Abstract
Background
Osteoclasts are bone resorbing cells and are responsible for bone erosion in diseases as diverse as osteoporosis, periodontitis, and rheumatoid arthritis. Fexaramine has been developed as an agonist for the farnesoid X receptor (FXR). This study investigated the effects of fexaramine on receptor activator of nuclear factor (NF)-κB ligand (RANKL)-induced osteoclast formation and signaling pathways.
Methods
Osteoclasts were formed by culturing mouse bone marrow-derived macrophages (BMMs) with macrophage colony-stimulating factor (M-CSF) and RANKL. Bone resorption assays were performed using dentine slices. The mRNA expression level was analyzed by real-time polymerase chain reaction. Western blotting assays were conducted to detect the expression or activation level of proteins. Lipopolysaccharide-induced osteoclast formation was performed using a mouse calvarial model.
Results
Fexaramine inhibited RANKL-induced osteoclast formation, without cytotoxicity. Furthermore, fexaramine diminished the RANKL-stimulated bone resorption. Mechanistically, fexaramine blocked the RANKL-triggered p38, extracellular signal-regulated kinase, and glycogen synthase kinase 3β phosphorylation, resulting in suppressed expression of c-Fos and NF of activated T cells (NFATc1). Consistent with the in vitro anti-osteoclastogenic effect, fexaramine suppressed lipopolysaccharide-induced osteoclast formation in the calvarial model.
Bone homeostasis is maintained via continual resorption of bone by osteoclasts and its replacement by osteoblasts. The disruption of bone homeostasis leads to pathogenic conditions such as osteoporosis, rheumatoid arthritis, lytic bone metastases, or Paget's bone disease.[12] Osteoclasts are multinucleated cells that are derived from the monocyte-macrophage lineage. Excessive osteoclastic bone resorption plays a critical role in bone destruction in pathological bone diseases such as osteoporosis, rheumatoid arthritis, periodontal disease, and some metastatic cancers.[2] Thus, it is clinically important to develop drugs that can modulate osteoclast differentiation.
The receptor activator of nuclear factor (NF)-κB ligand (RANKL) is essential for osteoclastic differentiation in the presence of macrophage colony-stimulating factor (M-CSF). [34] For osteoclast differentiation and activation, the interaction between receptor activator of NF-κB (RANK) and RANKL is essential.[456] The binding of RANKL and RANK on osteoclast progenitor cells triggers the activation of downstream signaling pathways including mitogen-activated protein kinase (MAPKs) and glycogen synthase kinase 3β (GSK3β).[78] c-Fos and NF of activated T cells (NFATc1) are downstream transcription factors in the RANKL/RANK signal pathway and play a crucial role in osteoclastogenesis. Particularly, NFATc1, a key molecule in osteoclastogenesis, induces a series of osteoclast-specific genes including cathepsin K, tartrate-resistant acid phosphatase (TRAP), dendritic cell-specific transmembrane protein (DC-STAMP), and αvβ3 integrins.[9101112]
Fexaramine is a synthetic, selective and potent farnesoid X receptor (FXR) agonist.[13] FXR is a member of the nuclear hormone receptor family and functions as a bile acid sensor. FXR plays important roles in the regulation of bile acid and cholesterol homeostasis, glucose metabolism, and insulin sensitivity.[14] A recent, new finding suggests that FXR has much broader roles than previously thought [15] including diverse pharmacologic activities. Therefore, FXR modulators have been extensively studied as a new therapeutic target for numerous metabolic disorders.
We have previously shown that deletion of FXR (FXR−/−) in vivo resulted in a significant reduction in bone mineral density compared with FXR+/+ mice.[16] Furthermore, FXR agonist enhances osteoblast differentiation and suppresses osteoclast differentiation, indicating that therapeutic modulation of FXR could be beneficial in patients with osteoporosis.[16] However, the mechanisms underlying the effect of FXR agonist in osteoclast differentiation are poorly understood. Thus, we investigated the role of fexaramine on RANKL-induced osteoclastogenesis, using mouse primary osteoclast precursors. Our results suggested that fexaramine has therapeutic potential for diseases associated with bone loss.
Fexaramine was purchased from Sigma-Aldrich Corporation (St. Louis, MO, USA). Antibodies against extracellular signal-regulated kinase (ERK), phospho-ERK, p38, phospho-p38, phospho-GSK and c-Fos were purchased from Cell Signaling Technology (Danvers, MA, USA). Antibodies against β-actin were purchased from Abcam (Cambridge, MA, USA). Antibodies against NFATc1 and rabbit were purchased from Santa Cruz (Santa Cruz, CA, USA). All other reagents were from Sigma-Aldrich.
Bone marrow cells were obtained from the long bones of 8- to 10-week-old C57BL/6J mice (Samtako Inc., Osan, Korea). Bone marrow cells were cultured in the presence of M-CSF (30 ng/mL; PeproTech Inc., Rocky Hill, NJ, USA) for three days to generate the bone marrow-derived macrophages (BMMs). To examine osteoclast formation, BMMs were treated with fexaramine in the presence of M-CSF (30 ng/mL) and RANKL (100 ng/mL; PeproTech Inc., Rocky Hill, NJ, USA) in 96-well culture plates (Corning, MA, USA). After four days, the cells were fixed and stained for TRAP. All experiments were performed in accordance with the institutional guidelines approved by the Sookmyung Women's University Animal Care and Use Committee.
Cell cytotoxicity was determined by the microtitration (MTT) assay. BMMs (1×104 cells/well) were placed in a 96-well plate and cultured with M-CSF (30 ng/mL, R&D) and fexaramine (5 µM) in α-minimum essential medium (MEM) for 48 hr. The MTT solution was added and incubated in the dark. After 5 hr, solubilization buffer (10% sodium dodecyl sulfate [SDS] in 0.01 M hydrochloric acid [HCl]) was added and the cells were incubated overnight. The proliferation of the BMMs was determined by measuring the optical density (OD) of the wells at 570 nm.
Total RNA was purified with Easy-Blue (iNtRON Biotechnology Inc., Seongnam, Korea). The cDNA was synthesized from 5 µg of RNA by using the Revert Aid™ first-strand cDNA synthesis kit (iNtRON Biotechnology Inc.) and amplified using real-time PCR. The following primers of osteoclastogenic genes were used in this study: Cathepsin K (F) 5'-ACGGAGGCATTGACTCTGAAGATA-3', (R) 5'-GTTGTTCTTATTCCGAGCCAAGAG-3'; DC-STAMP (F) 5'-TGGAAGTTCACTTGAAACTACGTG-3', (R) 5'-CTCGGTTTCCCTCAGCCTCTCTC-3'; αv-Integrin (F) 5'-CCTCAAGAGGGAGATGTTCACAC-3', (R) 5'-AACTGCCAAGATGATCACCCACAC-3'; β3-Integrin (F) 5'-GATGACATCGAGCAGGTGAAAGAG-3', (R) 5'-CCGGTCATGAATGGTGATGAGTAG-3'; glyceraldehyde 3-phosphate dehydrogenase (GAPDH) (F) 5'-TGCACCACCAACTGCTTAGC-3', (R) 5'-GGCATGGACTGTGGTCATGAG -3'. Real-time PCR reactions were performed in a total volume of 20 µL using SYBR® Green PCR Master Mix (Applied Biosystems, Foster City, CA, USA) according to the manufacturer's instructions. Thermocycling was performed using a 7500 real-time PCR System (Applied Biosystems) with the following conditions: initial hold, 95℃ for 10 min; followed by 40 cycles of denaturation at 95℃ for 15 sec, annealing at 58℃, and extension at 60℃ for 1 min. An index mRNA level was assessed using a threshold cycle value and normalized against GAPDH expression.
Total cell lysates were separated by SDS-polyacrylamide gel electrophoresis (PAGE) and transferred onto Immobilon-P membranes (Millipore, Bedford, MA, USA). The membranes were blocked with 5% non-fat-milk in phosphate buffered saline (PBS)-Tween (PBS-T), immunostained with anti-phospho ERK (1:1,000), anti-phospho p38 (1:1,000), anti-ERK (1:1,000), anti-p38 (1:1,000), anti-phospho GSK (1:1,000), anti-NFATc1 (1:200), anti-c-Fos (1:1,000), and anti-β-actin (1:4,000), and incubated with a horseradish peroxidase-conjugated secondary antibody (1:5,000). The membranes were developed using an advanced chemiluminescence detection kit (Amersham Biosciences, Buckinghamshire, UK).
BMMs were differentiated on dentine slices with M-CSF (30 ng/mL) and RANKL (100 ng/mL) for four days, and then treated with fexaramine for two days. The surfaces of the dentine slices were wiped to remove the cells, and the slices were then stained with toluidine blue (1 µg/mL; J.T. Baker Chemical Co., Phillipsburg, NJ, USA). The number of pits formed by bone resorption on the dentine slices was counted.
Plat-E retroviral packaging cells were seeded in culture dish before 1 day transfection. Next day, PMX-puro-GFP and PMSCV-GFP-CA-NFATc1 were transfected into plat-E cells using Lipofectamine 2000 CD (Invitrogen, Carlsbad, CA, USA). After 2 days culture, culture supernatants were collected. BMMs were plated with supernatants which containing PMX-puro-GFP and PMSCV-GFP-CA-NFATc1 virus producing by plat-E cells in the presence of polybrene (10 µg/mL) and M-CSF (30 ng/mL) overnight in 48-well plate. Infected cells were then selected with puromycine (2 µg/mL) for 2 days and then further cultured with or without fexaramine (5 µM) in the presence of M-CSF (30 ng/mL) and RANKL (100 ng/mL) for 4 days.
C57BL/6J mice (8-weeks-old) were i.p. injected with vehicle (dimethylsulfoxide [DMSO]; corn oil, 1:9) or fexaramine (5 mg/kg) every day. The day after the first injection of fexaramine, lipopolysaccharide (LPS; 0.5 mg) or PBS was directly injected on the calvarium. Six days after the LPS injection, mice were sacrificed and the calvaria were extracted. Calvaria were fixed in 4% paraformaldehyde for 24 hr at 4℃, and then stained for TRAP. Image analysis was accomplished using ImageJ software (version 1.32; National Institute of Health, Bethesda, MD, USA) according to the manufacturer's protocol.
We investigated the effects of fexaramine on RANKL-induced osteoclast differentiation, using mouse BMMs. BMMs were cultured with RANKL and M-CSF in the presence or absence of fexaramine for 4 days. BMMs differentiated into mature TRAP-positive (TRAP+) multinucleated osteoclasts (MNCs) during the culture period, while treatment with fexaramine inhibited osteoclast differentiation in a dose-dependent manner (Fig. 1A). The MTT assay showed that the anti-osteoclastogenic effect of fexaramine was not attributable to cellular toxicity (Fig. 1B). We further assessed the inhibitory effect of fexaramine on osteoclastogenesis, by evaluating the RANKL-induced mRNA expression levels of osteoclast-related genes, such as cathepsin K, DC-STAMP, and Integrin αV/β3. Real-time-PCR analysis revealed the expression levels of those genes were dramatically increased in BMMs challenged by RANKL and M-CSF for 4 days. However, the treatment with fexaramine significantly inhibited RANKL-induced osteoclast-related gene expression (Fig. 1C).
We next examined at which stage fexaramine impaired osteoclast development. Fexaramine was added to osteoclast-generating cultures on different days (D0-4), and TRAP staining was performed on day 4. Fexaramine effectively inhibited osteoclast formation when added on the first 2 days (D0-2) and the last 2 days (D2-4) of culture, suggesting that it affects both early and late osteoclastogenesis (Fig. 2A).
As mentioned above, fexaramine is known as a selective FXR agonist.[13] Therefore, we examined if the inhibitory effect of fexaramine on RANKL-induced osteoclast formation is dependent on FXR, using FXR-deficient cells. Figure 2B shows the deficiency of FXR did not affect the anti-osteoclastogenic effect of fexaramine. These data suggest that the effect of fexaramine on RANKL-induced osteoclast formation is FXR-independent.
Then, to examine whether the effect of fexaramine on osteoclast formation could be reflected in osteoclastic activity, we performed an in vitro resorption pit assay, using a dentine slice. Many resorption pits were generated with RANKL-treated cells (Fig. 2C). In contrast, fexaramine treatment strongly inhibited the formation of resorption pits by the RANKL-treated cells. Therefore, these results suggested that fexaramine exerted inhibitory effects on osteoclast formation that led to reduced bone resorption.
Next, we examined the molecular mechanism of the anti-osteoclastogenic effect of fexaramine, using BMMs. We first investigated the effects of fexaramine on NFATc1 and c-Fos expression levels. RANKL stimulation increased the expression of c-Fos and NFATc1 in BMMs. Fexaramine abolished the RANKL-induced protein expression of c-Fos and NFATc1 (Fig. 3A, B). We next investigated whether the overexpression of NFATc1 restored the effect of fexaramine. BMMs were transduced with the constitutively active form of NFATc1 (CA-NFATc1) and were cultured with M-CSF and RANKL in the absence or presence of fexaramine for 4 days. As shown in Figure 3C, suppression of osteoclastogenesis by fexaramine was efficiently overcome by the forced expression of CA-NFATc1, suggesting that the anti-osteoclastogenic effect of fexaramine is due mainly to the reduction in NFATc1 expression.
When we examined the effects of fexaramine on the early signaling pathways induced by RANKL in BMMs, we found that fexaramine pretreatment suppressed the phosphorylation of p38 and ERK by RANKL (Fig. 4A, B). We also examined effects on the GSK3β signaling pathway. Upon RANKL stimulation, GSK-3β is phosphorylated at Ser-9, causing its inactivation. GSK3β inactivation is a prerequisite for the NFATc1 amplification process, thereby, resulting in upregulation of osteoclast formation.[8] RANKL stimulation led to the phosphorylation of GSK3β at Ser-9, and fexaramine interfered with this process (Fig. 3C). Altogether, the results suggested that fexaramine downregulated RANKL-induced expression of NFATc1 and c-Fos via the p38, ERK, and GSK3β signaling pathways.
We finally evaluated the in vivo effect of fexaramine on osteoclast formation, using a LPS-challenged mouse model. LPS has been reported to stimulate bone loss, by increasing the number of osteoclasts in vivo.[17] When we injected LPS into the supracalvarial region of mice, with or without fexaramine, TRAP staining of whole calvariae showed that LPS dramatically increased osteoclast numbers (Fig. 5A). In parallel with the effects in vitro, fexaramine notably reduced LPS-induced osteoclast formation (Fig. 5B). Collectively, we conclude that fexaramine has an inhibitory effect on inflammation-induced osteoclastogenesis in vivo.
In this study, we have shown that fexaramine effectively inhibits RANKL-induced osteoclast differentiation, without cytotoxicity. The suppressive effect of fexaramine on pit formation on dentine slices also suggested that fexaramine inhibits the bone resorptive function of osteoclasts. Although fexaramine has been developed as a selective agonist for FXR, a nuclear orphan receptor, our results suggested that the anti-osteoclastic effect of fexaramine occurs regardless of the presence of FXR.
RANKL-RANK signaling leads to the induction of NFATc1, which is an essential transcription factor for osteoclast differentiation.[1112] For this pathway, activation of the MAPK and GSK3β pathways is a prerequisite for osteoclast differentiation.[78] The addition of fexaramine to osteoclast precursors attenuated the RANKL-induced phosphorylation of p38 and ERK MAPK, as well as of GSK3β. Furthermore, fexaramine also inhibited RANKL-induced NFATc1. NFATc1 is a key transcription factor for the expression of TRAP and other osteoclastogenesis-associated genes. Our mechanistic study suggests that the inhibitory mechanism of fexaramine appears to be related to this pathway. Also, fexaramine significantly inhibited the LPS-induced osteoclast formation in calvarial bone in vivo.
Previous study have shown that FXR agonists significantly enhanced osteoblastic differentiation through the upregulation of Runx2 and enhanced ERK and β-catenin signaling.[16] Furthermore the therapeutic use of FXR agonists in association with bisphosphonates has been suggested as a new additional strategy to limit the deleterious consequences of bone loss.[18] Given that anti-osteoclastogenic effect of fexaramine in our study, fexaramine may regulate both arms of bone remodeling; ie, bone formation and resorption. Since fexaramine has FXR-independent effect on osteoclast formation, however, development of new FXR agonist with higher specificity would be needed to decrease the undesired adverse effects in the future.
Bone loss is a growing international health care issue. Bone loss is the result of a negative unbalance between bone formation ad bone resorption. Continuous efforts have been done to find out the new target molecule to treat bone loss.[1920] Since RANKL/RANK mechanism is involved in the pathological processes inducing bone loss, antiresorptive agents, such as bisphosphonates and denosumab (anti-RANKL antibody), has been considered as the promising treatment strategies to treat bone loss.[21] Despite these remarkable advances, concerns about side-effects of anti-resorptive drugs are leading many patients, who could benefit from drug therapy, to not take these medications. As such, there remains an important clinical need to develop new drugs that do not cause these side-effects. In this study, we demonstrated that fexaramine, a FXR agonist, inhibits osteoclastogenesis in vitro and in vivo. Consequently, continued advanced studies on the structural optimization of FXR agonist with higher specificity will provide a basis for the development of new therapeutic agents.
Figures and Tables
Fig. 1
Fexaramine (Fexa) inhibits receptor activator of nuclear factor-κB ligand (RANKL)-induced osteoclast formation. (A) Bone marrow-derived macrophages (BMMs) were cultured with RANKL (100 ng/mL) and macrophage colony-stimulating factor (M-CSF) (30 ng/mL) in the presence of the indicated concentration of fexa for 4 days and tartrate-resistant acid phosphatase-positive (TRAP+) multinucleated osteoclasts (MNCs) were counted. (B) BMMs were cultured with M-CSF (30 ng/mL) in the presence or absence of fexa (5 µM) for 2 days and an microtitration assay was performed. (C) BMMs were cultured with M-CSF (30 ng/mL) in the presence or absence of fexa (5 µM) for 4 days and messenger ribonucleic acids expression level was determined by real time-polymerase chain reaction. Data are expressed as mean±standard deviation from at least three independent experiments. *P<0.05. Veh, vehicle; GAPDH, glyceraldehyde 3-phosphate dehydrogenase; DC-STAMP, dendritic cell-specific transmembrane protein.
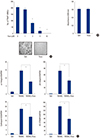
Fig. 2
Fexaramine (Fexa) inhibits bone resorption. (A) Fexa (5 µM) was added during the indicated culture days in the presence of macrophage colony-stimulating factor (M-CSF) (30 ng/mL) and receptor activator of nuclear factor-κB ligand (RANKL) (100 ng/mL). (B) Bone marrow-derived macrophages (BMMs) from farnesoid X receptor (FXR)+/+ and FXR−/−mice were cultured with M-CSF (30 ng/mL) and RANKL (100 ng/mL) in the presence of indicated concentrations of fexa for 4 days, and tartrate-resistant acid phosphatase-positive (TRAP+) osteoclasts were counted. (C) BMMs were differentiated on dentine slices with M-CSF (30 ng/mL) and RANKL (100 ng/mL) for four days and fexa (5 µM) was treated for an additional two days. The number of resorption pits were counted. Scale bar=200 µm. Data are expressed as mean±standard deviation from at least three independent experiments. *P<0.05. MNCs, multinucleated osteoclasts; WT, wild-type; Veh, vehicle.
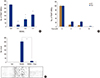
Fig. 3
Fexaramine (Fexa) inhibits receptor activator of nuclear factor-κB ligand (RANKL)-induced expression of c-Fos and nuclear factor of activated T cells (NFATc1). (A, B) Bone marrow-derived macrophages (BMMs) were preincubated in the absence or presence of fexa (5 µM) for 30 min, and then treated with or without 200 ng/mL of RANKL for 24 hr. Cell lysates were then subjected to Western blot analysis using (A) NFATc1 or (B) c-Fos antibodies. (C) BMMs were infected through the retrovirus packaging system. Infected BMMs were cultured with RANKL (100 ng/mL) and macrophage-colony stimulating factor (30 ng/mL) in the absence or presence of fexa (5 µM) for 4 days. The recovery rate was defined as the percentage of osteoclast formation in the presence of fexa. The osteoclast formation in the presence of vehicle was given as 100%. Data are expressed as mean±standard deviation from at least three independent experiments. *P<0.05. Veh, vehicle.
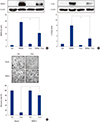
Fig. 4
Fexaramine (Fexa) inhibits receptor activator of nuclear factor-κB ligand (RANKL)-induced signaling pathways. (A-C) Bone marrow-derived macrophages (BMMs) were preincubated in the absence or presence of fexa (5 µM) for 30 min, and then treated with or without 200 ng/mL RANKL for (A, B) 15 min or (C) 24 hr. Cell lysates were then subjected to Western blotting analysis with the indicated antibodies. Data are expressed as the mean±standard deviation from at least three independent experiments. *P<0.05. Veh, vehicle; p-p38, phospho-p38; ERK, extracellular signal-regulated kinase; p-ERK, phosphor-extracellular signal-regulated kinase; p-GSK, phosphor-glycogen synthase kinase.
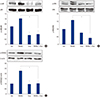
Fig. 5
Fexaramine (Fexa) suppresses lipopolysaccharide (LPS)-induced osteoclast formation in vivo. (A) Calvaria of mice that received vehicle, LPS, or LPS plus fexa (5 mg/kg) were subjected to tartrate-resistant acid phosphatase (TRAP) staining. (B) TRAP+stained area in calvaria were quantified using the image J program. Representative images are shown. Data are expressed as the mean±standard deviation from at least three independent experiments. *P<0.05. Veh, vehicle.
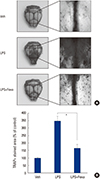
ACKNOWLEDGEMENT
This study was supported by research funding from Korean Society for Bone and Mineral Research.
References
3. Suda T, Takahashi N, Udagawa N, et al. Modulation of osteoclast differentiation and function by the new members of the tumor necrosis factor receptor and ligand families. Endocr Rev. 1999; 20:345–357.


4. Boyle WJ, Simonet WS, Lacey DL. Osteoclast differentiation and activation. Nature. 2003; 423:337–342.


5. Wong BR, Rho J, Arron J, et al. TRANCE is a novel ligand of the tumor necrosis factor receptor family that activates c-Jun N-terminal kinase in T cells. J Biol Chem. 1997; 272:25190–25194.


6. Theill LE, Boyle WJ, Penninger JM. RANK-L and RANK: T cells, bone loss, and mammalian evolution. Annu Rev Immunol. 2002; 20:795–823.


7. Lee ZH, Kim HH. Signal transduction by receptor activator of nuclear factor kappa B in osteoclasts. Biochem Biophys Res Commun. 2003; 305:211–214.


8. Jang HD, Shin JH, Park DR, et al. Inactivation of glycogen synthase kinase-3beta is required for osteoclast differentiation. J Biol Chem. 2011; 286:39043–39050.


9. Matsuo K, Galson DL, Zhao C, et al. Nuclear factor of activated T-cells (NFAT) rescues osteoclastogenesis in precursors lacking c-Fos. J Biol Chem. 2004; 279:26475–26480.


10. Wagner EF, Eferl R. Fos/AP-1 proteins in bone and the immune system. Immunol Rev. 2005; 208:126–140.


11. Asagiri M, Sato K, Usami T, et al. Autoamplification of NFATc1 expression determines its essential role in bone homeostasis. J Exp Med. 2005; 202:1261–1269.


12. Takayanagi H, Kim S, Koga T, et al. Induction and activation of the transcription factor NFATc1 (NFAT2) integrate RANKL signaling in terminal differentiation of osteoclasts. Dev Cell. 2002; 3:889–901.


13. Downes M, Verdecia MA, Roecker AJ, et al. A chemical, genetic, and structural analysis of the nuclear bile acid receptor FXR. Mol Cell. 2003; 11:1079–1092.


14. Wang YD, Chen WD, Moore DD, et al. FXR: a metabolic regulator and cell protector. Cell Res. 2008; 18:1087–1095.


16. Cho SW, An JH, Park H, et al. Positive regulation of osteogenesis by bile acid through FXR. J Bone Miner Res. 2013; 28:2109–2121.


17. Ha H, Lee JH, Kim HN, et al. Alpha-Lipoic acid inhibits inflammatory bone resorption by suppressing prostaglandin E2 synthesis. J Immunol. 2006; 176:111–117.


18. Id Boufker H, Lagneaux L, Fayyad-Kazan H, et al. Role of farnesoid X receptor (FXR) in the process of differentiation of bone marrow stromal cells into osteoblasts. Bone. 2011; 49:1219–1231.


19. Kim HJ, Ohk B, Kang WY, et al. Deficiency of lipocalin-2 promotes proliferation and differentiation of osteoclast precursors via regulation of c-fms expression and nuclear factor-kappa B activation. J Bone Metab. 2016; 23:8–15.

