlenbruck G. A neuraminic acid containing mucoprotein from cattle erythrocyte stroma. Hoppe Seyler Z Physiol Chem. 1958. 311:227–233.
8. Rogers GN, Paulson JC. Receptor determinants of human and animal influenza virus isolates: differences in receptor specificity of the H3 hemagglutinin based on species of origin. Virology. 1983. 127:361–373.


9. Yamakawa T, Nagai Y. Glycolipid at the cell surface and their biological functions. Trends Biochem Sci. 1978. 3:128–131.
10. Rogers GN, Paulson JC, Daniels RS, Skehel JJ, Wilson IA, Wiley DC. Single amino acid substitutions in influenza haemagglutinin change receptor binding specificity. Nature. 1983. 304:76–78.


11. Stephenson I, Wood JM, Nicholson KG, Zambon MC. Sialic acid receptor specificity on erythrocytes affects detection of antibody to avian influenza haemagglutinin. J Med Virol. 2003. 70:391–398.


12. Ito T, Suzuki Y, Mitnaul L, Vines A, Kida H, Kawaoka Y. Receptor specificity of influenza A viruses correlates with the agglutination of erythrocytes from different animal species. Virology. 1997. 227:493–499.


13. Suzuki Y, Ito T, Suzuki T, Holland RE Jr, Chambers TM, Kiso M, et al. Sialic acid species as a determinant of the host range of influenza A viruses. J Virol. 2000. 74:11825–11831.


14. Chutinimitkul S, Chieochansin T, Payungporn S, Samransamruajkit R, Hiranras T, Theamboonlert A, et al. Molecular characterization and phylogenetic analysis of H1N1 and H3N2 human influenza A viruses among infants and children in Thailand. Virus Res. 2008. 132:122–131.


15. Makkoch J, Payungporn S, Prachayangprecha S, Tantilertcharoen R, Poovorawan Y. Determination of antibody response to the human pandemic influenza H1N1 2009 among patients with influenza-like illness and high risk group. Asian Pac J Allergy Immunol. 2010. 28:67–75.
16. CDC protocol of realtime RT-PCR for influenza A (H1N1). World Health Organization. Updated on Apr 2009. http://www.who.int/csr/resources/publications/swineflu/realtimeptpcr/en/index.html.
17. Prachayangprecha S, Makkoch J, Payungporn S, Chieochansin T, Vuthitanachot C, Vuthitanachot V, et al. Serological analysis of human pandemic influenza (H1N1) in Thailand. J Health Popul Nutr. 2010. 28:537–544.


18. Kendal AP, Pereira MS, Skehel JJ. Concepts and procedures for laboratory-based influenza surveillance. 1982. Atlanta, GA: U.S. Department of Health and Human Services, Public Health Service, Centers for Disease Control.
19. Martensson E, Raal A, Svennerholm L. Sialic acid in blood serum. Biochim Biophys Acta. 1958. 30:124–129.
20. Hancock K, Veguilla V, Lu X, Zhong W, Butler EN, Sun H, et al. Cross-reactive antibody response to the 2009 pandemic H1N1 influenza virus. N Engl J Med. 2009. 361:1945–1952.
21. Rowe T, Abernathy RA, Hu-Primmer J, Thompson WW, Lu X, Lim W, et al. Detection of antibody to avian influenza A (H5N1) virus in human serum by using a combination of serologic assays. J Clin Microbiol. 1999. 37:937–943.


22. Reed LJ, Muench H. A simple method of estimating fifty percent endpoint. Am J Hyg. 1938. 27:493–497.
23. Hara S, Takemori Y, Yamaguchi M, Nakamura M, Ohkura Y. Fluorometric high-performance liquid chromatography of N-acetyl-and N-glycolylneuraminic acids and its application to their microdetermination in human and animal sera, glycoproteins, and glycolipids. Anal Biochem. 1987. 164:138–145.
24. Naiki M. Chemical and immunochemical properties of two classes of globoside from equine organs. Jpn J Exp Med. 1971. 41:67–81.
25. Pettersson S, Sivertsson OR, Sjogren S, Svennerholm L. The sialic acids of hog pancreas. Biochim Biophys Acta. 1958. 28:444–445.
26. Schauer R, editor. Sialic acids, chemistry, metabolism, and function. 1982. New York: Springer-Verlag.
28. Higa HH, Rogers GN, Paulson JC. Influenza virus hemagglutinins differentiate between receptor determinants bearing N-acetyl-, N-glycollyl- and N,O-diacetylneuraminic acids. Virology. 1985. 144:279–282.
29. Connor RJ, Kawaoka Y, Webster RG, Paulson JC. Receptor specificity in human, avian, and equine H2 and H3 influenza virus isolates. Virology. 1994. 205:17–23.


30. Ilyulshina NA, Kim JK, Negovetich NJ, Choi YK, Lang V, Bovin NV, et al. Extensive mammalian ancestry of pandemic (H1N1) 2009 virus. Emerg Infect Dis. 2010. 16:314–317.
31. Suzuki Y, Matsunaga M, Matsumoto M. N-Acetylneuraminyllactosylceramide, GM3-NeuAc, a new influenza A virus receptor which mediates the adsorption-fusion process of viral infection. Binding specificity of influenza virus A/A/ichi/2/68 (H3N2) to membrane-associated GM3 with different molecular species of sialic acid. J Biol Chem. 1985. 260:1362–1365.


32. Wiriyarat W, Lerdsamran H, Pooruk P, Webster RG, Louisirirotchanakul S, Ratanakorn P, et al. Erythrocyte binding preference of 16 subtypes of low pathogenic avian influenza and 2009 pandemic influenza A (H1N1) viruses. Vet Microbiol. 2010. 146:346–349.


33. Carboneras C. del Hoyo J, Elliot A, Sargatal J, editors. Family Anatidae (ducks, geese, and swans). Handbook of the birds of the world. 1992. Barcelona: Lynx Edicions;536–628.